Chapter 47 Endotracheal Tube and Respiratory Care
II. Properties of the Endotracheal Tube
III. Endotracheal Tubes and Other Airway Adjuncts
IV. Proper Safeguarding of the Airway
V. Maintenance of the Endotracheal Tube
I Introduction
The earliest recorded use of airway manipulation with an artificial device dates back to early Roman civilization when Asclepiades performed a tracheostomy for laryngeal edema. Today it is clear that the role of the endotracheal tube (ETT) in medicine is as invaluable as that of any other medical device created to date. The establishment of a definitive airway via the ETT in both elective and emergency situations has allowed for the delivery of immediate life-sustaining therapies during resuscitation, the maintenance of oxygenation and ventilation in prolonged illness, and the (temporary) delivery of inhaled anesthesia.1 This chapter begins with a brief history of the development of the ETT. It describes the various ETTs available along with their indications for use and respective limitations. It reviews basic airway anatomy with regard to ETT placement, proper positioning and stabilization of the ETT, and complications attributed to its use. Finally, it addresses respiratory care of the intubated and mechanically ventilated patient.
II Properties of the Endotracheal Tube
A Anatomy of the Endotracheal Tube
Between the time of Asclepiades and the present, ETTs have been constructed of a variety of materials, including reed, brass, and steel. Eventually, in 1917, Magill and Rowbotham manufactured them from rubber for the purpose of administering anesthesia.2 In 1928, when Guedel and Waters added a protective cuff to prevent aspiration, the modern ETT was born. Rubber, however, had limitations in this application, such as increased stiffness with rising temperature and limited adhesive properties with different polymers, which required the cuffs to be manufactured from the same polymer as the tube.3 These shortcomings led to the search for alternative materials. In 1967, polyvinyl chloride (PVC) was popularized by Dr. S. A. Leader, and it has since been the material most commonly used. One property that makes PVC attractive is that it provides stiffness to an ETT at room temperature to assist with intubation yet becomes more malleable with the increased temperature in situ. Other properties include the ability to embed radiopaque lines in the material to assist with positioning and recognition on a radiograph. Because it accepts many materials, the addition of an exteriorized inflation line to connect the pilot balloon to the cuff can allow for varied cuff materials. Finally, it simply is much lower in cost than other available materials.3
The 15-mm adapter allows for universality between ventilating devices such as a bag-mask ventilation system, anesthesia circuit, or ventilator circuit. The adapter fits ETTs as large as 12 mm internal diameter and as small as 3 mm, thereby providing further commonality among multiple ETTs and ventilating devices. Having one standard size also allows for interchange between devices made for tracheostomies or ETTs. The adapter is removable to allow for passage of intraluminal devices (e.g., bronchoscope, suction catheter) or to allow passage of the ETT via a supraglottic airway device such as a laryngeal mask airway (LMA, LMA North America, San Diego, CA). Adapter removal may facilitate the extraction of extensive biofilm accumulation or mucus plugs. Additionally, some clinicians choose to resize (shorten) the ETT.4
The cuffs on the early ETTs were, like the tubes themselves, composed of rubber. The rubber ETT cuffs had limitations such as the need for elevated inflation pressures (high pressure, low volume [HPLV]) to fill the cuff and occlude the airway surrounding the ETT. These high inflation pressures result in the transmission of high lateral pressures to the tracheal wall, albeit in a very minimal contact area, to maintain a seal. The trachea is not circular but rather D–shaped. HPLV cuffs inflate in a circular manner, thereby altering the structure of the trachea; the high pressure exerted on the tracheal wall impairs capillary pressure and possibly results in greater mucosal ischemia.5 The most commonly used HPLV cuffs in today’s practice are the reusable silicone ETTs found with intubating LMAs (ILMAs) (Fig. 47-1). Caution should be exercised when using these ETTs for prolonged periods, given their inherent risk of tracheal mucosal damage. The introduction of PVC-based cuffs reduced this problem because the cuff wall was more supple and thinner, allowing the cuff to accommodate high volume and low pressure (HVLP) and thus providing an adequate seal with lower lateral wall pressures.3,5,6 The main value of the HVLP cuff is its ability to conform to the irregular borders of the trachea.7–9 Polyurethane is even thinner and more pliable, with increased tensile strength, allowing for higher volumes, larger contact areas, and minimal mucosal pressures.10 Foam-based cuffs exist and provide maximal conformation to the tracheal walls, but they do little for the prevention of microaspiration.9
