Chapter 61 Epilepsy Surgery in the Pediatric Population
Epilepsy is one of the most common chronic disorders facing children and adolescents. The overall prevalence of epilepsy has been estimated to be 5–8 per 1000 [Hauser et al., 1975, Hauser, 1994, 1996, 1998; Olafsson et al., 1996; Osuntokun et al., 1987]. Extrapolating the Hauser data from Rochester, Minnesota, of 6.66 per 1000 to the total population in 2004, approximately 2.3 million persons in the United States have epilepsy [Hauser et al., 1991]. In children, there are approximately 10.5 million worldwide with epilepsy. The annual incidence of epilepsy in children is reported to be 61–124 per 100,000 in developing countries and 41–50 per 100,000 in developed countries [Guerrini, 2006]. The cumulative risk of developing epilepsy from birth through adolescence is 1 percent [Hauser et al., 1991, 1994]. Unfortunately, only 60–70 percent of patients will achieve seizure freedom with antiepileptic medications [Kwan and Brodie, 2000; Mohanraj and Brodie, 2006]. The introduction of several new antiepileptic drugs over the past 15 years has not changed the fact that approximately 30–40 percent of patients with epilepsy will be medically refractory [Perucca et al., 2007; Mohanraj and Brodie, 2006].
In addition, the longer epilepsy persists without control, the less likely is the chance of remission. Specifically, if seizures remain inadequately controlled for longer than 4 years, the chance of remission decreases to approximately 10 percent [Annegers et al., 1979]. Seizure duration of over 10 years also decreases the likelihood of achieving control in patients who undergo surgery. The presence of multiple seizure types and frequent generalized tonic-clonic seizures also lessens the chance for complete remission. As stated above, 30–40 percent [Mohanraj and Brodie, 2006] of all persons with epilepsy will be intractable. There are approximately 20,000 cases of new-onset epilepsy annually. Therefore, 6000–8000 cases of new-onset epilepsy are intractable each year. In the United States, the number of patients with drug-resistant epilepsy – adult and pediatric – is estimated to be 700,000, a higher number than the number of individuals affected with Parkinson’s disease and multiple sclerosis combined [Hiritiz et al., 2007]. Approximately 60 percent of these patients will have partial seizures. Estimates by several investigators suggest that many of these patients are epilepsy surgery candidates [Hauser, 1993; Unnwongse et al., 2010]. This figure may increase as new technologies enable more precise identification of an underlying epileptogenic focus. Additionally, as medical intractability for children with epilepsy is further defined, the number of pediatric epilepsy surgical procedures will probably increase. However, currently, epilepsy surgery is underutilized in the treatment of intractable epilepsy. In fact, over the past 15 years, the mean duration of epilepsy before referral to a tertiary care epilepsy center for evaluation for epilepsy surgery has been over 20 years [Engel et al., 2003; Unnwongse et al., 2010; Choi et al., 2009].
The developing brain is highly susceptible to recurrent seizures. Until recently, the brain was believed to be relatively resistant to injury. A growing body of evidence in animal models, however, suggests that early seizures, even if brief and recurrent, can result in demonstrable structural and physiologic changes in the developing brain’s circuitry, resulting in aberrant excitation and inhibition. Clinically, these defects produces spontaneous seizures (epilepsy) and cognitive impairments [Holmes and Ben-Ari, 1998; Holmes et al., 1998; Stafstrom et al., 2000; Galanopoulou and Moshé, 2009], with the possibility of missed windows of developmental opportunity. Thus, plasticity of the brain in a young infant and child is a “double-edged sword”. It protects the brain from the neurologic consequences of destructive lesions and status epilepticus; however, recurrent seizures, or even frequent interictal epileptiform discharges, in this age group can produce permanent abnormal neuronal circuitry, resulting in long-term developmental delays and continued, intractable seizures [Holmes and Lenck-Santini, 2006]. In addition, chronic uncontrolled epilepsy in infants and children poses a significant risk for emotional, behavioral, social, cognitive, and family dysfunction. Population studies have demonstrated that epilepsy reduces life expectancy, and poorly controlled seizures further increase the risk of death in children and adults [Nashef et al., 1995; Hitiris et al., 2007]. In population-based studies, the estimated risk of sudden unexpected death in epilepsy (SUDEP) is estimated to be between 1:500 and 1:1000 per year. For those with uncontrolled epilepsy, the rate of SUDEP is approximately 1:200 per year. [Harvey et al., 1993c; Hauser et al., 1980; Meyer et al., 2010; So et al., 2009]. It should be noted that patients being evaluated for epilepsy surgery have the highest risk of SUDEP, estimated at between 2.2 and 9.3 per 1000 patient-years [Hiritiz et al., 2007].
