Endocrine Anatomy and Physiology
Maintaining dynamic equilibrium among the various cells, tissues, organs, and systems of the human body is a highly complex and specialized process. Two systems regulate these critical relationships: the nervous system and the endocrine system. The nervous system communicates by nerve impulses that control skeletal muscle, smooth muscle tissue, and cardiac muscle tissue. The endocrine system controls and communicates by distributing potent hormones throughout the body. Figure 31-1 lists the endocrine glands and their hormones, target tissues, and actions. When stimulated, the endocrine glands secrete hormones into surrounding body fluids. In the circulation, these hormones travel to specific target tissues, where they exert a pronounced effect. Receptors found on or within these specialized target tissue cells are equipped with molecules that recognize the hormone and bind it to the cell, producing a specific response.
Pancreas
Anatomy
Exocrine Cells
Specialized exocrine cells within the pancreas secrete digestive enzymes into a duct that is 3 mm wide and transverses the length of the pancreas. The pancreatic duct joins with the cystic duct, carrying bile from the liver and gallbladder, before it empties into the duodenum at the major duodenal papilla. This common ductal exit for the two organs raises the danger of a gallstone lodging at the duodenal papilla and blocking the outflow of pancreatic enzymes. Pancreas exocrine anatomy is illustrated in Figure 28-9 in Chapter 28, and pancreatic digestive juices are discussed as part of the physiology of the gastrointestinal (GI) system. Most pancreatic tissue is devoted to production of exocrine digestive juices.
Physiology
In the pancreas, clusters of cells that appear to form tiny islands among the exocrine cells accomplish the pancreatic endocrine functions. These clusters are known as the islets of Langerhans and are composed of four distinct cell types: alpha, beta, delta, and PP. The locations of the cells that produce these hormones are shown in Figure 31-2. Alpha cells secrete glucagon, beta cells secrete insulin, delta cells secrete somatostatin, and PP cells secrete pancreatic polypeptide hormone. Glucagon, insulin, somatostatin, and polypeptide hormones are released into the surrounding capillaries to empty into the portal vein, where they are distributed to target cells in the liver. The hormones then travel into general circulation to reach other target cells.
Insulin
Insulin is a potent anabolic hormone produced by the beta cells of the pancreas. Elevated levels of blood glucose stimulate insulin production. Insulin is the only hormone produced in the body that directly lowers blood glucose levels. Insulin is responsible for the storage of carbohydrate, protein, and fat nutrients. Insulin also augments the transport of potassium into the cells, decreases the mobilization of fats, and stimulates protein synthesis (Table 31-1). Box 31-1 defines the terms commonly used when discussing glucose and insulin balance. The major stimulant for insulin secretion is an elevation of serum glucose. The greater the rise in blood glucose, the more insulin the normal pancreas produces. Other hormones inhibit the release of insulin (Table 31-2).
TABLE 31-1
CELL | HORMONE | STIMULANT RELEASE FACTOR | TARGET TISSUE | RESPONSE OR ACTION |
Alpha | Glucagon | ↓ Glucose | Hepatocyte | ↑ Glucose in bloodstream |
Exercise | Myocyte | ↑ Gluconeogenesis | ||
↑ Amino acids | ↑ Glycogenolysis | |||
SNS stimulation | ↑ Fat mobilization | |||
↑ Protein mobilization | ||||
Beta | Insulin | Glucose | Skeletal cells | ↓ Blood glucose |
Muscle cells | ↓ Fat mobilization | |||
Cardiac cells | ↑ Fat storage | |||
↓ Protein mobilization | ||||
↑ Protein synthesis | ||||
↑ Glucogenesis | ||||
Delta | Somatostatin | Hyperglycemia | Alpha cells | ↓ Blood glucose |
Beta cells | ↓ Glycogen secretion | |||
↓ Insulin secretion | ||||
PP | Pancreatic polypeptide | Acute hypoglycemia | Gallbladder | ↑ Gallbladder contraction |
Smooth muscle | ↓ Pancreatic enzyme |
SNS, Sympathetic nervous system; ↑, increases; ↓, decreases.