Remodeling of the cuff has been particularly driven by the desire to improve prevention of ventilator-associated pneumonia (VAP), and the shape of the cuff has also been altered. The Mallinckrodt TaperGuard Evac ETT (Covidien, Boulder, CO) is a new option that has been demonstrated in randomized, controlled trials to reduce microaspiration by as much as 83%, compared with traditional HVLP barrel shaped cuffs (Fig. 47-2).8 It is postulated that a barrel-shaped tube tends to wrinkle and fold in an attempt to conform to the tracheal wall, allowing small channels for potential microaspiration, whereas the bulbous, conical shape of the TaperGuard ETT may reduce wrinkling and thus decrease the incidence of microaspiration. Continued work in this area may lead to improved tracheal wall sealing capabilities at safe levels of pressure while minimizing potential pathways for the translocation of oronasal and gastric secretions, which is thought to be the prime etiologic pathway for VAP.11
The pilot balloon of an ETT functions as an indirect volume gauge for the ETT cuff, relative to the amount of air located in the cuff (inflated or deflated). The pilot balloon does not provide information about the absolute volume insufflated or the pressure exerted on the tracheal mucosa. When a pilot balloon fails or is an impediment to an intubation, options are generally limited to a tracheal tube exchange.12–14 Pilot balloon failures have multiple causes. Shearing along the ETT connection (usually due to contact with dentition), cracked inflation valves (from syringe manipulation or trauma), material aging, and pilot tubing laceration due to biting all cause the ETT cuff to lose air over time.15–18 Simple techniques have been described to replace a pilot balloon in a variety of clinical situations using equipment readily available in the operating room. Needles or intravenous catheters with stopcocks or claves, epidural clamp connectors, and commercially available repair kits (Fig. 47-3) provide reliable substitutions for incompetent pilot balloons when they are connected to the pilot-cuff inflation line.19,20 The procedure for replacing an incompetent valve is as follows: cut the inflation tube distal to the pilot balloon; insert a needle or intravenous catheter into the cut end (or affix the hub of an epidural catheter to the cut end); and use a stopcock or clave (an item capable of stemming the entrance of air) on the needle or catheter after insufflation, paying careful attention not to overinflate.
B Development and Properties of the Endotracheal Tube
The purpose of the ETT has always been the same, and it has always had the same inherent problems. Technology continues to advance the standard ETT for improved function and decreased physiologic insult. Rather than compensate for the resistance produced by a rubber or PVC ETT, a newly designed ETT has been produced of ultrathin polyurethane that is reinforced with wire to resist collapsing and kinking. This wire is unique in that it has an elastic shape memory to prevent deformation. The internal diameter is increased without compromising the rigid shape of the ETT. The result is a tube with a resistance similar to that of the upper airway that is lighter, offers less airflow resistance, and, when compressed, forms an egg shape rather than an oval.21 Experimentally, this new design has been shown to decrease inspiratory and expiratory resistance by 60% each and the inspiratory, expiratory, and total work of breathing (WOB) by 70%, 47%, and 45%, respectively.22,23
The use of the ETT continues to expand. No longer is it expected to be simply a conduit for ventilation. As the technology has advanced, the original ETT has steadily been outfitted with a host of successful innovations to improve patient care, whether for convenience or necessity. For example, modifications to the cuff to improve occlusion of the trachea in an effort to prevent microaspiration, coupled with an extra subglottic suctioning port (and other patient care maneuvers), have served to vastly reduce the incidence of VAP.7–9 Another example is the modification of the surfaces of the ETT to minimize bacterial adhesion and thereby minimize biofilm accumulation.24 As for bells and whistles, there are ETTs with fiberoptic cameras distally, allowing for ease of placement and the possibility of continued intratracheal surveillance. Another example is the addition of multiple sensors, for so-called bioimpedance cardiography, that are capable of monitoring stroke volume variation, cardiac output, systemic vascular resistance, and arterial pressures (due to the close proximity of the ETT and the aorta) and thereby, at least theoretically, preventing the need for further invasive technologies. Continued study of these modifications may provide justification to adapt these technologies to patient care.
