Chapter 17 Disturbances of Smell and Taste
The senses of smell and taste play important roles in human safety, nutrition, and quality of life. In a study of 750 consecutive patients presenting to our center with chemosensory complaints, 68% reported altered quality of life, 46% reported changes in appetite or body weight, and 56% described adverse influences on daily living or psychological well-being (Deems et al., 1991a). In another study of 445 such patients, at least one hazardous event, such as food poisoning or failure to detect fire or leaking natural gas, was reported by 45.2% of those with anosmia, 34.1% of those with severe hyposmia, 32.8% of those with moderate hyposmia, 24.2% of those with mild hyposmia, and 19.0% of those with normal olfactory function (Santos et al., 2004). In a longitudinal study of 1162 older persons without dementia, mortality risk was 36% higher in those with low compared to high scores on a 12-item odor identification test after adjusting for such variables as sex, age, and education (Wilson et al., 2010). Of particular importance to the neurologist is the fact that chemosensory function can provide unique insight into neurological health. Thus, olfactory disturbances are among the early preclinical or presymptomatic signs of Alzheimer disease (AD) and sporadic Parkinson disease (PD) (Ross et al., 2008). Indeed, recent research suggests that a standardized odor identification test is as effective in detecting PD as a single-photon emission computed tomography (SPECT) scan employing [123I]ioflupane (DaTSCAN) (Deeb et al., 2010)
It is critical for the physician to realize that most complaints of decreased “taste” function reflect decreased olfactory function (Deems et al., 1991a). Flavor sensations such as cola, coffee, chocolate, strawberry, pizza, licorice, steak sauce, and vanilla depend upon stimulation of the olfactory receptors by molecules that enter the nasal pharynx during deglutition, a process called retronasal olfaction. Such “taste” sensations disappear when the olfactory epithelium is severely damaged, leaving intact only sensations from free nerve endings of the trigeminal nerve (CN V) and such taste bud–mediated sensations as sweet, sour, salty, bitter, and metallic. The ability to taste is much more resilient to pathological or trauma-related alterations than the ability to smell, largely reflecting the redundant innervation of the taste buds from multiple cranial nerves (i.e., CN VII, IX, X) (Deems et al., 1991a).
Anatomy and Physiology
Olfaction
The olfactory receptor cells, which number around 6 million in the human, are located within a pseudostratified columnar neuroepithelium that also contains sustentacular or supporting cells, basal cells (the precursors of other cell types within the epithelium), and the poorly understood microvillar cells. This epithelium lines the cribriform plate and sectors of the superior septum, the middle turbinate, and the superior turbinate and is supported by a highly vascularized lamina propria that contains Bowman glands, the major source of the overlying mucus and enzymes that detoxify xenobiotic agents. It is into this mucus that each of the bipolar receptor cells projects 3 to 30 receptor-bearing cilia that interact with odorant molecules. These cells are unique, since they serve as both a receptor cell and a first-order neuron and can regenerate to some degree from basal cells after being damaged. Moreover, they exhibit the most diverse molecular phenotype of any neuron, expressing a wide range of receptor protein types and cell-surface antigens. A photomicrograph of the surface of the olfactory epithelium is shown in Fig. 17.1.
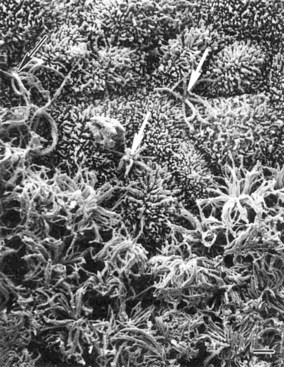
(From Menco, B.Ph.M., Morrison, E.E., 2003. Morphology of the mammalian olfactory epithelium: form, fine structure, function, and pathology. In: Doty, R.L. (Ed.), Handbook of Olfaction and Gustation, second ed. Marcel Dekker, New York, pp. 32-97, with permission.)
Unlike nearly all other central nervous system (CNS) neurons, the granule cells, as well as the largely dopaminergic periglomerular cells, undergo replacement over time (Altman, 1969). Astrocyte-like stem cells within the anterior subventricular zone of the brain generate large numbers of neuroblasts, some of which undergo restricted chain migration along the rostral migratory stream (Rousselot et al., 1994). This migration largely terminates within the granule cell layer of the olfactory bulb, from which some differentiating neuroblasts migrate more peripherally, thereby repopulating periglomerular cells. The architecture of the olfactory bulb, including its main cell types, is presented schematically in Fig. 17.2.
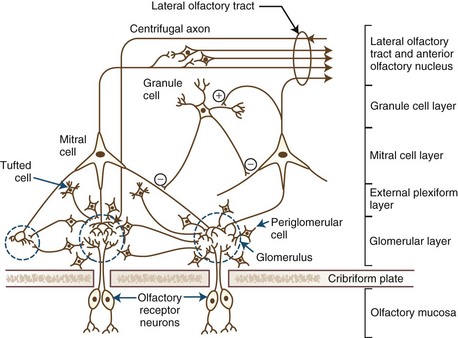
Fig. 17.2 Schematic of olfactory bulb structures, neurons, and layers.