TABLE 31-2
AGENTS THAT PROMOTE OR INHIBIT INSULIN RELEASE
INSULIN RELEASE (MAJOR STIMULANT: HIGH BLOOD GLUCOSE) | INSULIN INHIBITION (MAJOR INHIBITOR: LOW BLOOD GLUCOSE) |
Hormones | |
Glucagon | Somatostatin |
Corticotropic hormone | Norepinephrine |
Thyrotropin | Epinephrine |
Somatotropin | |
Glucocorticoids | |
Incretins | |
Medications | |
Beta-adrenergic stimulators | Beta-adrenergic blocking agents |
Sulfonylurea | Diazoxide |
Theophylline | Phenytoin |
Acetylcholine | Thiazide or sulfonamide diuretics |
Blood Glucose
Carbohydrate Anabolism.
Glucose is admitted to the skeletal, cardiac, and adipose cells for use as energy in the presence of effective insulin facilitated by the glucose transporter 4 (GLUT4), as described below. The movement of glucose from the circulation into the intracellular compartment reduces the concentration of glucose in the bloodstream and helps preserve the blood’s osmolality. Simultaneously, glucose is available to the cell as its main energy source. Excess glucose in the form of glycogen is stored in the hepatic and muscle cells for use as fuel at a later time. In skeletal muscle, 90% of the glucose in the cell is converted to glycogen for longer-term storage.1 Liver glycogen contributes up to 10% of total liver weight when fully replete.1
Fat Anabolism.
Adequate, effective insulin levels affect fat metabolism. Dyslipidemias are strongly associated with type 2 diabetes. Type 2 diabetes is characterized by overproduction of large, triglyceride-rich, very-low-density lipoproteins (VLDLs).2 Disorders of carbohydrate and fat metabolism are also associated with metabolic syndrome, a precursor to diabetes and cardiovascular disease.3
Glucagon
Glucagon, synthesized by alpha cells in the pancreas, has the opposite effect of insulin. Glucagon is released during hypoglycemia to induce hepatic glucose output. Because glucagon counter-regulates insulin levels and raises blood glucose levels, it is a potent gluconeogenic hormone. By means of gluconeogenesis, glucagon can form glucose from noncarbohydrate sources such as fat and protein when required. Glucagon release from the pancreas is stimulated by low blood glucose levels, starvation, exercise, or stimulation of the sympathetic nervous system (SNS), as listed in Box 31-2.4,5 Glucagon release protects the brain from the consequences of hypoglycemia.5
To meet short-term energy requirements, glucagon stimulates the release of glycogen stores from liver and muscle cells. Through a process called glycogenolysis, the glycogen stored in the liver is converted into a glucose form that can be used by the cells.5
For long-term energy needs, glucagon stimulates glucose release through the more complex process of gluconeogenesis. In gluconeogenesis, fat and protein nutrients are rapidly broken down into end products that are then changed into glucose.5
A normal blood glucose level is maintained in the healthy body by the insulin-to-glucagon ratio. When the blood glucose level is high, insulin is released, and glucagon is inhibited. When blood glucose levels are low, glucagon rather than insulin is released to raise the blood glucose level. The brain has a very limited supply of glucose, and glucagon release is essential to protect the brain from the effects of hypoglycemia.6
Somatostatin
Somatostatin is a hormone that is produced in the pancreatic delta cells. Somatostatin decreases glucagon secretion, and in high quantities, it decreases insulin release (see Table 31-1). Hyperglycemia stimulates the activity of the delta cells. It is theorized that the release of insulin enables somatostatin to control beta cell activity. Somatostatin may be involved in the regulation of the postprandial influx of glucose into cells.
Glucose Transporters
Human cells take up glucose by means of facultative glucose-transport proteins known by the term glucose transporter (GLUT). These proteins are specialized by tissue distribution and function as described in Table 31-3.7 At the cellular level, glucose crosses the cell plasma membrane through aqueous pores formed by GLUT transporters. At this time, 14 GLUTs have been identified.8 The GLUT number indicates the order in which the molecular sequence and GLUT tissue locations were identified.8 The functions of a few key GLUTs are described in more detail below.
TABLE 31-3
GLUCOSE TRANSPORTER (GLUT) | ANATOMIC LOCATIONS | FUNCTION |
GLUT1 | Erythrocytes, endothelial cells of brain | Basal glucose uptake |
Transport across blood–brain barrier | ||
GLUT2 | Pancreatic beta bells, liver, kidney, small intestine | High-capacity, low-affinity GLUT |
Can transport fructose | ||
GLUT3 | Brain cells, nerve cells | Transports glucose into neural tissue |
GLUT4 | Striated muscle and adipose tissue | Insulin-regulated transport in muscle and fat |
GLUT5 | Intestine, kidney, testis | Transports fructose |
GLUT6 | Spleen, leukocytes, brain | |
GLUT7 | Small intestine, colon, testis | Transports fructose |
GLUT8 | Testis, brain, muscle, adipocytes | Fuel supply of mature spermatozoa |
GLUT9 | Liver, kidney | |
GLUT10 | Liver, pancreas | Muscle-specific fructose transporter |
GLUT11 | Heart, muscle | |
GLUT12 | Heart, prostate, mammary gland |
GLUT1 and GLUT3.