D Complications of Endotracheal Tube Placement
Complications associated with ETT placement should be grouped into three major subcategories: those that occur at intubation, those that occur with the ETT in situ, and postextubation sequelae.25 The problems associated with placement are numerous and can be worsened in emergencies, with multiple attempts, use of a variety of devices, or inexperience of the operator.26 Problems at placement include dental and oral problems, maxillofacial damage, displacement of the arytenoid cartilages, vocal cord ulceration or dysfunction, airway perforation, autonomic hyperactivity, and, of course, failed intubation. Structural damage is unlikely to be repaired until the patient no longer requires intubation, unless the damage interferes with ventilation and oxygenation. Problems that occur as a result of an in situ ETT include those related to the ETT, such as aspiration, vocal cord paralysis, or transient nerve palsy; ulceration and granuloma formation in the trachea and on the cords; tracheal synechiae; subglottic stenosis; laryngeal webbing; tracheomalacia; tracheoesophageal, tracheoinnominate, or tracheocarotid fistula; and recurrent and superior laryngeal nerve damage.27 Other complications are related to mechanical ventilation facilitated by the ETT and include aspiration, barotrauma (pneumothorax, pneumomediastinum), VAP, and dislodgement.28 Finally, postextubation complications can lead to long-term morbidity or the urgent need for reintubation. Many of the postextubation culprits have already been encountered as complications of ETT placement or presence, particularly subglottic stenosis, vocal cord injury, and hoarseness.28,29
III Endotracheal Tubes and Other Airway Adjuncts
A Choice of Endotracheal Tube Size
In selecting an ETT, consideration must be given to the functional reason for placement as well as patient-specific factors such as body height, gender, airway integrity, airway pathology, and previous airway manipulation or instrumentation. Theoretically, short-term placement for anesthesia should be different than placement for prolonged support with mechanical ventilation or for fiberoptic bronchoscopy to aid therapy. Generally, the trachea of an adult female accepts a tube of 7.5 to 8.0 mm and that of a male accepts one of 8.5 to 9.0 mm, but typically a 7.0-mm ETT is used for females and an 8.0-mm tube for males, at least in the United States. It is also generally accepted that an ETT of at least 8.0 mm is needed for competent use of an adult-based bronchoscopic investigation.30
1 Small Tubes and Airway Resistance
The physics of laminar gas flow through a conduit are described by the Hagen-Poiseuille equation, which reflects the relationship of resistance varying inversely with the fourth power of tube radius. Despite the fact that gas flow through ETTs is often turbulent rather than laminar, the effect on resistance to gas flow represented by each millimeter decrease in tube size is considerable, ranging from 25% to 100%.31 Airway resistance is affected by more than tube diameter: the presence of secretion within the tube, ETT kinking, and positioning of the head and neck can also increase the tendency for turbulent flow.32,33 The fundamental principle of which to be mindful is that airway resistance induced by an ETT is inversely proportional to the tube size—hence, the mantra that the largest tube is usually the best size.34
Airway resistance increases with decreasing ETT diameter, whether due to internal occlusion, smaller size, or external compression. As airway resistance increases, WOB also increases.31 The increase in WOB associated with a 1-mm reduction in ETT diameter varies in accordance with tidal volume and respiratory rate at a given minute ventilation and can range from 34% to 154%.31 When ventilation is controlled, the increase in WOB related to ETT resistance is seldom of any consequence, because it is overcome by ventilator adjustments. However, small-diameter tubes create greater difficulty for patients in weaning from ventilatory support due to the higher levels of resistance encountered when attempting to breathe spontaneously.35,36 It has been suggested that an inability to spontaneously ventilate due to the increased WOB imposed by a 7-mm ETT might indicate that extubation will fail regardless of tube size.37,38