Several studies have examined outcomes after epilepsy surgery. A majority of these studies has been performed in older adolescents and adults. The focus of these studies has been primarily on the improvement of seizure control, with 64–69 percent of patients having seizure-free outcomes [Engel, 1996; Wyllie, 1998; Wyllie et al., 1998]. These studies have placed less emphasis on improvement in quality of life – for example, enhancement of self-image, improvement in academic performance and psychosocial functioning, and increased independence in activities of daily living [Spencer, 1996; Taylor et al., 1997]. An increasing number of long-term follow-up studies have concentrated on infants and young children. Malformations of cortical development are the most frequently cited pathologic abnormalities in pediatric surgical patients [Duchowny et al., 1996; Wyllie et al., 1998; Harvey et al., 2008; Zupanc et al., 2010]. The overall outcome of pediatric epilepsy surgery in young infants and children is roughly comparable to that in the older adolescent and adult population. In infants, 61–65 percent have seizure-free outcomes [Chugani et al., 1993; Duchowny et al., 1998; Sinclair et al., 2003; Wyllie et al., 1996, 1998; Zupanc et al., 2010]. In young children, the rate of seizure-free outcomes varies, ranging from 58 to 74 percent [Paolicchi et al., 2000; Sinclair et al., 2003; Wyllie et al., 1998; Cossu et al., 2008; Kan et al., 2008; Kim et al., 2008; Zupanc et al., 2010]. The etiology of the epilepsy appears to play the major role in determining prognosis, regardless of location (temporal versus extratemporal). In one study, children with malformations of cortical development had a seizure-free outcome of 58 percent, whereas patients with other pathologies had a 77 percent seizure-free outcome. Temporal lobectomies were more commonly performed in our older adolescent patients, consistent with other studies, and demonstrated a seizure-free outcome of 84 percent. Patients who had modified lateral hemispherectomies also demonstrated a high seizure-free outcome (i.e., approximately 100 percent), even those with cortical dysplasias [Zupanc et al., 2010].
Several studies have reported on cognitive function after surgery in children who have undergone temporal lobectomy (predominantly older children and adolescents) [Gillam et al., 1997; Gleissner et al., 2002; Mabbott and Smith, 2004; Meyer et al., 1986; Szabo et al., 1998; Westerveld et al., 2000; Lah, 2004]. These studies have generally found that memory and intelligence are unchanged. Some reports note a decline in verbal memory and improvements in language, attention, and memory, while other studies have reported that good seizure outcomes have been associated with an increase in IQ [Lah, 2004]. Reports on the cognitive effects of extratemporal resections have been relatively few, in part because of the young age of those having surgery. In a follow-up study of 24 children operated on before 3 years of age, younger age at surgery was correlated with an improvement in developmental quotients [Loddenkemper et al., 2007]. In patients who have undergone successful frontal lobe resection, outcomes include improvements in attention and concentration but no change in executive functions, manual coordination, and language [Blanchette and Smith, 2002; Lendt et al., 2002]. Studies on postoperative psychosocial functioning in children have been relatively rare, almost exclusively retrospective, and based on subjective measures. In children who have undergone temporal lobectomies, studies indicate improvement in behavior, mood, and self-esteem, with the changes linked to improvement in seizure control [Danielsson et al., 2002; Davidson and Falconer, 1975; Duchowny et al., 1992; Meyer et al., 1986; Zupanc et al., 2010]. Of children who have undergone extratemporal resections, improved social behavior was found in about 50 percent [Adler et al., 1991]. Other retrospective studies that combine temporal and extratemporal cases suggest that reduction of seizure frequency, although not necessarily complete elimination of seizures, resulted in improved family life, socialization and behavior, and quality of life [Adler et al., 1991; Keene et al., 1998; Lendt et al., 2002; Mihara et al., 1994; Smith et al., 2004; Whittle et al., 1981; Zupanc et al., 1996, 2010]. Other reports indicate that a reduction in seizures may result in a favorable and significant improvement in the quality of life, with behavioral and developmental “catch-up” progress [Asarnow et al., 1997; Bourgeois et al., 1999; Chugani et al., 1990b; Duchowny et al., 1990, 1992; Wyllie et al., 1996; Jonas et al., 2004; Zupanc et al., 2010]. More recent studies indicate that shorter seizure durations and earlier surgical intervention result in better seizure outcome and quality of life [Jonas et al., 2004; Loddenkemper et al., 2007; Zupanc et al., 2010].
