Chapter 96 Endoscopic Third Ventriculostomy
Neuroendoscopy and minimally invasive techniques have played an increasing role in contemporary neurosurgery. Endoscopic third ventriculostomy, specifically, is a procedure that has experienced a resurgence in popularity in the last 30 years and has become the most commonly performed neuroendoscopic procedure.1 However, endoscopy was present and utilized since the turn of the 20th century, when surgical interventions for hydrocephalus were first being developed.
Historical Background
The first neuroendoscopic procedure was performed in 1910 by Vincent Darwin L’Espinasse, a Chicago urologist, who used a cystoscope to fulgurate the choroid plexus in two infants with hydrocephalus.2 One of the patients died; however, the other lived for 5 years. Walter Dandy performed several procedures for hydrocephalus. In 1918, he performed the first open ventriculostomy by fenestrating the lamina terminalis through a transfrontal approach.3 In 1922, he unsuccessfully attempted a choroid plexectomy using the endoscope and at that time coined the terms “ventriculoscope” and “ventriculoscopy.”4 Fay and Grant were the first to photograph the inside of the ventricular system in 1923. Interestingly, the exposure time for their images ranged from 30 to 90 seconds, accentuating the extremely poor lighting and visualization of neuroendoscopy at that time.5
This first successful endoscopic third ventriculostomy (ETV) was performed in 1923 by William J. Mixter in a 9-month-old hydrocephalic patient. Utilizing a urethroscope, he was able to pass through the foramen of Monro, visualize the third ventricle and cerebral aqueduct, and make a hole in the floor of the third ventricle connecting it to the interpeduncular cistern. He subsequently enlarged the hole with side-to-side mechanical motion up to a diameter of 4 mm.6 The next successful ETV did not appear in the literature until 1935, when Scharff reported his initial results using a novel endoscope. Scharff described several modifications including an irrigation system that kept the ventricles open, a mobile cautery tip, and a moveable operating tip that could perforate the floor of the third ventricle.7 Around the same time, resection of the choroid plexus was still a popular treatment for hydrocephalus. Tracy Putnam modified a cystoscope for use in the ventricular system, specifically designing it to cauterize choroid plexus in order to treat children with hydrocephalus.8 In one of his series of 42 patients, which was the largest at that time, 17 patients (40%) responded favorably while 10 patients (25%) died perioperatively.9 In 1947, McNickle described a modified technique of performing a percutaneous third ventriculostomy utilizing a 19-gauge needle to puncture the floor of the third ventricle. Although he initially used an endoscope for the procedure, he later abandoned its use in favor of using x-rays and tactile feedback for localization.10
Despite the advances made by a few pioneering surgeons, neuroendoscopic procedures were not widely adopted during the first half of the 20th century.11–13 Technology was a significant limiting factor as lighting and visualization were very poor and the setup of the endoscopic lenses was complicated and cumbersome.11,12 In 1952, Nulsen and Spitz first reported creation of a shunt diverting cerebrospinal fluid (CSF) from the ventricular system to the jugular vein to successfully treat communicating hydrocephalus.14 This began a monumental shift in the hydrocephalus treatment paradigm toward shunt diversion. Although a select few surgeons would continue performing neuroendoscopic procedures to treat hydrocephalus, the majority of neurosurgeons adopted CSF shunt diversion as standard practice due to the low complexity and safety of the procedure.12
During this hiatus in the clinical use of neuroendoscopy, critical advances in technology would eventually lead to revisiting the role of neuroendoscopy in neurosurgery. First-generation endoscopes utilized conventional lenses, which had a fixed refractive index. This required the lenses of the endoscope to be carefully placed in series in order to relay an image. In 1966, Hopkins and Storz revolutionized lens technology by developing the SELFOC lens. These lenses used gradient-index glass, which allowed adjustment based on the lens radius.15 Compared to conventional lenses, a wider field of vision could be viewed while preserving the illumination.12,15,16 In 1969, Smith and Boyle developed the charge-coupled device, which allowed optical data to be converted into electrical current.12 This precluded the need for large relay stations and allowed for the development of smaller endoscopes. Perhaps most important was the development and improvement of fiberoptic cables that occurred between the 1950s to 1970s. Using fiberoptic cable technology, light could be separated from the rest of the endoscope and emitted from the tip without emitting significant amounts of heat or losing luminescence.12,15