Increased airway resistance associated with a smaller-diameter ETT may also be associated with inadvertent PEEP. Patients with high oxygen consumption, increased carbon dioxide production, or ventilation-perfusion relationships that produce high dead space ventilation often require higher minute ventilations to achieve appropriate ventilation and oxygenation. The gas flows necessary to maintain such a minute ventilation are also quite high, and the resistance imposed by a smaller-diameter ETT further prohibits the completion of expiratory flow before initiation of the subsequent inspiration. This breath stacking results in air trapping and unwanted PEEP, magnifying the risk of mechanical ventilation because barotrauma and subsequent intrathoracic overpressure could result in circulatory compromise.39
The restriction to gas flow through any ETT increases dramatically when devices such as a suction catheter or bronchoscope are placed in the lumen. The cross-sectional area of the tube is effectively reduced by an amount equal to the cross-sectional area of the device inserted into the tube. The limitation of gas flow has consequences for both the inspiratory and expiratory phases: inspiratory flow may be inadequate to maintain oxygenation and ventilation during the procedure, and retarded expiratory flow may lead to overdistention resulting in barotrauma or circulatory compromise.40
2 Large Tubes and Trauma
Whereas smaller-diameter ETTs have disadvantages related to gas flow and airway resistance, larger tubes are more frequently associated with traumatic placements and damage to both the laryngeal structures and the tracheal mucosa.37,38,41 Larger ETTs are associated with a higher incidence of sore throat after general anesthesia, compared with smaller-diameter tubes, but this difference is relatively negligible with long-term intubation.42 With prolonged intubation, laryngeal trauma is more likely. Women, because of the inherently smaller size of their airway, are more susceptible to injury than men.43,44
Laryngeal structures at particular risk for trauma are the arytenoid cartilages and the cricoid cartilage. Trauma results not only from the shape discrepancy between the round ETT and the angular, wedge-shaped glottic opening but also from direct contact and pressure on these structures and from repetitive tube movement, which leads to ulceration or erosion of the protective mucosa.44–46 Tracheal mucosal injury can also occur because of the irregular surfaces created by wrinkling and folding of the ETT cuff or the externalized pilot tube used to fill the ETT cuff. If the tracheal lumen is “overcrowded,” airway injury is more likely to occur when large tubes are used and little cuff volume is required to seal the airway.47
B Potentially Beneficial Alternatives to the Standard Endotracheal Tube
1 Preformed and Reinforced Tubes
Rigid, preformed tubes such as those developed for long-term use in tracheostomy were known to maintain their patency despite the need for angulation. Preformed tubes have been developed for specific application in anesthesia practice as well. The Ring-Adair-Elwyn (RAE) tubes (Mallinckrodt Inc., Pleasanton, CA), both oral and nasal (Fig. 47-4), maintain a fixed contour similar to the average facial profile, allowing for head and neck surgery while minimizing surgical field interference. Their contour also reduces the risk of pressure injury to the posterior pharynx when repositioning is desired. The intra-airway length is tied to the size of the ETT, with a relatively appropriate depth based on the average size of a patient for whom the tube might be selected.48,49
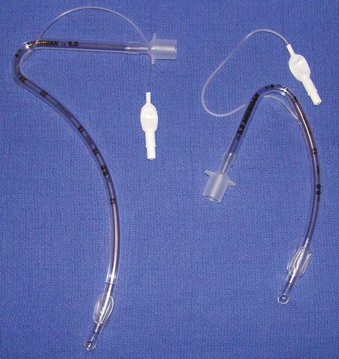
Figure 47-4 Nasal (left) and oral (right) Ring-Adair-Elwyn (RAE) preformed endotracheal tubes.
(Courtesy Covidien, Mansfield, MA.)
The embedded wire concept of the armored ETT has also been developed for long-term tracheostomy use. Although the armored tracheostomy tube is not free of risks, one advantage is that its flexibility allows its length and intratracheal depth to be adjusted, which may be beneficial if tracheomalacia at the level of the cuff develops.50 These tracheostomy tubes are also popular for use in morbidly obese patients, in whom, because of the depth of tissue, preformed tracheostomy tubes may not have the shape required to fit an individual patient. One major consequence of this type of reinforced ETT may occur when external pressure is applied to the wire-reinforced component (i.e., by patient biting). Once a compression threshold is reached, the luminal support provided by the wire may be compromised, and a permanent, irreparable dent remains that can significantly endanger ventilation and suctioning capabilities.