Historical Background
Epilepsy has always been a part of human existence. A generalized tonic-clonic seizure was first described in Akkadian, the oldest written language, more than 3000 years ago [Goldensohn et al., 1997]. Since that time, many descriptions of epilepsy appear in literature, including the Bible. In “On the Sacred Disease”, written in the 5th century, Hippocrates stated that epilepsy was a brain disease caused by an excess of phlegm that resulted in an abnormal brain consistency. He proposed diet and drugs as therapy [Scott, 1993]. Until the late 19th century, the treatment of epilepsy was surrounded by superstition, exorcism, magic, and alchemy. Caton’s (1842–1926) discovery in 1875 of spontaneous electrical activity of the brain and evoked potentials suggested that seizures might be the result of aberrant electrical activity in the brain [Caton, 1875].
The first effective treatment for epilepsy was potassium bromide, introduced in 1857 [Locock, 1857]. In 1886, Horsley performed the first epilepsy surgery on a patient with intractable post-traumatic epilepsy. Several decades later, the antiepileptic drug phenobarbital was introduced in 1912, followed by phenytoin in 1937. Epilepsy surgery did not advance until 1950, when Penfield published his article on 70 cases of temporal lobectomy [Flanigin et al., 1991; Penfield and Flanigin, 1950]. His neurosurgical career was devoted to the study of seizure semiology (i.e., clinical description) and its correlation with the brain cortex. He used cortical mapping and stimulation in much the same way in which it is used today. He also recognized the substrates of epilepsy, particularly trauma and infection. His seminal clinical research has been instrumental in guiding the hands of contemporary epileptologists and neurosurgeons interested in the surgical approach to epilepsy.
Epilepsy surgery was not regarded as a conventional treatment for intractable epilepsy until recently. In the past 30 years, dramatic improvements in brain imaging that identify specific anatomic substrates of epilepsy have sparked renewed interest in epilepsy surgery. Temporal lobectomy with amygdalohippocampectomy has become the standard of care in adult patients with intractable epilepsy emanating from the temporal lobe. The surgical success rate for a seizure-free outcome in these carefully selected patients approaches 80–90 percent [Duchowny et al., 1992; King et al., 1986; Penfield and Flanigin, 1950]. In a randomized, controlled trial of surgery for temporal lobe epilepsy compared to treatment with antiepileptic drugs, 58 percent of patients demonstrated seizure freedom after 1 year compared to only 8 percent of patients treated with medications [Wiebe et al., 2001]. Unfortunately, surgical success does not necessarily translate to an improved quality of life. The accumulation of years of low self-esteem, loss of independence, poor peer relations, and academic failure, coupled with high financial costs, often without benefit of full insurance coverage, translates to continued lack of employment and depression [Reeves et al., 1997]. The lifetime cost of epilepsy for an estimated 181,000 people with onset in 1995 is projected at $11.1 billion, and the annual cost for the estimated 2.3 million people with epilepsy is estimated at $12.5 billion [Bazil, 2004; Begley et al., 1994, 2000; Hathaway et al., 1995]. In one recent study, the calculated total aggregated annual economic impact of epilepsy on the U.S. economy was $9.6 billion in direct medical costs. This analysis did not consider the indirect costs of loss of productivity, quality of life, and comorbidities [Yoon et al., 2009]. With respect to children, it is estimated that the annual cost of medical care for a child with epilepsy is $6379, compared to $1032 for peers without epilepsy [Yoon et al., 2009]. Indirect costs probably account for 80–85 percent of the total costs, and include delayed or missed educational opportunities, psychiatric and social service needs, and lost employment. The direct costs of epilepsy are concentrated in the patients with intractable epilepsy. The growing recognition of the real costs of epilepsy – medical, psychological, educational – has led to increased interest in the early identification of children who might benefit from epilepsy surgery [Jalava et al., 1997]. In older children and adolescents, temporal lobectomies are common epilepsy surgical procedures [Harvey et al., 2008]. In younger children and infants, however, extratemporal resections, including multilobar resections and hemispherectomies, are the more typical procedures [Harvey et al., 2008].