With improved technology, use of the endoscope was revisited for hydrocephalus treatment. In 1978, Vries reported a series of five patients who he had treated with ETV utilizing a fiberoptic endoscope.17 Although all of his patients eventually required a shunt diversion procedure, the patients did well postoperatively demonstrating that ETV was technically feasible with current technology. In 1990, Jones et al. reported 50% successful outcome from ETV in 24 patients with noncommunicating hydrocephalus with 8% morbidity and no mortality.18 In 1994, the same group reported an even better success rate (61%) in a series of 103 patients.19 Given the technological advances in endoscopic illumination and optics, the demonstrated safety of the procedure with second-generation endoscopes, and the clinical efficacy reported in the literature, ETV was widely adopted in the 1990’s by the neurosurgical community to treat hydrocephalus.1,12
Patient Selection
Selection of the appropriate patient for ETV requires an understanding of CSF physiology, detailed study of radiographic anatomy, an appreciation for the location of CSF obstruction, and knowledge of results for various age groups and etiologies of hydrocephalus.3,20 The CSF circulation is analogous to a hydraulic system. CSF flows sequentially through each chamber starting in the choroid plexus until being reabsorbed in the arachnoid villi. Pathologic obstruction can occur at any point in the circuit or at the end. ETV treats hydrocephalus by creating an internal shunt from the third ventricle to the interpeduncular cistern in the cortical subarachnoid space (Fig. 96-1). If the pathologic obstruction lies between the third ventricle and the cortical subarachnoid space, then ETV will likely be an effective treatment. If the pathologic obstruction lies downstream from the cortical subarachnoid space, then ETV will be of no benefit.20
Pathologic Considerations
The etiology of the pathologic obstruction should be determined when considering the most appropriate treatment for hydrocephalus. The most responsive etiologies of hydrocephalus to ETV are tumors of the tectal region and congenital aqueductal stenosis.21,22 Tectal gliomas or pineal region tumors can compress the cerebral aqueduct, obstructing CSF flow from the third ventricle to the fourth ventricle. Likewise, congenital aqueductal stenosis also results in impaired flow from the third ventricle to the fourth ventricle. It can present in infants, children, adolescents, or adults.23,24 The later it presents, the better chance that ETV will successfully treat the hydrocephalus. Care must be taken not to confuse congenital aqueductal stenosis with narrowing of the cerebral aqueduct that occurs secondary to inward displacement of the brainstem. This scenario can be present in infants or young children with increased pressure of the superior sagittal sinus leading to decreased terminal absorption of CSF. When the sutures are open, this can lead to global increases in CSF volume, including in the ventricular system and the basal cisterns. An increase in the CSF volume in the prepontine and interpeduncular cisterns can produce compression of the brainstem leading to secondary compression of the cerebral aqueduct, mimicking aqueductal stenosis.20 In these cases, the site of obstruction lies distal to the interpeduncular cistern and placement of a ventricular shunt, not ETV, would be the indicated intervention.20,25
Nontectal/pineal region tumors producing hydrocephalus can also benefit from ETV if properly selected.26–28 Prior to surgical intervention, the exact point of CSF obstruction needs to be determined. If the point of obstruction occurs along the course of CSF flow between the third ventricle and the interpeduncular cistern, then ETV may be useful. If the point of obstruction lies outside this course, then shunting should be considered. However, tumor resection may be the only treatment needed to cure the hydrocephalus. For example, in patients with noncommunicating hydrocephalus secondary to mass effect from fourth ventricular tumors, we prefer surgical resection of the tumor to correct hydrocephalus. An intraventricular catheter can be placed for intracranial pressure monitoring and temporary CSF drainage as needed prior to the tumor resection. Others advocate performing an ETV prior to tumor resection.29,30 Although this option bypasses the site of CSF obstruction, these patients need to be carefully selected due to the risk of upward herniation of the superior cerebellar vermis which can result in midbrain compression.20
Congenital abnormalities can be associated with hydrocephalus. Approximately 80% to 90% of patients with myelomeningocele have hydrocephalus requiring intervention.31,32 The localization of CSF obstruction in these patients is often more complex than in patients with obstruction secondary to a tumor. For example, Chiari II patients can have up to four pathologic sites of obstruction.33 If there are multiple sites of obstruction, ETV may not effectively bypass the entire site and hence be unsuccessful. However, not all Chiari II patients have the same patterns of obstruction. For example, ETV has been shown to be successful in a small cohort of adult Chiari II patients, implying a more simplified pattern of obstruction between the third ventricle and the interpeduncular cistern.34 Thus, the role of ETV in cases of congenital malformations associated with hydrocephalus is not clear and consideration of intervention must be done on a case-by-case basis.