2 Laser Tubes
Progress in laser technology has advanced surgical capabilities, particularly for airway surgery. To protect patients and health care providers from laser-induced injury to eyes and airways, special precautions are required. Fire is the most serious danger associated with the use of lasers in the operating room, especially when a laser is used in airway surgery.30,51–53 A major complication related to the use of lasers for laryngeal surgery is ignition of the ETT.54 The laser beam may ignite the tube by direct penetration or indirectly if burning tissue is inhaled into the tube.30,51,52,55 The ease of ignition is related to the ETT material, the concentration of oxygen in use, and any other adjunctive materials or gases that could support combustion.30,52,55 Most ETTs are constructed of PVC, which is highly flammable. Ideally, PVC tubes should not be used for airways when a laser is employed.51,55,56
ETTs can be laser-proofed or protected from the laser beam by wrapping them with either reflective metal tape or muslin. Ideally, they should be constructed from noncombustible materials. In particular, the ETT cuff is vulnerable to puncture by the laser beam and should be filled with saline or water, which allows more energy to be absorbed before disruption.30,52,55 One trick to enhance appreciation of a penetrated, defective cuff, is to place a dye indicator, such as methylene blue, into the solution that is instilled into the cuff. Any leakage will clearly mark the airway and alert the provider to the potential dangers.57 Protecting the tube from the laser beam by wrapping it with a foil tape has proved effective (commercial devices are available) (Fig. 47-5).58 Tubes made of materials such as metal and silicone and those with special double cuffs also reduce the risk of airway fires and injury during laser airway surgery.51,58
3 Subglottic Suctioning Evac Endotracheal Tubes
Hospitalized patients who require mechanical ventilation are susceptible to the development of aspiration pneumonia. VAP is known to increase hospital length of stay, health care costs, and mortality.59 Organisms that grow in pooled subglottic secretions above the inflated cuff of the ETT, but beneath the glottis, have previously been unmeasurable with any reliability and are now demonstrated to be a major impetus for VAP. Several nursing care measures may be taken to reduce the incidence of VAP caused by this route, including improved oral care, patient positioning by elevating the head of the bed past 30 degrees, frequent suctioning, and ensuring postpyloric tube feedings, but none of these measures completely stops the production.60
The presence of these pooled collections has led to the development of specific ETTs that possess a dedicated suction system capable of emptying this area of debris. Drainage of subglottic secretions has been shown to prevent VAP.61–64 The currently available subglottic drainage ETTs have a suction lumen that opens on the external (posterolateral) surface of the ETT immediately above the cuff (Fig. 47-6). The lumen is attached to constant or intermittent suction for active drainage of the space. Although these ETTs are beneficial, their efficacy is not 100%, and therefore all of the aforementioned nursing care actions remain vital to good hygiene and prevention of VAP. The subglottic drainage tubes have been further developed to include variations in cuff construction (materials, shapes, volumes, locations) that help to prevent aspiration of the subglottic debris.
Subglottic secretions are not the only recognized cause for VAP. Biofilm is an accumulation of debris adhered to the internal circumference of the ETT that is composed of tissue, secretions, mucus, and undetermined bacteria load. Biofilm can be aspirated, leading to a nidus for infection or causing an area of obstruction to airflow. Biofilm removal and reduction by hygiene care are currently better researched than prevention. However, there is a growing interest in the reduction of biofilm through construction of ETTs impregnated with antimicrobial agents.65–67 The ability of such developments to affect the incidence of VAP has not yet been proved.
4 Double-Lumen Endotracheal Tubes
The uses of a double-lumen endotracheal tube (DLT) (Fig. 47-7) can be separated into relative and absolute indications. The absolute indications are isolation, to avoid soilage or contamination of the contralateral lung tissue when dealing with infections or frank hemoptysis from a unilateral location, bronchoalveolar lavage, and one-lung ventilation (OLV). The most common reason for placement is OLV for surgical exposure, but OLV can also be important in cases of bronchopleural or bronchocutaneous fistula, unilateral pulmonary hemorrhage, giant unilateral bulla or cyst, and severe unilateral ventilation-perfusion mismatch. The relative indications all deal with surgical exposure. Complementing the DLT as another option for lung isolation, particularly if a DLT cannot be placed, are bronchial blocking devices. However, the DLT has an advantage because of the ability to pass suctioning catheters or fiberoptic devices into the area on collapse without drastically jeopardizing OLV or contaminating the contralateral side.