In recent studies, it has been shown that the costs of epilepsy surgery are offset by a decline in health-care costs after successful surgery. One study documented that the total costs for adult patients who were seizure-free following epilepsy surgery declined by 32 percent by 2 years following surgery. In the 24 months after surgery, epilepsy-related costs were $2068–2094 in patients with persisting seizures, as opposed to $582 in patients who were seizure-free following surgery [Langfitt et al., 2007]. In addition, in adult patients, it appears that epilepsy surgery increases quality-adjusted life expectancy by 7.5 years [Choi et al., 2008]. In the pediatric population, additional benefits of epilepsy surgery appear to be improved long-term developmental outcomes and quality of life [Loddenkemper et al., 2007; Zupanc et al., 2010].
Indications for Epilepsy Surgery
Criteria have been proposed for referral and evaluation of children for epilepsy surgery, although there is currently insufficient class I evidence to produce a practice guideline [Cross et al., 2006]. Practice guidelines for temporal lobe and localized neocortical resections for epilepsy have been proposed for adults [Engel et al., 2003]. In determining whether a child is a candidate for epilepsy surgery, several key issues must be considered. The decision-making task must take into account the following:




The proper classification of seizure type and epilepsy syndrome is crucial in the determination of whether or not a patient is an appropriate epilepsy surgery candidate [Aicardi, 1994; Holmes, 1993]. The benign seizure disorders, such as benign rolandic epilepsy or benign epilepsy with centrotemporal spikes, must be recognized. With rare exceptions, these syndromes usually are easily treated, and affected patients do not present to tertiary epilepsy centers.
Children with Sturge–Weber syndrome who have frequent, medically refractory seizures accompanied by progressive hemiparesis and cognitive impairment should be evaluated promptly for hemispherectomy [Thomas-Sohl et al., 2004; Vining et al., 1997]. Clinical outcome studies indicate that early surgical resection can result in the elimination of seizures, improvement in cognitive abilities, and overall improvement in quality of life, despite hemiparesis and visual field defect as residual neurologic deficits [Erba and Cavazzuti, 1990; Hoffman et al., 1979; Ogunmekan et al., 1991; van Empelen et al., 2004].
Children with hemimegalencephaly, a unilateral or focal malformation of cortical development, can present in infancy with multiple daily seizures, developmental stagnation or decline, and hemiparesis. Hemispherectomy provides relief from seizures (especially in those patients with unilateral epileptiform abnormalities) and improved developmental outcome [Andermann et al., 1993; Vigevano and DiRocco, 1990; Vigevano et al., 1989; Jonas et al., 2004]. The patients with symptomatic infantile spasms who have underlying focal cortical dysplasias, usually temporal-parietal-occipital, should be considered for early focal cortical resection. University of California at Los Angeles investigators have provided the seminal clinical research in this area and have documented a significant improvement in seizure control and enhanced developmental gains following epilepsy surgery, greater than would have been predicted using the natural history of infantile spasms as a comparison [Asarnow et al., 1997; Chugani et al., 1990a, 1993; Duchowny et al., 1990].