The central nervous system (CNS) is freely permeable to glucose transported by GLUT1 and GLUT3.6,9 The CNS does not rely on insulin for transport of glucose across the neural cell membrane. The brain and other CNS cells require a constant source of glucose because they retain minimal glucose and glycogen stores.
GLUT2.
The GLUT2 proteins are associated with glucose sensing and facilitate rapid entry of glucose into specialized cells such as the pancreatic beta cells and into the glucose-sensing cells in the hepatoportal vein area.8 In the intestine, in the presence of a high-glucose meal, GLUT2 proteins may translocate to the apical cell surface to increase glucose absorption from the gut to the bloodstream.8
GLUT4.
The GLUT4 protein plays a pivotal role in the way glucose moves from the bloodstream into the cell.10 After a meal, the levels of sugars and amino acids in the bloodstream rise. This increase signals pancreatic beta cells to release insulin into the bloodstream. As the insulin circulates in the vascular system, it activates an insulin receptor on the plasma membrane of cells, primarily peripheral muscle and adipose cells. This receptor initiates signaling cascades inside the cell to activate GLUT4, which resides within the cells in clathrin-coated pits until needed. GLUT4 translocates (travels) from intracellular storage sites to the plasma membrane in response to the signal from the insulin receptor.10,11 At the cell surface, GLUT4 facilitates the passive transport of glucose along a concentration gradient into striated muscle and fat cells.
In the baseline state (between meals with normal blood glucose) only 4% to 10% GLUT4 are on the cell surface, whereas 90% are within the cell.12 Within 10 to 15 minutes of insulin stimulation of muscle cells, GLUT4 levels at the cell surface double as rapid translocation from the interior to the cell surface occurs.12 Between meals, the liver normally provides sufficient glucose output to maintain constant circulating blood glucose levels within the normal range.
Incretins
The incretins, which are hormones that are released from the GI tract after a meal, increase the production of insulin from the pancreatic beta cells. Two incretins are of particular clinical importance: 1) glucagon-like-peptide 1 (GLP-1) and 2) glucose-dependent insulinotropic polypeptide (GIP). The physiology of the incretins has been used to develop new medicines that reduce postprandial blood glucose levels in type 2 diabetes as listed in Table 33-3 in Chapter 33. More insulin is released following oral glucose ingestion than in response to the same amount of intravenous glucose because of release of the gut incretins.13,14 The increase in insulin section caused by stimulation from the incretins may make up to 70% of the insulin response, depending on the size of the meal.13,14 This incretin-effect is impaired in patients with type 2 diabetes.13,14 The physiologic effects of GIP and GLP-1 are listed in Table 31-4.
TABLE 31-4
INCRETINS IMPACT ON METABOLISM
ACTIONS | GIP | GLP-1 |
Pancreas | Stimulates insulin synthesis and release from pancreatic beta cells after a meal when BG is elevated | Stimulates insulin synthesis and release from pancreatic beta cells after a meal when BG is elevated |
Maintains beta cell mass and function Decreases beta cell death (apoptosis) |
Maintains beta cell mass and function Decreases beta cell death (apoptosis) |
|
Increases GLUT2 expression in pancreas | Increases GLUT2 expression in pancreas | |
Increases somatostatin release from the pancreatic delta cells | ||
Decreases glucagon release from the pancreas | ||
Liver | Decreases release of glucose from the liver | |
Gastrointestinal system | Delays gastric emptying | |
Central nervous system | Decreases appetite, sense of satiety after a meal | |
Muscle | Increases glucose uptake in muscle | |
Adipose tissue | Increases glucose uptake and free fatty acid synthesis to triglycerides | |
Bone | Increases bone formation Decreases bone resorption |
Increases bone formation Decreases bone resorption |
The incretins have other beneficial physiologic effects, including proliferation of pancreatic beta cells, decrease in beta cell death (apoptosis), and increase in GLUT2 proteins in the islets of Langerhans. Incretins augment the effects of insulin to increase glucose and fatty-acid uptake and storage in triglycerides.15
Glucagon-Like-Peptide 1.