Relative contraindications to the placement of a DLT are fairly minimal. They include patient refusal (likely due to risk of trauma secondary to the large size), a known difficult airway, and the speed with which an isolated airway must be established. In patients with difficult airways, specially designed airway exchange catheters (Cook Medical, Bloomington, IN) (Fig. 47-8) can be used after placement of a conventional ETT to facilitate DLT placement. Additionally, some makers of video laryngoscopic technologies have developed specific DLT devices. The time required for placement is usually the biggest detractor to their use. Situations such as frank hemoptysis may be better served by a rapid-sequence induction and placement of a contralateral, main stem, single-lumen ETT for stabilization.
5 Supraglottic Airways
Supraglottic devices are continually coming onto the market. Largely designed for shorter surgical procedures to deliver general anesthesia, their use seems to be growing past their original intent. Supraglottic airways (Fig. 47-9) such as the LMA, the esophageal-tracheal Combitube (ETC, Tyco Healthcare, Mansfield, MA), the King LT (King Systems, Noblesville, IN), and other variants have provided valuable means of establishing an (unsecured) airway in an emergency. Equally important is their ability to function as a conduit for endotracheal intubation. For example, the ILMA is a blind passage device that is designed to place an ETT through the LMA. Recently, use of the LMA as a conduit for fiberoptic bronchoscopy and subsequent ETT placement has also been demonstrated in emergency situations.68 Although these devices do not provide classically definitive airways, their utility is unsurpassed in helping to manage the difficult airway.
IV Proper Safeguarding of the Airway
A Airway Evaluation: Predicting the Difficult Airway
However, a difficult airway should be assumed when one approaches the patient outside the controlled setting of the operating room. An airway physical examination is paramount to predicting a successful attempt. Criteria such as dental status, mouth opening, thyromental distance, cervical range of motion, Mallampati score, and neck circumference are all standard examination points. However, the emergent intubation presents a host of new, potentially detrimental issues not seen in operating room intubations. For example, hemodynamics may not allow for the controlled process typically seen in the operating room. Trauma patients may have actively unstable facial fractures or cervical vertebral injuries that merit inline stabilization and modified techniques for tube placement. Neurosurgical patients may have external fixators to stabilize injuries or intracranial monitoring devices that make it difficult to position the head. The type of bed a patient is in can also create access issues with intubation. A bariatric bed with an inflatable mattress can be very difficult to properly ramp, thereby making positioning suboptimal. Last, but not least, is the patient with failed extubation who must be reintubated. Issues with anxiety, hypoxemia, decreased functional residual and closing capacities, copious secretions, residual airway edema, and subglottic stenosis all make a repeat attempt more difficult than the first pass.28
B Identifying Proper Position of the Endotracheal Tube
1 Detection of Esophageal Intubation
Once the clinician has deemed intubation necessary and has performed the intervention, confirmation of proper ET placement must be provided expeditiously. An incorrectly positioned ETT can produce adverse effects, especially in an already apneic patient. Unrecognized esophageal intubation can have disastrous consequences with a reported incidence as high as 8% in critical patients.69 Therefore, a brief discussion of verification of ETT placement is warranted.
Once intubation has been accomplished, confirmation of proper placement of the ETT in the trachea needs to be achieved, ideally by the detection of end-tidal carbon dioxide (EtCO2) in expired gases using capnography or other capnometric (colorimetric) methods.70 The detection of EtCO2 is not fail-safe and does not guarantee that the ETT is positioned within the tracheal lumen (e.g., the tip may lie above the vocal cords). After three to five breaths, the absence of EtCO2 suggests a nontracheal placement. Blockage or soilage of the EtCO2 detection device can hamper efforts. EtCO2 detection should be complemented with indirect maneuvers such as auscultation, ETT misting or fogging, bag compliance, chest wall excursions, lack of phonation, and improved oxygen saturation. The dependence of any EtCO2 detection device on adequate cardiac output has spurred utilization of an esophageal detector device (Fig. 47-10). It is essentially an air-filled bulb placed on the end of an in situ ETT. After a vacuum has been created by squeezing the bulb, immediate re-expansion should occur when the bulb is placed on an ETT in the trachea (any column of air). If the ETT is esophageal, the suction created by the bulb will draw the pliable esophageal tissue into the distal lumen of the ETT, preventing full expansion of the bulb on top of the ETT (column of soft tissue).71–73
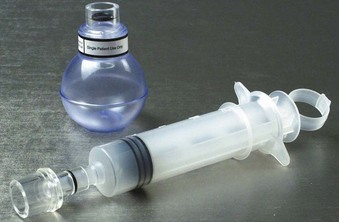
Figure 47-10 Esophageal bulb detector and a homemade syringe device.