Rasmussen’s encephalitis is characterized by intractable focal motor seizures, often evolving into epilepsia partialis continua, cognitive decline, and progressive hemiparesis. Recent findings of glutamate receptor antibodies in some patients with Rasmussen’s encephalitis implicate a possible autoimmune pathophysiology [Antel and Rasmussen, 1996; Pardo et al., 2004; Rogers et al., 1994]. Although initial trials of intravenous immunoglobulin and plasmapheresis have been encouraging, long-term studies have not confirmed efficacy [Andrews et al., 1996; Hart et al., 1994; Krauss et al., 1996]. Therefore, the only definitive treatment for Rasmussen’s encephalitis remains hemispherectomy [van Empelen et al., 2004; Jonas et al., 2004].
In addition to those with the catastrophic epilepsies of infancy and childhood, all children with tumors and concomitant localization-related epilepsy should be considered for early surgical intervention. Compelling reasons for such intervention exist. Most tumors need to be biopsied or excised. Additionally, although the tumors associated with epilepsy usually are slow-growing, cortical, and well circumscribed, some tumors, especially astrocytomas, are not necessarily benign and can undergo malignant change [Jack, 1995]. Without resection, the natural history of these tumor-associated epilepsy syndromes is one of continued seizures with little hope of remission. Antiepileptic drugs produce side effects that can affect cognitive function and behaviors, with concomitant impact on psychosocial development [Meador, 2002] Examples of tumors that usually are easily resectable are the gangliogliomas and dysembryonic neuroectodermal tumors, which have a predilection for the temporal lobe (Figure 61-1) [Duchowny et al., 1992; Tice et al., 1993; Vali et al., 1993].
Children with other types of lesional symptomatic localization-related epilepsy also should be considered as epilepsy surgery candidates. Common substrates of epilepsy include encephalomalacias, vascular malformations, tubers, and malformations of cortical development [Harvey et al., 2008; Zupanc et al., 2010].
Patients who have generalized epilepsy syndromes may also be candidates for epilepsy surgery. The presence of generalized or multifocal epileptiform discharges on surface EEG monitoring should not necessarily exclude someone from epilepsy surgery. Children with generalized or multifocal epilepsy should be considered for epilepsy surgery, if data suggest an underlying focal generator for the epileptic condition [Wyllie, 1995; Wyllie et al., 2007; Zupanc et al., 2010]. Specifically, in the presence of a lesion on magnetic resonance imaging (MRI), the epilepsy syndrome is most likely to be due to a symptomatic localization-related epilepsy with rapid secondary bisynchrony. The mechanisms of underlying generalized epileptiform discharges in focal cerebral lesions can be seen as a form of “maladaptive plasticity” of the immature brain, whereby the lesions in the immature neural network of the young brain permanently alter the neural circuitry, producing spontaneous hypersynchrony and generalized discharges [Sutula, 2004]. These generalized discharges may also involve the thalamocortical network, resulting in generalized rhythmic discharges [Van Hirtum-Das et al., 2006]. Approximately 10–15 percent of children with Lennox–Gastaut syndrome, one of the most common symptomatic “generalized” epilepsy syndromes, have underlying focal malformations of cortical development and should be evaluated carefully for epilepsy surgery. In one study, children who had generalized discharges and focal lesions had identical seizure-free outcomes (72 percent seizure-free) to children with similar lesions and ipsilateral focal epileptiform discharges [Wyllie et al., 2007]. In addition, children with intractable epilepsy who have tonic-atonic seizures associated with generalized spikes/polyspikes (usually Lennox–Gastaut syndrome) and no identifiable lesion on neuroimaging may respond to a complete corpus callosotomy, a palliative treatment that can have a significant impact on quality of life [Wyllie et al., 1993; Zupanc et al., 2010]. An alternative approach is that of a multistaged epilepsy surgery, initially performing a complete corpus callosotomy, then placing lateralizing strip electrodes with the hope of identifying epileptogenic cortex that can be resected. In one study of 14 patients undergoing this approach, 9 went on to have a focal cortical resection and 5 out of 9 (56 percent) were seizure-free [Zupanc et al., 2011).