Vascular pathologies such as subarachnoid hemorrhage (SAH) and intraventricular hemorrhage (IVH) can produce hydrocephalus. The mechanism of hydrocephalus in the acute setting is usually decreased CSF absorption from obstruction in the arachnoid granulations and a mechanical outflow obstruction from clot within the ventricular system. However, over time as the blood resolves, obstruction at the level of arachnoid granulations and within the ventricles may not be the long-term cause of hydrocephalus. Once the inflammatory process progresses, the point of obstruction usually becomes localized to the basal cisterns, at the interface of the spinal subarachnoid/cranial subarachnoid spaces.20 In the setting of SAH, external ventricular drainage is commonly performed to temporize the patient. In some SAH patients, there is minimal residual obstruction and the external ventricular drainage can be weaned off without further intervention. Other patients with SAH may require permanent CSF diversion. In patients without prior surgical intervention, ETV can be considered if there is no anatomic contraindication. In patients with a prior surgical intervention, ETV may be of more limited use.35 For example, fenestration of the lamina terminalis during open surgery for a ruptured aneurysm is direct, safe, and potentially avoids the need for a repeat operation to treat hydrocephalus.36 Patients with IVH can similarly show a clinically significant, time-dependent change in the localization of CSF obstruction. In the series by Siomin et al., a higher rate of ETV success was associated with previous history of ventriculoperitoneal shunt placement post-hemorrhage.37
Post-infectious hydrocephalus can also require CSF diversion. Obstruction resulting from infection can be localized to the basal cisterns or to the arachnoid granulations.20 If the obstruction is in the basal cisterns then ETV can be considered. If the obstruction is in the arachnoid granulations, then ETV would not be beneficial. However, determination of the exact site of obstruction in these patients can be challenging and is not always possible. Another consideration in post-infectious patients is that there can be significant meningeal thickening of the leptomeninges and third ventricular floor. Thus, creation of a hole in the floor of the third ventricle can be more challenging and have a higher risk of closure.37 History of infection has been shown to be a negative predictor of ETV success.22,38 However, in properly selected patients ETV can be successful in treating post-infectious hydrocephalus.20,37
The pathophysiology of idiopathic normal-pressure hydrocephalus (iNPH) has been the subject of much debate. Although some patients exhibit asymmetry between the third and fourth ventricles, suggestive of an associated late-onset idiopathic aqueductal stenosis, others exhibit global enlargement of the ventricular system. Indeed, the pathophysiology of iNPH is likely unrelated to a mechanical obstruction, but rather an abnormality of ventricular compliance and CSF flow.37,39 It is known that certain patients with iNPH respond favorably to ETV. Gangemi and colleagues reported the largest series to date of iNPH patients treated with ETV. Out of 110 patients, improvement was seen in 69.1% of patients, no change in 21.8%, and continued deterioration was seen in 9.1%.39 This compares favorably to other reported outcomes from shunting, although no randomized comparison has yet been performed.39,40 ETV may be a more physiologic solution and the problems associated with overdrainage have not been seen, which make it a promising alternative to shunt diversion in select iNPH patients.39
Other Considerations
There are factors other than pathology that have been shown to impact success of ETV for hydrocephalus. Age is one of the most important factors in predicting success of ETV in the pediatric population. Kulkarni and co-workers performed a multicenter, retrospective analysis on 618 pediatric patients who had undergone ETV.22 A training set of 455 patients was used to identify predictors of ETV success and the remaining 163 patients were used to internally validate those predictors. In their analysis, age was the most significant predictor of ETV success. Younger patients (<1 year old) had a higher chance of failure compared to older patients, and infants (<1 month old) in particular had the highest likelihood of failure.22 This confirmed previous reports and added weight to those that discourage ETV in infant patients.41,42 In adult patients, there does not appear to be an association between age and risk of failure.39
The presence of a previous shunt has different implications when considering treatment with ETV. In the prior analysis of 618 pediatric patients by Kulkarni et al., presence of a shunt was shown to increase risk of ETV failure, although to a lower degree than certain hydrocephalus etiologies (e.g., postinfectious and very young patient age).22 However, the effect of treatment with shunting prior to ETV likely is different depending on the pathology. In the series reported by Siomin and colleagues, treatment with shunting prior to ETV was actually a positive predictor of ETV success. This difference was especially pronounced in the subset of patients with a history of premature birth.43
In chronically shunted patients with associated symptoms, conversion to an ETV from a shunt can be done by following an algorithm20 (Fig. 96-2). The shunt is first removed and replaced with an external ventricular drain. The patient is placed in the intensive care unit for monitoring and the external ventricular drain subsequently clamped. If the ventricles enlarge and the intracranial pressure (ICP) rises, ETV is attempted. If there is subsequently no ventricular enlargement after ETV, patients are either observed for 48 hours (no change in ICP) or shunted (significant rise in ICP).20
Contraindications to Endoscopic Third Ventriculostomy
There are certain situations in which an ETV is contraindicated. Anatomically, the lateral and third ventricles can be too small to safely pass an endoscope. This occurs in situations of “normal volume hydrocephalus,” when little to no change is observed in ventricular size when ICP rises such as in pseudotumor cerebri. In other cases, one of the anatomic structures can block the path to the floor of the third ventricle. For example, the massa intermedia in Chiari II patients can be so large as to prevent safe passage of the endoscope. Pathology, such as a tumor or other mass lesion can also block the trajectory to the floor of the third ventricle. Physiologically, the CSF flow should be examined on preoperative imaging. If the obstruction is above the level of the third ventricle, distal to the interpeduncular cistern, or if no clear obstruction can be identified then the utility of ETV is limited.20
Surgical Equipment
There are three basic types of endoscopes: rigid, flexible, and semiflexible (Fig. 96-3). Rigid scopes offer the best optics, a wide-angled view, allow magnification without loss of resolution, and can have instruments passed through them. Flexible scopes allow a wide degree of bending, which allows the operator to look around corners. However, their resolution diminishes with magnification and they may not allow many instruments to be passed through them. Semiflexible scopes have better resolution and less flexibility compared to flexible scopes, but do not have the resolution or wide-angled view seen with rigid scopes.44 We prefer rigid scopes with a 0- or 12-degree angle of view for ETV.