(Courtesy Wolfe-Tory Medical, Inc.)
If intubation is to occur in a patient with cardiac arrest, capnometry may be fallible given the amount of down time and the lack of any life-sustaining cardiac output. If cardiopulmonary resuscitation (CPR) is adequate, this technicality is likely to be moot. However, esophageal bulb detectors can be helpful in this situation, although false-positives and false-negatives do occur and confound ETT verification. Still other methods that are independent of carbon dioxide and cardiac output exist, such as a tracheal whistle to verify correct placement (Box 47-1), but not one is without limitations.72–77 Ideally, two methods are to be considered fail-safe: direct or indirect visualization of the ETT traversing the vocal cords and fiberoptic verification via the ETT lumen. The limitation of direct laryngoscopy is the operator’s line of sight to the glottic opening. The key is to identify the ETT positioned in the glottis, not simply to see it go in. An indirect method such as video laryngoscopy does improve the validity of ETT passage. Fiberoptic verification is hampered by equipment availability at the bedside, any airway or ETT soilage, time constraints, and operator skill. Chest rise and condensation from expired gas found in the ETT have also been used, but these signs may occur in esophageal intubations as well.77 Therefore, the gold standard used today is the presence of EtCO2 in expired gases as demonstrated by capnography or other colorimetric techniques.70
Box 47-1
Methods Used to Verify Endotracheal Tube Placement
Sustainable, exhaled CO2 by capnography or colorimetric methods
Fiberoptic visualization of carina
Videolaryngoscopic visualization of translaryngeal endotracheal tube position
Direct laryngoscopic visualization of translaryngeal position
Positive response with esophageal bulb detector device or syringe method
Auscultation of breath sounds in bilateral lung fields and absence of same in epigastric area
Visualization of chest wall movement with spontaneous patient efforts
Reservoir bag synchrony with spontaneous patient efforts
Palpable ballottement of cuff in suprasternal notch
Condensation in endotracheal tube
Lack of phonation in the nonparalyzed, semiconscious patient
Bougie passage to distinguish between tracheobronchial tree and esophagus
Capnography yields quantifiable measurements of inspired and expired gases in addition to a waveform generated with each tidal volume. Although not without limitations (e.g., lack of portability, need for a power source), capnography reliably identifies initial proper placement of the ETT (nonesophageal) and provides a continuous verification of ETT security. If esophageal intubation has occurred, a gradual reduction in height of the capnograph waveforms is observed with successive breaths. False-positive results during esophageal intubations may occur in situations of ingested CO2-containing or -liberating substances (e.g., carbonated beverages) before intubation, bag-mask ventilation with inflation of expired air into the stomach, or intubation of the supralaryngeal hypopharynx.78 Inappropriate extubations may occur due to misinterpretation of a false-negative situation because an EtCO2 waveform is lacking despite proper placement. This error may occur with unrecognized circuit disconnections, an obstructed or kinked ETT, a disconnected or contaminated gas sampling line (water, secretions, entrainment of room air), equipment failure, severe bronchospasm, or inadequate cardiac output. Unquestionably, capnography is dependent on pulmonary blood flow. In the absence of perfusion (e.g., cardiac arrest), the utility of capnography can be limited; however, even in very low-flow states (e.g., CPR, separation from cardiopulmonary bypass), it has been shown to provide effective detection. Ornato and colleagues used an animal model to evaluate the relationship between cardiac output (CO) and EtCO2. Through manipulation of CO, with inotropes or controlled hemorrhage, a logarithmic relationship between CO and EtCO2 was demonstrated.50 This finding shows that capnography is useful in cardiac resuscitation to assist with evaluation of low-flow states and adequacy of perfusion.