Patients with tuberous sclerosis and medically refractory, symptomatic, localization-related epilepsy should also be considered for epilepsy surgery. In patients with multiple tubers, emerging neuroimaging techniques, particularly interictal α-[11C]methyl-l-tryptophan (AMT) positron emission tomography (PET) scans, offer promise in identifying the most highly epileptogenic tuber [Asano et al., 2000; Chugani et al., 1998; Juhasz et al., 2003]. If the presurgical evaluation points to a specific tuber, studies have shown that it can be successfully removed, with a significant improvement in seizure control [Bebin et al., 1993; Koh et al., 2000; Romanelli et al., 2004]. There is also precedent for removing multiple tubers in the brain, as several tubers may be producing medically refractory seizures [Weiner et al., 2006].
In summary, factors that favor early intervention with epilepsy surgery include the following:
Even if the lesion is outside the temporal lobe, in carefully selected patients, epilepsy surgery can generally be performed with little risk of neurologic sequelae and a high rate of surgical success [Britton et al., 1994; Cascino et al., 1990, 1992, 1993, 1994; Montes et al., 1995; Paolicchi et al., 2000; Zupanc et al., 2010].
The suspected central nervous system pathology, based on MRI, may also have an impact on whether or not a patient is an epilepsy surgery candidate. For example, patients with low-grade tumors, infarctions, and mesial temporal sclerosis are likely to undergo remission of their epilepsy and typically have an excellent seizure-free outcome. If the epilepsy is temporal in onset and pathologic features include associated hippocampal formation atrophy or mesial temporal sclerosis, the surgical success rate approaches 80–90 percent [Cascino et al., 1993; Sinclair et al., 2001] (Figure 61-2). Data from our own retrospective study and from the work of Palmini and co-workers suggest that malformations of cortical development, no matter where the cortical location, carry a significant risk for status epilepticus and intractability [Laoprasert et al., 1997; Palmini et al., 1997]. Epilepsy surgery in patients with underlying malformations of cortical development have a lower seizure-free outcome, but it is still close to 60 percent, which is significantly better than additional trials of antiepileptic medication. Patients with mild malformations of cortical development, such as focal cortical dysplasia (FCD) type 1a, fared better than patients with more severe malformations of cortical development, such as FCD type 2a [Fauser et al., 2004]. In addition, the patients with known central nervous system lesions can be identified early, with detailed MR imaging.
Preoperative Evaluation
Techniques and Technologies
Seizure Semiology
Seizure semiology can provide insightful clues to the lateralization and localization of the underlying epileptogenic focus. The presence of versive head movements, unilateral motor clonic activity, and eye deviation may constitute critical lateralizing information. Specifically, versive head movements indicate that the epileptogenic zone resides in the contralateral hemisphere. In similar fashion, seizures consisting of olfactory or gustatory hallucinations, followed by complex motor automatisms and staring unresponsively, are virtually diagnostic of involvement of the temporal lobe. These seizures generally are seen in older children or adolescents, but also may occur in younger children. It should be noted, however, that seizures emanating from the temporal lobe in infants and young children commonly are associated with behavioral arrest, motor dystonic posturing, and fewer automatisms [Brockhaus and Elger, 1995; Jayakar and Duchowny, 1990; Wyllie et al., 1993]. Additionally, young children are typically incapable of describing the premonitory symptoms before the onset of the more overt clinical seizure. Video EEG monitoring has been helpful in fully elucidating the seizure semiology in these patients. Children with infantile spasms may have partial seizures before, during, or after the onset of the infantile spasms [Kobayashi et al., 2001; Watanabe et al., 2001]. Partial seizures can be a helpful clue that prompts the epileptologist to screen carefully for an underlying focal abnormality, such as a tuber or focal cortical dysplasia.