GLP-1 is synthesized and released from the L-cells of the ileum and colon.13 When blood glucose levels are high, GLP-1 stimulates insulin release from the pancreas and inhibits glucagon release from the liver. GLP-1 has a short half-life of less than 2 minutes because it is rapidly broken down by the enzyme dipeptidyl peptidase-4 (DPP-4).16 Gliptins are medicines that have been developed to inhibit the DPP-4 enzyme and slow the inactivation of endogenous GLP-1 to reduce postprandial glucose elevation after a meal.
GLP-I exerts other beneficial effects, including decreased gastric emptying and the feeling of satiety (feeling of being full) after a meal. When blood glucose levels are within normal range, GLP-1 inhibits the release of somatostatin from the pancreatic delta cells and glucagon from the liver.14
Pituitary Gland and Hypothalamus
Anatomy
The hypothalamus lies in the base of the brain, superior to the pituitary gland. It is composed of specialized nervous tissue responsible for the integrated functioning of the nervous system and endocrine system, which is called neuroendocrine control. The hypothalamus weighs approximately 4 grams (g) and forms the walls and lower portion of the third ventricle of the brain. The area composing the floor of the ventricle thickens in the center and elongates. It is from this funnel-shaped portion, called the infundibular stalk or stem, that the pituitary gland is suspended, as illustrated in Figure 31-3. The infundibular stalk contains a rich vascular supply and a network of communicating neurons that travel from the hypothalamus to the pituitary. The vascular network and neural pathways transport chemical and neural signals and maintain constant communication between the nervous system and the endocrine system.
The pituitary gland is also called the hypophysis. It is attached below the hypothalamus and is found recessed in the base of the cranial cavity in a hollow depression of the sphenoid bone known as the sella turcica. Secured in such a protected environment, the pituitary is one of the most inaccessible endocrine glands in humans. However, because of this location, the pituitary gland is susceptible to injury from surgical and accidental trauma to the face and head. The pituitary is composed of the anterior lobe and the posterior lobe (see Fig. 31-3). Each component within the pituitary has its own origin, morphology, and function.
Anterior Pituitary
The anterior lobe of the pituitary, also called the adenohypophysis, is the largest portion of the gland. It communicates with the hypothalamus by means of a vascular network. The glandular tissue of the anterior pituitary produces several hormones, including adrenocorticotropic hormone (ACTH), thyroid-stimulating hormone (TSH), follicle-stimulating hormone (FSH), luteinizing hormone (LH), growth hormone (GH), and prolactin. Information about all the hormones, their target tissues, and their actions is found in Figure 31-1.
Physiology
Antidiuretic Hormone
ADH has two functions: 1) by means of the V1 receptors, it constricts smooth muscles within the arterial wall and 2) through V2 receptors, it regulates fluid balance by altering the permeability of the kidney tubule to water. ADH also contributes to control of the sodium level in the extracellular fluid by control of plasma osmolality. The sodium ion concentration in the plasma largely determines plasma osmolality. Osmoreceptors, located in the hypothalamus are sensitive to changes in the circulating plasma osmolality.17
Disorders of water metabolism are divided into hyperosmolar and hypo-osmolar states. Hyperosmolar disorders have a deficit of body water relative to body solute. Hypo-osmolar disorders have an excess of body water relative to total body solute.18
A low sodium level is associated with a low serum osmolality (hypo-osmolar state). When sodium levels rise, plasma osmolality increases (hyperosmolar state). ADH is then released to stimulate water resorption at the nephron to maintain sodium balance. This process decreases water loss from the body and subsequently concentrates and reduces urine volume. Fluid conserved in this manner is returned to the circulating plasma, where it dilutes the concentration (osmolality) of plasma, as shown in Figure 31-4.
The release of ADH increases with hypovolemia. Primarily, the plasma osmotic pressure and the volume of circulating blood regulate the release of ADH. Stretch receptors located in the left atrium are sensitive to volume changes in the plasma that may be caused by vomiting, diarrhea, or blood loss. Hemorrhage that is sufficient to lower the blood pressure or emesis that is sufficient to reduce fluid volume stimulates the release of ADH. Other factors capable of influencing ADH secretion are pain, stress, malignant disease, surgical intervention, alcohol, and some medications. Table 31-5 lists additional factors that affect ADH levels.
