Effect of intracardiac shunts on inhalation induction
< ?xml:namespace prefix = "mml" />

Right-to-left shunt
These examples demonstrate that, with highly soluble agents, such as ether, uptake is limited primarily by ventilation. With poorly soluble agents, such as N2O, uptake is limited primarily by blood flow. Subsequently, the impact of shunting is greater with agents of lower solubility (Figures 63-1 and 63-2).
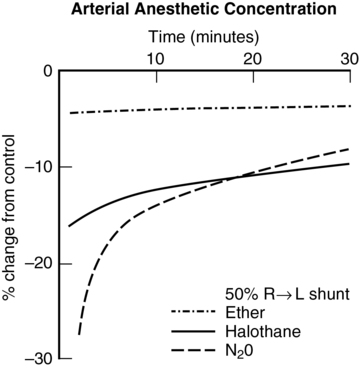
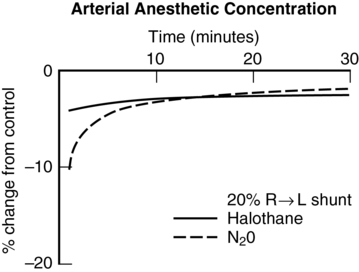
Left-to-right shunt
With a left-to-right shunt, no significant change occurs in the speed of induction, assuming that systemic blood flow is normal. If tissue perfusion is decreased because of the left-to-right shunt, then induction will initially be slowed because less anesthetic agent will be delivered to the brain per unit of time. CO usually increases to compensate for the shunting, and local control of vasculature maintains cerebral perfusion and minimizes the effect of the shunt (Figure 63-3).
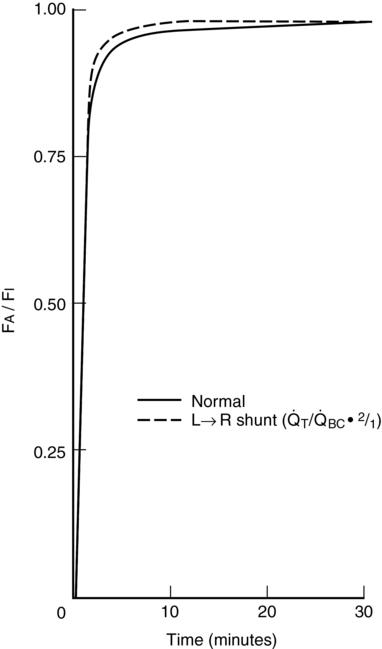