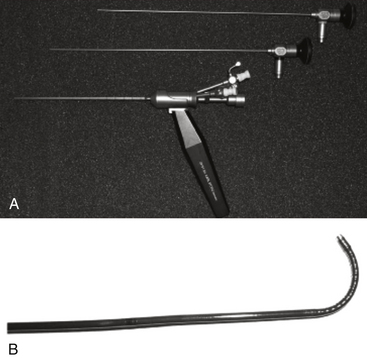
FIGURE 96-3 An example of a 0- and 30-degree rigid endoscope with sheath (A) and a flexible endoscope (B).
(From Sciubba DM, Noggle JC, Jallo GI: Equipment for neuroendoscopic procedures. In: Jallo GI, Conway JE, Bognar L, eds., eds. Neuroendoscopy of the Central Nervous System. San Diego: Plural; 2008:13-22.)
The light source provides bright, cold illumination and typically comes from a halogen, xenon, or metal-halide lamp. In particular, the color of the picture seen under illumination with xenon most closely resembles the color in natural sunlight.15,44 The video unit is composed of a chip video camera, a camera control unit, video monitor, and a video documentation system. The video documentation system can include still-shot capture, video recording, a CD/DVD burner, and a printer (Fig. 96-4).
Operative Technique
The patient is first placed supine with the head in the neutral position on a doughnut pillow or in three-pin fixation when neuronavigation is used. The head of bed is placed at 30 degrees to prevent air entry and excessive loss of CSF. The monitor and neuroendoscopy tower should be placed at the foot of the bed so that the surgeon may directly visualize the monitor while operating (Fig. 96-5). Antibiotics are given within 1 hour prior to skin incision to reduce risk of infection. Site of entry will vary slightly depending on age, and side of surgery is determined preoperatively depending on the anatomy and pathology of the patient. If no contraindication is present, the nondominant side is selected.45 A useful reference point superficially is the intersection between the midpupillary line and the coronal suture. A burr hole is made just anterior to the coronal suture. The dura is opened in a cruciate fashion and the dural leaflets and pia are coagulated with bipolar cautery. If a large cortical vein is present, it is important to make minor adjustments in the surgical tract in order to avoid unnecessary damage to the vein.
A peel-away catheter (12.5 or 14 French) is passed into the lateral ventricle by aiming medially toward the ipsilateral medial canthus and posteriorly toward the contralateral external acoustic meatus. Once CSF return is appreciated and the peel-away catheter is frankly in the lateral ventricle, the stylet is removed and the sheath is secured by peeling away its two sides. The sides are secured to the drapes with staples to avoid inadvertent deepening of the sheath. The endoscope is inserted through the sheath and the ventricle is inspected. The main landmarks including choroid plexus, fornix, posterior commissure, veins, and the foramen of Monro are identified45,46 (Fig. 96-6A). In adults, the foramen of Monro sits 5 to 6 cm from the dura mater, however, in children this distance is less. Continuous irrigation is usually unnecessary during inspection. If bleeding is encountered, gentle irrigation clears the operative view. Most small hemorrhages tend to stop spontaneously after irrigating for several minutes. A bipolar probe may also be used if irrigation is unsuccessful. Once the foramen of Monro is clearly visualized, the endoscope is passed through until the third ventricle comes into view. On average, the floor of the third ventricle lies 7 cm deep to the dura, however, this can vary depending on the age of the patient and the extent of the hydrocephalus.45
Perforation of the floor of the third ventricle is the most dangerous step of an ETV. Inspection of the anatomic landmarks must be done to identify the safe zone in the floor of the third ventricle. These landmarks include the mammillary bodies, the tuber cinereum, the infundibular recess, and optic chiasm45,46 (Fig. 96-6B). The target for the ventriculostomy lies between the mammillary bodies and the infundibular recess. Care must be taken not to injure the basilar artery, which lies just anterior and inferior to the mammillary bodies and can sometimes be visualized through the diaphanous floor of the third ventricle (Fig. 96-7). We prefer using the Bugbee wire to bluntly puncture the floor of the third ventricle. A 3-French Fogarty balloon catheter is passed into the opening in the floor of the third ventricle and the balloon is inflated with 0.2 ml of fluid. The inflating balloon widens the aperture and the process is repeated until the opening is sufficiently wide. It is not recommended to inflate the balloon under the floor and pull back through the stoma; rather, the balloon is inflated while its center is within the newly created fenestration. Doppler devices are also available to help locate the basilar artery prior to fenestration. While the technique described is the one we feel is the safest, several other techniques are used. Perforation of the floor of the third ventricle can be done using a variety of instruments including electrocautery, ultrasonic microprobes, or laser devices. Likewise, enlarging the opening can be done using hooks, scissors, or by using blunt forceps.45,47 The technique originally described by Mixter, which used the tip of the endoscope to bluntly perforate the floor of the third ventricle and widened it with a side-to-side motion, is still used by some surgeons today.6,47 Each of these instruments has their advantages and limitations. Regardless of the technique used, care should be taken to avoid the structures within the interpeduncular cistern, which underlies the floor of the third ventricle. These include the basilar artery and its branches, and both oculomotor nerves.