Electroencephalography
In the adult preoperative evaluation, prolonged video EEG monitoring provides the baseline data with which all of the other data are compared. In children, however, it is becoming increasingly clear that the surface EEG data may be poorly localized and at times misleading in the preoperative evaluation [Wyllie, 1995]. Therefore, this modality may be less important in localizing the epileptogenic focus. In these children, other modalities, particularly neuroimaging studies (MRI, MR spectroscopy, PET, and SPECT), may provide the pivotal information that determines the location of the epileptogenic zone and may reduce the need for invasive subdural EEG monitoring. Indeed, in the near future, the improvement of noninvasive functional brain imaging techniques may obviate the need for invasive EEG monitoring. Even now, invasive EEG monitoring may be deferred if surface EEG monitoring and MRI data are congruent [Wyllie et al., 1998; Zupanc et al., 2010].
Magnetic Resonance Imaging
MRI scans have greatly enhanced the ability to visualize intraparenchymal brain structures. The linkage between intracranial abnormalities and epilepsy is well accepted [Zupanc, 1997a]. The mechanism(s) involved in the production of epilepsy is an area of intense research and involves structural changes, synaptic reorganization, stimulation of mossy fibers, astroglial proliferation with neuronal cell loss, and neurotransmitter or corresponding receptor changes. With the recent advances in MRI technology, the ability to identify the substrates of epilepsy has been greatly enhanced. This modality provides some of the most sensitive and specific neuroimaging data for localization of the epileptogenic zone [Brooks et al., 1990; Cascino, 1994; Cascino et al., 1989, 1991; Kuzniecky et al., 1993a, 1993c; Madan and Grant, 2009]. The following new technologies are exciting and innovative [Jack, 1995; Madan and Grant, 2009]:





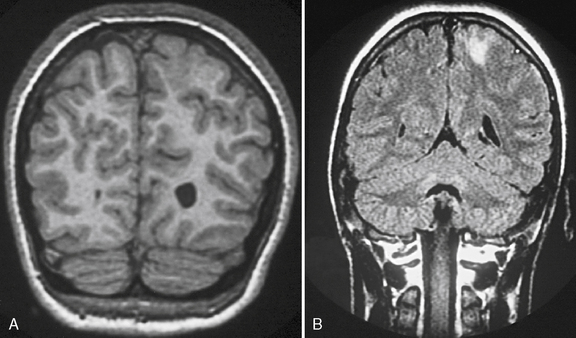
Fig. 61-3 MRI scans from a patient with focal cortical dysplasia of the posterior left parasagittal region.
With use of these techniques, MRI scans can identify many substrates of epilepsy, including malformations of cortical development, tumors, vascular malformations, and encephalomalacias secondary to trauma, infection, and infarction. Malformations of cortical development are increasingly recognized as being highly epileptogenic [Kuzniecky and Ruben, 1995; Kuzniecky and Barkovich, 2001; Palmini et al., 1995; Raymond et al., 1995]. Advances in MRI technology have greatly improved the ability to identify these abnormalities. They may account for more than 60 percent of the intractable localization-related epilepsies of childhood [Kuzniecky et al., 1993c]. These malformations can be small and difficult to detect, even with sophisticated MRI scans of the brain, or can be widespread and diffuse, as with lissencephaly [Dobyns and Truwit, 1995; Dobyns et al., 1996] (Figure 61-5 and Figure 61-6). The unilateral and focal malformations of cortical development are most often targeted for surgical excision. Clinically, information on the natural history of the malformations of cortical development is emerging. Status epilepticus is a common initial presentation, usually in the latter half of the first decade of life [Laoprasert and Zupanc, 1997]. Many of the malformations of cortical development produce an epilepsy syndrome that is intractable to medical management [Palmini et al., 1997].
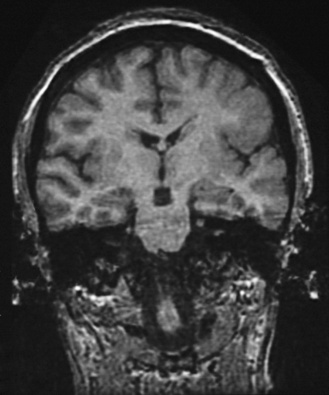
Fig. 61-6 MRI scan from a patient with unilateral perisylvian dysplasia with polymicrogyria (left hemisphere).