To alleviate the logistic concerns with capnography in emergency situations—mainly the lack of portability and the need for a power source—a portable and reliable means of detecting EtCO2 was developed. Colorimetric EtCO2 uses a detector impregnated with metacresol purple (Fig. 47-11). This indicator is pH sensitive and changes color, from purple to yellow, in the presence of CO2. The devices are disposable, attach between the ETT and the circuit or bag, and provide a rapid and reliable indication of CO2 concentration on a graded scale: A (purple) corresponds to an EtCO2 level of 0.5%, B (tan) to a level of 0.5% to 2%, and C (yellow) to a level greater than 2%.70,77 Limitations of this method are that it is ineffective with exposure to humidified gases, vomitus, or secretions and in cases of prolonged cardiac arrest or low-perfusion states. False-positive results can occur as well, just as in capnography. Delays in recognition of esophageal intubation with colorimetric capnometry have been reported far more often than with capnography, particularly in patients with prolonged bag-mask ventilation or ingestion of EtCO2-containing substances before intubation. Therefore, capnography simply is the best method for detection of esophageal intubation.79
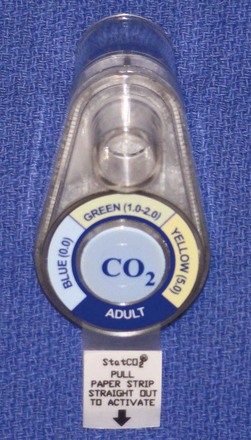
Figure 47-11 Example of a colorimetric capnometric device.
(Courtesy Mercury Medical, Clearwater, FL.)
As mentioned previously, the esophageal bulb detector capitalizes on the physical characteristics of the esophagus, which, unlike the trachea, collapses when negative pressure is applied. Commercial devices exist, but a homemade version can be improvised using a syringe that attaches to the end of the ETT. When the plunger is withdrawn, resistance is appreciated if an esophageal intubation has occurred, because the walls of the esophagus collapse around the ETT. Unencumbered aspiration of the plunger occurs with proper tracheal placement.71 Bulb detector devices are reported to be reliable and effective, with one study demonstrating a sensitivity of 100% and a specificity of 99%.72 However, other studies have reported limitations and false-negative results in patients with copious or aspirated secretions, gastric distention, vomitus in the airway, morbid obesity, or reduced functional residual capacity.71,80–83
2 Confirmation of Appropriate Depth of Insertion
After correct tracheal placement of the ETT has been verified, it is imperative to identify the correct depth of the ETT to ensure adequate ventilation of each lung.49 Before addressing the various methods that assist in confirmation of appropriate ETT depth, a brief discussion of what is considered to be the correct depth is warranted. Malpositioning of ETTs occurs frequently, with unrecognized right main stem intubation occurring in approximately 4% of chest radiographs.49,69,84 The generally accepted depth of insertion of the ETT is between 2 and 7 cm above the carina, optimally between 4 and 7 cm above the carina with the head and neck in a neutral position.49,77,85–87 It is important to realize this, because flexion-extension movements of the neck can displace the ETT upward or downward with resultant extubation or main stem intubation, respectively. Typically the right main stem bronchus is entered, given its straighter trajectory in relation to the trachea. If endobronchial intubation remains undetected, an inadvertent hyperinflation of the ipsilateral lung can occur with subsequent pneumothorax and concomitant atelectasis of the hypoventilated contralateral lung. Indeed, it has been reported that up to 15% of chest radiographs reveal malpositioned ETTs in intubated patients.84,88,89 This is more frequently seen after difficult airway management.
In the operating room, chest radiographs are not used to confirm proper position; rather, indirect clinical assessment methods are used (i.e., auscultation of bilateral breath sounds, visualization of equal chest expansion, and direct visualization of the ETT tip placed just below the vocal cords). The ETT is also manufactured with distance measurements to aid with the depth of insertion. In orally intubated patients, a depth of 23 cm at the teeth or corner of the mouth has been advocated for men and 21 to 22 cm for women.90,91 In nasotracheal intubations, a depth of 26 cm at the nares in women and 28 cm in men should be sufficient for proper tracheal position.92 Other methods used include direct visualization of the ETT tip in reference to the carina with a fiberoptic scope or catheter,69 transtracheal illumination, and ballottement of the ETT cuff in the suprasternal notch.93–96