(From Alloway, K.D., Pritchard, T.C., 2007. Medical Neuroscience. Hayes Barton, Raleigh, North Carolina, with permission.)
The relative roles of central brain structures in odor perception are poorly understood. The piriform cortex appears to encode higher-order representations of odor quality, identity, and familiarity. This brain region also plays a role in odor learning and memory, as well as in coordinating information between olfaction, taste, and vision (Gottfried et al., 2002). The entorhinal cortex preprocesses information entering the hippocampus, whereas the amygdala seems to respond to the intensity of emotionally significant odors. The rostral regions of the orbitofrontal cortex are involved in odor memory, whereas the caudal regions are associated with odor detection. The processing of hedonic information about odors seems to occur within the orbitofrontal cortex, with pleasant odors activating the medial orbitofrontal cortex and unpleasant odors activating the lateral orbitofrontal cortex.
Gustation
Taste plays a critical role in identifying substances in foods and beverages, such as sugars and poisonous alkaloids, that promote or disrupt homeostasis. The taste receptor cells are found within the taste buds, small flask-like structures located on the surface of the oral epithelium (Fig. 17.3). These cells extend microvilli into the lumen of the bud near its apical opening, termed the taste pore. Like olfactory receptor cells, taste receptors die and become replaced at various intervals from basal cells within the bud.
Humans possess approximately 7500 taste buds, most of which are found on lingual protuberances called papillae (Fig. 17.4). Taste buds innervated by the chorda tympani division of the facial nerve (CN VII) are found on the fungiform papillae, which are most dense on the tip and lateral margins of the anterior tongue. The palatine branch of the greater superficial petrosal division of CN VII innervates the taste buds on the soft palate. Some taste buds found on the anterior foliate papillae, located on a sector of the tongue’s posterior lateral margins, may also be innervated by branches of the chorda tympani nerve. Most foliate buds, as well as the buds on the circumvallate papillae—six to eight large structures that resemble flattened hills across the “chevron” of the posterior tongue—are innervated by the glossopharyngeal nerve (CN IX). Taste buds within the oral pharynx are supplied by the vagus (CN X) nerve. The small and somewhat pointed filiform papillae, which cover the entire tongue, harbor no taste buds. Although not involved in taste perception as such, the trigeminal nerve (CN V) participates in the formation of flavor via free nerve endings in the oral mucosa signaling sensations of touch, pain, and temperature. Thus, the fizziness of carbonated soft drinks and the warmth of coffee are dependent upon the stimulation of this nerve.
Individuals differ markedly in terms of the number and distribution of their taste buds. Although some physiology textbooks suggest that different regions of the tongue are responsible for the four basic taste qualities, this is an oversimplification of the facts. In general, the front of the tongue is more sensitive than other tongue regions to all taste qualities, although in the case of bitter, the back of the tongue is typically much more sensitive. The relative average sensitivity of tongue regions to the four prototypical taste qualities is shown in Fig. 17.5, although it must be emphasized that significant individual differences exist.
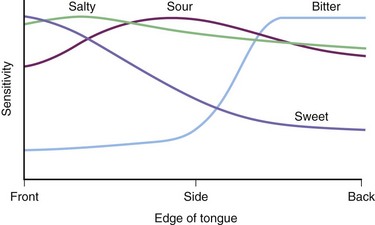
Fig. 17.5 Relative sensitivity of the edge of the tongue to the four classic taste qualities. Sensitivity reflects the reciprocal of the threshold value and is plotted as a ratio of maximal sensitivity = 1. Threshold data are from Hänig (1901). Note that all regions of the tongue that were evaluated were responsive to some degree to all stimuli, but that the anterior tongue was most sensitive to sweet, sour, and salty and least sensitive to bitter. The rear (base) of the tongue was relatively more sensitive to bitter.
(Adapted from Boring, E.G., 1942. Sensation and Perception in the History of Experimental Psychology. Appleton-Century Crofts, New York.)
The specific receptors involved in sensing taste stimuli have now been identified (Hoon et al., 1999). A small family of three G protein–coupled receptors (GPCRs)—T1R1, T1R2, and T1R3—encode sweet and umami (monosodium glutamate–like) sensations. Bitter sensations are mediated by the T2R receptors, a family of about 30 GPCRs that are expressed on cells different from those that express the sweet and umami receptors. The salty sensation of sodium chloride arises from the entrance of Na+ ions into the cells via specialized membrane channels such as the amiloride-sensitive Na+ channel. Although sour taste has been suggested to depend upon a range of receptors, PKD2L1 is likely the primary sour taste receptor.
The nerves innervating the taste buds converge centrally onto the nucleus of the solitary tract of the brainstem. Although species differences exist, the afferent taste nerve fibers can be classified electrophysiologically into categories based upon their relative responsiveness to sweet, sour, bitter, and salty-tasting stimuli. In the hamster, for example, sucrose-best, HCl-best, and NaCl-best fibers have been observed (Frank et al., 1988). Despite the fact that fibers are generally “tuned” for rather specific stimuli, they can nonetheless respond to other stimuli. For example, a few sucrose-best fibers also respond to NaCl and HCl. NaCl-best fibers and HCl-best fibers are less tightly tuned than sucrose-best fibers, with more fibers responding to multiple classes of stimuli.