TABLE 31-5
FACTORS AFFECTING ANTIDIURETIC HORMONE
ANTIDIURETIC HORMONE STIMULATION | ANTIDIURETIC HORMONE RESTRICTION |
Increased serum osmolality | Decreased serum osmolality |
Emesis | Hypervolemia |
Hypovolemia | Water intoxication |
Hemorrhage | Cold |
Pain | Congenital defect |
Carbon dioxide inhalation | |
Hypothalamic-Pituitary System Damage | |
Accidental trauma | Accidental trauma |
Surgical trauma | Surgical trauma |
Pathologic trauma | Pathologic trauma |
Stress: physical and emotional | |
Acute infections | |
Malignancies | |
Nonmalignant pulmonary disorders | |
Stimulated pulmonary baroreceptors | |
Nocturnal sleep | |
Medications | |
Nicotine | Phenytoin |
Barbiturates | Chlorpromazine |
Oxytocin | Reserpine |
Glucocorticoids | Norepinephrine |
Anesthetics | Ethanol |
Acetaminophen | Opioids |
Amitriptyline | Lithium |
Carbamazepine | Demeclocycline |
Cyclophosphamide | Tolazamide |
Chlorpropamide | |
Potassium-depleting diuretics | |
Vincristine | |
Isoproterenol |
Thyroid Gland
Anatomy
The thyroid gland weighs 15 to 25 g in the adult human.19 The size of the adult gland varies according to the availability of dietary iodine in different geographic regions. The gland partially encases the trachea, is wrapped around the second to fourth tracheal rings anteriorly and laterally, and is located at the level of the sixth and seventh cervical vertebrae posteriorly. The thyroid gland lies inferior the thyroid cartilage and the articulating surface of the cricoid cartilage. This bow tie–shaped gland has two lateral lobes that are partially covered by the sternohyoid and sternothyroid muscles. The thyroid isthmus, the band of narrow thyroid tissue that connects the lateral lobes, lies directly inferior to the cricoid cartilage, as shown in Figure 31-5.
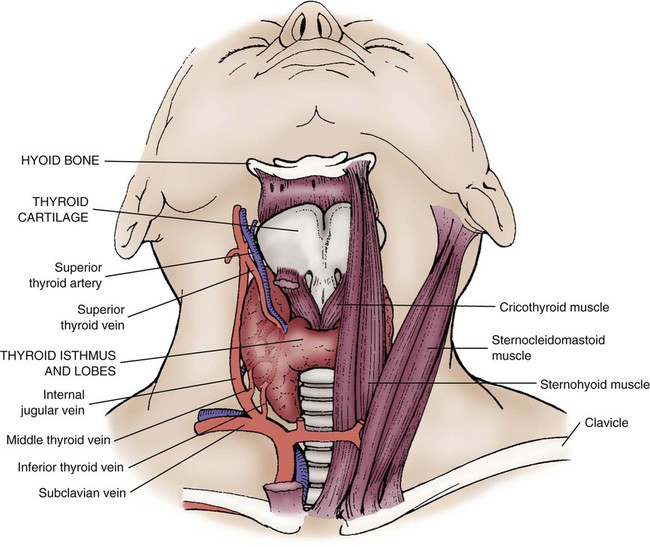
Two important nerves associated with speech and swallowing pass close to the thyroid gland. The recurrent laryngeal nerve and superior laryngeal nerve are branches of the vagus nerve. The considerable anatomic variety in the location of these nerves increases the risk of injury during surgical procedures such as thyroidectomy.19
The thyroid gland has a rich blood supply from the superior and inferior thyroid arteries. The superior thyroid artery is the first branch of the external carotid artery.19 Venous drainage is from the superior, middle, and inferior thyroid veins. Lymphatic drainage follows the route of the thyroid veins.19 The functional units of the thyroid gland are spherical cells called follicles. Follicles are filled with the protein thyroglobulin.20
Physiology
Triiodothyronine and Thyroxine
TSH prompts the thyroid cells to produce thyroid hormones (T3 and T4) in the presence of iodine in the thyroid follicles. In the normal thyroid gland, 90% of the thyroid hormone that is produced is in the form of thyroxine (T4) and 10% as triiodothyronine (T3). These hormones are named according to the number of iodine atoms in their structure; T3 has three iodine atoms and T4 has four iodine atoms.20
Hypothalamic–Pituitary–Thyroid Axis Feedback Loop
The hypothalamic–pituitary–thyroid axis regulates the mechanism for the synthesis and secretion of thyroid hormone. The production and secretion of thyroid hormone is regulated by a feedback mechanism that limits the amount of hormone circulating to the cellular need at that time, as illustrated in Figure 31-6.