Postoperative Monitoring
The patient is observed for 24 hours in the intensive care unit or monitored setting. If an external ventricular drain was left due to bleeding, CSF is aggressively drained during this time period in order to clear the fluid and minimize the risk of intraventricular clot formation. If the external ventricular drain was left in because the ETV was to replace a previous shunt, the drain should remain clamped and ICP continuously monitored. In these patients, the ventricular drain needs to be weaned slowly as it has been noted to take up to 10 days for patients to tolerate the new absorption pathway.45 Once the drain is removed, the patient may be sent home if ambulating and tolerating a diet. If the patient is having headaches, nausea, or vomiting, it is prudent to keep the patient in the hospital until symptoms have largely resolved. In infants, the fontanelle should be soft and sunken when in an upright position.
A postoperative CT scan is helpful prior to patient discharge. Although the initial change in ventricular size may be unimpressive, the subarachnoid space often appears less effaced. MRI is usually performed within 2 months of the procedure, which can show smaller ventricular size, decreased transependymal edema, and a flow void in the floor of the third ventricle. A cine-MRI with CISS sequence may be especially helpful in demonstrating flow from the third ventricle to the interpeduncular cistern.
Complications
The morbidity reported for ETV in modern series, in which contemporary endoscopic equipment and techniques were used, ranges from 6% to 21%.48 Additionally, the procedure-abort rate varied from 0.4% to 26%.48 Mortality rates are low following ETV and are usually related to fatal subarachnoid hemorrhage or late failure of the third ventriculostomy.
Hypothalamic injury can occur intraoperatively with a variety of effects. It is first important to remember that the floor of the third ventricle is in fact part of the hypothalamus. Serious bradycardia and asystole have been noted at the time of fenestration and upon reversal of the neuromuscular block. This may be caused by a mechanism involving distortion of the posterior hypothalamus. Additionally, increased appetite, loss of thirst, diabetes insipidus, and amenorrhea have also been reported postoperatively secondary to presumed hypothalamic injury.49
Vascular injury can also occur intraoperatively and ranges from clinically insignificant to devastating. In a series of 55 patients, Teo and coworkers reported a 10.9% incidence of “clinically insignificant” hemorrhage.50 In most instances, minor intraventricular bleeding can be controlled with irrigation although it can result in aborting the procedure. Although extraordinarily rare, major vascular injury resulting in significant morbidity and mortality has been reported. This includes trauma to the basilar artery or one of its branches, subarachnoid, intraventricular, or intracerebral hemorrhage, pseudoaneurysm formation at the basilar tip, and arterial or venous infarcts.51–53
CSF leak after ETV is a rare, but known postoperative complication. In order to prevent CSF leak, it is important to perform a multilayered closure and to minimize the size of the dural opening used. However, CSF leak can be a sign of increased ICP, and ETV failure must not be ruled out.49 Although fever in the first 24 to 48 hours postoperatively is common, ventriculitis is rare. The presence of blood in the ventricular system is the most common cause of perioperative fever.49 However, any previous shunt hardware can harbor bacteria and should be completely removed in order to prevent late ventriculitis.54 Other rare complications include overdrainage resulting in subdural hematoma or hygroma, symptomatic pneumoencephalus, and psychiatric or memory disorders caused by forniceal or mammillary body injury.49
Abbott R. History of neuroendoscopy. Neurosurg Clin North Am. 2004;15:1-7.
Bhatia R., Tahir M., Chandler C.L. The management of hydrocephalus in children with posterior fossa tumours: the role of pre-resectional endoscopic third ventriculostomy. Pediatr Neurosurg. 2009;45:186-191.