Finally, although mesial temporal sclerosis and hippocampal atrophy are not commonly found in children with intractable epilepsy who are younger than 10 years of age, identification of these abnormalities is a powerful indicator of the zone of epileptogenesis [Cascino, 1994; Cascino et al., 1991; Jack, 1995; Swartz et al., 1992]. The pathophysiology of mesial temporal sclerosis and hippocampal formation atrophy is poorly understood. Do the seizures themselves cause mesial temporal sclerosis and hippocampal formation atrophy? Does an underlying malformation of cortical development cause the initial seizures, ultimately resulting in mesial temporal sclerosis and hippocampal formation atrophy [Cendes et al., 1993; Kuks et al., 1993; Trenerry et al., 1993]? Prolonged febrile seizures, head injury, nonfebrile status epilepticus, encephalitis, hypertensive encephalopathy, and viruses, including human herpesvirus 6 (HHV6), have also been implicated as potential causes of mesial temporal sclerosis [Scott et al., 2001; Solinas et al., 2003; Donati et al., 2003; Theodore et al., 2008]. These questions have not yet been clearly answered. It is known, however, that the degree of volume loss correlates with the amount of cellular loss, as measured in pathologic specimens [Cascino et al., 1991]. The neuronal cell loss, coupled with the presence of aberrant mossy fibers, and synaptic reorganization and excessive glutamatergic activity, probably accounts for the recurrent, recalcitrant seizures [Fuerst et al., 2003; Holmes and Ben-Ari, 1998; Jokeit et al., 1999; Kalviainen et al., 1998; Kotloski et al., 2002; Sutula et al., 1988, 1989; Tasch et al., 1999; Eid et al., 2008].
Co-registration of scalp EEG and MRI data has become an important tool in the determination of source localization relative to the patient’s brain anatomy [Lamm et al., 2001]. This technique provides a three-dimensional rendition of the topographic EEG activity on to the patient’s head, taking into consideration anatomical differences specific to the patient. More recently, co-registration of the intracranial EEG and MRI data has provided the epileptologist and neurosurgeon with a three-dimensional map of electrode placement used to define the epileptogenic zone, and hence, the boundaries of surgical resection [LaViolette et al., 2011] (Figure 61-7).
Single-Photon Emission Computed Tomography
SPECT also has enhanced the ability to identify the epileptogenic zone. Penfield and colleagues observed relative hyperperfusion in the epileptogenic zone during a seizure [Penfield, 1958]. Interictally, blood flow and metabolism decrease. SPECT scan technology enables quantification of cerebral blood flow and identification of areas of relative blood flow change. SPECT images are reconstructed from data obtained by recording photon emissions from radiotracers injected intravenously. These radiotracers rapidly cross the blood–brain barrier because of their lipophilic nature and bind within minutes to the brain, producing an instantaneous picture of cerebral blood flow [English and Brown, 1990]. Clinical research has focused on both interictal and ictal SPECT scans, with a substantial portion of the clinical research using the radioisotope 99mtechnetium-hexamethylpropyleneamine oxime (99mTc-HMPAO) [Cross et al., 1995, 1997; Harvey et al., 1993a, b]. More recently, 99mTc ethyl cysteinate dimer (ECD) (i.e., 99mTc-N,N′(1,2-ethylenediyl)bis-l-cysteine diethyl ester), prepared as technetium 99mTc bicisate (Neurolite), has been introduced [Lanceman et al., 1997]. Logistically, Neurolite provides distinct advantages for ictal SPECT scans because it is a stable isotope tracer that can be mixed well ahead of the time of injection, as opposed to 99mTc-HMPAO, which decomposes quickly in vitro and must be used less than 30 minutes after it is reconstituted. For ictal SPECT scans, a technologist or nurse trained in the delivery of these radioisotopes can sit at the bedside and deliver the Neurolite within seconds after the onset of a seizure. Spatial resolution with SPECT scans also has improved because of the development of gamma cameras with multiple detectors that provide more data points, with subsequent enhanced sensitivity.