In response to decreased circulating levels of T3 and T4, the hypothalamus releases TRH. TRH activates TSH in the anterior pituitary and TSH stimulates the thyroid gland to manufacture and release the thyroid hormones T3 and T4 in the presence of iodine.20 When serum blood levels of T3 and T4 become high, the pituitary inhibits the production of additional TSH. When levels of T3 and T4 become too low, the pituitary is stimulated to secrete additional TSH.
T4 prompts the activation of beta-adrenergic receptors in widespread areas of the body. These receptors trigger an SNS response and release norepinephrine at sympathetic nerve endings.21 The effect is stimulation of the cardiac tissue, nervous tissue, and smooth muscle tissue, as well as an increase in metabolism and thermogenesis (increased body heat). Box 31-3 lists the major actins of thyroid hormones in more detail.
Adrenal Gland
Anatomy
The adrenal glands, also called suprarenal glands, are small, yellowish, bilateral, pyramidal or semilunar-shaped organs located at the superior pole of the kidney. As a neighbor to the kidney, they are retroperitoneal and embedded in the fat pad of the kidney. The normal adrenal gland is 3 to 4 cm in its longest axis and weighs approximately 5 g in the adult (Fig. 31-7). Functionally and histologically, two glands exist within the suprarenal gland: 1) the outer cortex and 2) the inner medulla. Both regions secrete hormones that are integral to the body’s response to stress.
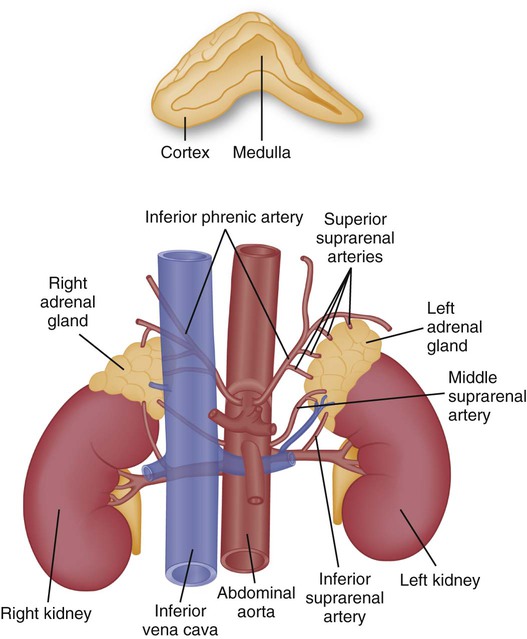
A, Cross section of the adrenal gland showing the outer cortex and inner medulla. B, Anatomical relationship of the adrenal glands to the kidneys.
Adrenal Medulla
The inner region is called the adrenal medulla. The inner medulla is a part of the SNS, and it resembles a cluster of neurons more than an endocrine gland. The adrenal medulla contains clusters of specialized chromaffin cells, which are modified preganglionic sympathetic neurons.22,23 Different sets of chromaffin cells contain chromaffin granules that are specific for each of catecholamines epinephrine or norepinephrine.22 The granules for each catecholamine appear in different sets of cells within the adrenal medulla. A chromaffin cell usually contains granules only for one catecholamine or the other.22
The adrenal medulla is stimulated by the SNS by preganglionic bundles of sympathetic nerve fibers that originate in the spinal cord.22 The role of the chromaffin cells is to secrete the catecholamines epinephrine and norepinephrine. Under physiologic stress, these hormones produce a widespread excitatory effect described as a “surge of adrenaline” or as the “fight-or-flight response.”23
Adrenal Blood Supply
The rich arterial blood supply comes to the adrenal gland from three sources (see Fig. 31-7): (1) the superior suprarenal artery is a branch of the inferior phrenic artery; (2) the middle suprarenal artery branches directly off of the aorta; and (3) the inferior suprarenal artery branches off the renal artery.
Physiology
Hormones of the Adrenal Cortex
Pharmacologic corticosteroids are administered to prevent rejection of newly transplanted solid organs (see Chapter 37). Corticosteroids are also used to treat inflammatory disorders, and they are administered when the adrenal gland is cortisol deficient.