Conway J.E., Baird C.J., Li K.W., et al. Ventricular anatomy. In: Jallo G.I., Conway J.E., Bognar L. Neuroendoscopy of the Central Nervous System. San Diego: Plural; 2008:23-36.
Dandy W. Extirpation of the choroid plexus of the lateral ventricles in communicating hydrocephalus. Ann Surg. 1918;68:569.
Dandy W.E. An operative procedure for hydrocephalus. Bull Johns Hopkins Hosp. 1922;33:189-190.
Drake J.M., Iantosca M.R.. Cerebrospinal fluid shunting and management of pediatric hydrocephalus, Schmidek H.H., Roberts D.W., editors. Schmidek and Sweet’s Operative Techniques in Neurosurgery, 5th ed, vol 1. Philadelphia: Elsevier, 2005;487-508.
Fay T., Grant F.C. Ventriculoscopy and intraventricular photography in internal hydrocephalus. JAMA. 1923;80:461-463.
Gangemi M., Maiuri F., Naddeo M., et al. Endoscopic third ventriculostomy in idiopathic normal pressure hydrocephalus: an Italian multicenter study. Neurosurgery. 2008;63:62-69.
Grant J.A. Victor Darwin Lespinasse: a biographical sketch. Neurosurgery. 1996;39:1232-1233.
Iantosca M., Hader W., Drake J. Results of endoscopic third ventriculostomy. Neurosurg Clin North Am. 2004;15:67.
Kulkarni A.V., Drake J.M., Mallucci C.L., et al. Endoscopic third ventriculostomy in the treatment of childhood hydrocephalus. J Pediatr. 2009;155:254-259. e251
Li K.W., Nelson C., Suk I., Jallo G.I. Neuroendoscopy: past, present, and future. Neurosurgical Focus. 2005;19:E1.
Mixter W.J. Ventriculoscopy and puncture of the floor of the third ventricle. Boston Medical and Surgical Journal. 1923;188:277-278.
Putnam T.J. Treatment of hydrocephalus by endoscopic coagulation of the choroid plexus: description of a new instrument and preliminary report of results. New England Journal of Medicine. 1934;210:1373-1376.
Rekate H. Selecting patients for endoscopic third ventriculostomy. Neurosurg Clin North Am. 2004;15:39.
Renard V.M., Jallo G.I. Endoscopic third ventriculostomy. In: Jallo G.I., Conway J.E., Bognar L. Neuroendoscopy of the Central Nervous System. San Diego: Plural; 2008:43-50.
Sainte-Rose C., Cinalli G., Roux F.E., et al. Management of hydrocephalus in pediatric patients with posterior fossa tumors: the role of endoscopic third ventriculostomy. J Neurosurg. 2001;95:791-797.
Scarff J. Endoscopic treatment of hydrocephalus: description of a ventriculoscope and preliminary report of cases. Arch Neurol Psychiatry. 1936;35:853.
Sciubba D.M., Noggle J.C., Jallo G.I. Equipment for neuroendoscopic procedures. In: Jallo G.I., Conway J.E., Bognar L. Neuroendoscopy of the Central Nervous System. San Diego: Plural; 2008:13-22.
Siomin V., Cinalli G., Grotenhuis A., et al. Endoscopic third ventriculostomy in patients with cerebrospinal fluid infection and/or hemorrhage. J Neurosurg. 2002;97:519-524.
Smyth M.D., Tubbs R.S., Wellons J.C.3rd, et al. Endoscopic third ventriculostomy for hydrocephalus secondary to central nervous system infection or intraventricular hemorrhage in children. Pediatr Neurosurg. 2003;39:258-263.
Teo C., Rahman S., Boop F.A., Cherny B. Complications of endoscopic neurosurgery. Childs Nerv Syst. 1996;12:248-253. discussion 253
Yamini B., Refai D., Rubin C.M., Frim D.M. Initial endoscopic management of pineal region tumors and associated hydrocephalus: clinical series and literature review. J Neurosurg. 2004;100:437-441.
1. Clayton J., Sinha R., Macarthur D. Trends in neuroendoscopic practice into the 21st century: a 12 yr review of a single unit’s activity. Cerebrospinal Fluid Res. 3(Suppl 1), 2006. S6
2. Grant J.A. Victor Darwin Lespinasse: a biographical sketch. Neurosurgery. 1996;39:1232-1233.
3. Dandy W. Extirpation of the choroid plexus of the lateral ventricles in communicating hydrocephalus. Ann Surg. 1918;68:569.