The principal mineralocorticoid hormone aldosterone is secreted from the cells of the zona glomerulosa. Secretion of aldosterone is the final step in the RAAS. Aldosterone is secreted in response to intravascular hypovolemia, and its target of action is the distal tubules of the kidneys to retain more sodium and water in the bloodstream. In the healthy person, aldosterone contributes to the equilibrium of water and potassium in the body. Figure 15-18 in Chapter 15 illustrates the neurohormonal role of the RAAS in heart failure. In patients with heart failure, medications to block the effect of aldosterone on the kidneys often are prescribed. The most frequently used medication is spironolactone (Aldactone) (see “Neurohormonal Compensatory Mechanisms in Heart Failure” in Chapter 15). Some androgen hormones such as dehydroepiandrosterone (DHEA) are also secreted from the zona reticularis in the cortex. The function of DHEA is not fully understood.
Hormones of the Adrenal Medulla
The adrenal medulla (inner region) secretes two important catecholamines: epinephrine, also known as adrenaline, and norepinephrine, also known as noradrenaline. The adrenal medulla acts as a functional extension of the SNS. Stimulation of the SNS stimulates the chromaffin cells in the adrenal medulla to secrete predominantly epinephrine and some norepinephrine into the bloodstream. This results in an epinephrine surge described as the fight-or-flight response. In the critical care unit, intravenous infusions of epinephrine and norepinephrine are used in shock states to raise the blood pressure. The pharmacologic effects of these catecholamines are described in Chapter 16, Table 16-18.
Hypothalamic–Pituitary–Adrenal Axis
The adrenal glands are physiologically linked to the hypothalamus and the pituitary gland. When the brain perceives a stressful or threatening situation, the hypothalamus releases corticotropin-releasing hormone (CRH), which acts on the anterior pituitary to release adrenocorticotrophic hormone (ACTH), which circulates in the bloodstream to reach the adrenal cortex and stimulate glucocorticoid hormone release. Under stress-free conditions, cortisol is secreted in a diurnal pattern, and levels are highest in the early morning and lowest in the late evening.24 Illness or trauma disrupts this normal physiology with resultant loss of the diurnal pattern.24
Summary
Pancreas
• The pancreas is a long, triangular organ approximately 15 cm long and 4 cm wide. It is situated in the C-shaped curvature of the duodenum and extends behind and below the stomach toward the spleen.
• Insulin is released by the beta cells of the pancreas. An elevated blood glucose level is the stimulus for insulin secretion from the pancreas. The higher the blood glucose, the more insulin the normal pancreas produces.
• Glucagon is synthesized from the alpha cells in the pancreas. Its effect is the opposite of the effect of insulin. Glucagon release is stimulated by hypoglycemia, and it stimulates glucose output from the liver.
• In addition to insulin, human cells take up glucose by means of facultative glucose-transport proteins known as GLUTs.
• Incretins are hormones released from the GI tract after a meal that increase the production of insulin from the pancreatic beta cells.
Pituitary
• The pituitary gland is attached below the hypothalamus and recessed in the base of the skull in a hollow depression known as the sella turcica.
• The anterior pituitary gland secretes ACTH, TSH, and other hormones.
• The posterior pituitary gland secretes ADH, also known also as arginine vasopressin.
• Sodium and water metabolism are regulated by two complementary systems within the body; sodium metabolism by the RAAS and water metabolism by arginine vasopressin (ADH).
Thyroid
• The thyroid gland has a “bow-tie” shape. It is wrapped around the trachea anteriorly and laterally at the level of the sixth and seventh cervical vertebrae. The thyroid isthmus, the band of narrow thyroid tissue that connects the lateral lobes, lies directly below to the cricoid cartilage.
• The thyroid hormones are T4 and T3. Most T4 is converted to the more potent and biologically active T3 in the peripheral tissues, liver, and kidneys.
• The thyroid gland produces a third hormone, thyrocalcitonin (calcitonin), which acts in concert with parathyroid hormone to maintain normal calcium blood levels.
Adrenal
• The adrenal glands are small, pyramid-shaped organs located at the superior pole of the kidneys.
• The adrenal gland contains a cortex and medulla that represent two functionally different endocrine zones. The adrenal cortex secretes cortisol and aldosterone. The adrenal medulla secretes epinephrine and norepinephrine.