4. Dandy W.E. An operative procedure for hydrocephalus. Bull Johns Hopkins Hosp. 1922;33:189-190.
5. Fay T., Grant F.C. Ventriculoscopy and intraventricular photography in internal hydrocephalus. JAMA. 1923;80:461-463.
6. Mixter W.J. Ventriculoscopy and puncture of the floor of the third ventricle. Boston Med Surg J. 1923;188:277-278.
7. Scarff J. Endoscopic treatment of hydrocephalus: description of a ventriculoscope and preliminary report of cases. Arch Neurol Psychiatry. 1936;35:853.
8. Putnam T.J. Treatment of hydrocephalus by endoscopic coagulation of the choroid plexus: description of a new instrument and preliminary report of results. N Engl J Med. 1934;210:1373-1376.
9. Putnam T. The surgical treatment of infantile hydrocephalus. Surg Gynecol Obstetr. 1943;76:171-182.
10. McNickle H. The surgical treatment of hydrocephalus. A simple method of performing third ventriculostomy. Br J Surg. 1947;34:302-307.
11. Enchev Y., Oi S. Historical trends of neuroendoscopic surgical techniques in the treatment of hydrocephalus. Neurosurg Rev. 2008;31:249-262.
12. Li K.W., Nelson C., Suk I., Jallo G.I. Neuroendoscopy: past, present, and future. Neurosurg Focus. 2005;19:E1.
13. Li K.W., Noggle J.C., Jallo G.I. History of neuroendoscopy. In: Jallo G.I., Conway J.E., Bognar L. Neuroendoscopy of the Central Nervous System. San Diego: Plural; 2008:1-4.
14. Nulsen F., Spitz E. Treatment of hydrocephalus by direct shunt from ventricle to jugular vain. Surg Forum. 399, 1951.
15. Anderson W.S. Neuroendoscopic optics. In: Jallo G.I., Conway J.E., Bognar L. Neuroendoscopy of the Central Nervous System. San Diego: Plural; 2008:5-12.
16. Abbott R. History of neuroendoscopy. Neurosurg Clin North Am. 2004;15:1-7.
17. Vries J.K. An endoscopic technique for third ventriculostomy. Surg Neurol. 1978;9:165-168.
18. Jones R., Stening W., Brydon M. Endoscopic third ventriculostomy. Neurosurgery. 1990;26:86.
19. Jones R.F., Kwok B.C., Stening W.A., Vonau M. Neuroendoscopic third ventriculostomy. A practical alternative to extracranial shunts in non-communicating hydrocephalus. Acta Neurochir Suppl. 1994;61:79-83.
20. Rekate H. Selecting patients for endoscopic third ventriculostomy. Neurosurg Clin North Am. 2004;15:39.
21. Etus V., Ceylan S. Success of endoscopic third ventriculostomy in children less than 2 years of age. Neurosurg Rev. 2005;28:284-288.
22. Kulkarni A.V., Drake J.M., Mallucci C.L., et al. Endoscopic third ventriculostomy in the treatment of childhood hydrocephalus. J Pediatr. 2009;155:254-259.
23. Partington M.D. Congenital hydrocephalus. Neurosurg Clin North Am. 2001;12:737-742. ix
24. Chahlavi A., El-Babaa S.K., Luciano M.G. Adult-onset hydrocephalus. Neurosurg Clin North Am. 2001;12:753-760. ix
25. Nugent G.R., Al-Mefty O., Chou S. Communicating hydrocephalus as a cause of aqueductal stenosis. J Neurosurg. 1979;51:812-818.
26. Yamini B., Refai D., Rubin C.M., Frim D.M. Initial endoscopic management of pineal region tumors and associated hydrocephalus: clinical series and literature review. J Neurosurg. 2004;100(5 Suppl Pediatrics):437-441.
27. O’Brien D.F., Hayhurst C., Pizer B., Mallucci C.L. Outcomes in patients undergoing single-trajectory endoscopic third ventriculostomy and endoscopic biopsy for midline tumors presenting with obstructive hydrocephalus. J Neurosurg. 2006;105(Suppl 3):219-226.
28. Macarthur D.C., Buxton N., Punt J., et al. The role of neuroendoscopy in the management of brain tumours. Br J Neurosurg. 2002;16:465-470.
29. Bhatia R., Tahir M., Chandler C.L. The management of hydrocephalus in children with posterior fossa tumours: the role of pre-resectional endoscopic third ventriculostomy. Pediatr Neurosurg. 2009;45:186-191.
30. Sainte-Rose C., Cinalli G., Roux F.E., et al. Management of hydrocephalus in pediatric patients with posterior fossa tumors: the role of endoscopic third ventriculostomy. J Neurosurg. 2001;95:791-797.
31. Stein S.C., Schut L. Hydrocephalus in myelomeningocele. Childs Brain. 1979;5:413-419.
32. McLone D.G. Care of the neonate with a myelomeningocele. Neurosurg Clin North Am. 1998;9:111-120.
33. Rekate H.L. Neurosurgical management of the newborn with spina bifida. In: Rekate H.L., editor. Comprehensive Management of Spina Bifida. Boca Raton, FL: CRC Press; 1991:1-22.
34. Jenkinson M.D., Hayhurst C., Al-Jumaily M., et al. The role of endoscopic third ventriculostomy in adult patients with hydrocephalus. J Neurosurg. 2009;110:861-866.
35. Fukuhara T., Shimizu T., Namba Y. Limited efficacy of endoscopic third ventriculostomy for hydrocephalus following aneurysmal subarachnoid hemorrhage. Neurol Med Chir. 2009;49:449-455.
36. Komotar R.J., Olivi A., Rigamonti D., Tamargo R.J. Microsurgical fenestration of the lamina terminalis reduces the incidence of shunt-dependent hydrocephalus after aneurysmal subarachnoid hemorrhage. Neurosurgery. 51, 2002. 1403–1412
37. Siomin V., Cinalli G., Grotenhuis A., et al. Endoscopic third ventriculostomy in patients with cerebrospinal fluid infection and/or hemorrhage. J Neurosurg. 2002;97:519-524.
38. Smyth M.D., Tubbs R.S., Wellons J.C.3rd, et al. Endoscopic third ventriculostomy for hydrocephalus secondary to central nervous system infection or intraventricular hemorrhage in children. Pediatr Neurosurg. 2003;39:258-263.
39. Gangemi M., Maiuri F., Naddeo M., et al. Endoscopic third ventriculostomy in idiopathic normal pressure hydrocephalus: an Italian multicenter study. Neurosurgery. 2008;63:62-69.
40. McGirt M.J., Woodworth G., Coon A., et al. Diagnosis, treatment, and analysis of long-term outcomes in idiopathic normal-pressure hydrocephalus. Neurosurgery. 2005;57:699-705.
41. Koch D., Wagner W. Endoscopic third ventriculostomy in infants of less than 1 year of age: which factors influence the outcome? Childs Nerv Syst. 2004;20:405-411.
42. Kim S.K., Wang K.C., Cho B.K. Surgical outcome of pediatric hydrocephalus treated by endoscopic III ventriculostomy: prognostic factors and interpretation of postoperative neuroimaging. Childs Nerv Syst. 2000;16:161-168. discussion 169
43. Siomin V., Cinalli G., Grotenhuis A., et al. Endoscopic third ventriculostomy in patients with cerebrospinal fluid infection and/or hemorrhage. J Neurosurg. 2002;97:519-524.
44. Sciubba D.M., Noggle J.C., Jallo G.I. Equipment for neuroendoscopic procedures. In: Jallo G.I., Conway J.E., Bognar L. Neuroendoscopy of the Central Nervous System. San Diego: Plural; 2008:13-22.
45. Renard V.M., Jallo G.I. Endoscopic third ventriculostomy. In: Jallo G.I., Conway J.E., Bognar L. Neuroendoscopy of the Central Nervous System. San Diego: Plural; 2008:43-50.
46. Conway J.E., Baird C.J., Li K.W., et al. Ventricular anatomy. In: Jallo G.I., Conway J.E., Bognar L. Neuroendoscopy of the Central Nervous System. San Diego: Plural; 2008:23-36.
47. Drake J.M., Iantosca M.R. Cerebrospinal fluid shunting and management of pediatric hydrocephalus. In: Schmidek H.H., Roberts D.W. Schmidek and Sweet’s Operative Techniques in Neurosurgery. 5th ed. Philadelphia: Elsevier; 2005:487-508.
48. Iantosca M., Hader W., Drake J. Results of endoscopic third ventriculostomy. Neurosurg Clin North Am. 2004;15:67.
49. Walker M. Complications of third ventriculostomy. Neurosurg Clin North Am. 2004;15:61.
50. Teo C., Rahman S., Boop F.A., Cherny B. Complications of endoscopic neurosurgery. Childs Nerv Syst. 1996;12:248-253. discussion 253
51. Abtin K., Thompson B.G., Walker M.L. Basilar artery perforation as a complication of endoscopic third ventriculostomy. Pediatr Neurosurg. 1998;28:35-41.
52. McLaughlin M.R., Wahlig J.B., Kaufmann A.M., Albright A.L. Traumatic basilar aneurysm after endoscopic third ventriculostomy: case report. Neurosurgery. 1997;41:1400-1403. discussion 1403–1404
53. Schroeder H.W., Warzok R.W., Assaf J.A., Gaab M.R. Fatal subarachnoid hemorrhage after endoscopic third ventriculostomy. Case report. J Neurosurg. 1999;90:153-155.
54. Fukuhara T., Vorster S.J., Luciano M.G. Risk factors for failure of endoscopic third ventriculostomy for obstructive hydrocephalus. Neurosurgery. 2000;46:1100-1109. discussion 1109–1111