Chapter 79 Determination of Brain Death in Infants and Children
The diagnosis of brain death in infants and children can be established after careful review of the medical history and performance of a detailed neurologic examination. Although criteria in children follow those established in adults, there are no universally accepted worldwide criteria for brain death determination in adults [Haupt and Rudolf, 1999; Wijdicks, 2001, 2002]. In the United States and most other countries, the 1987 pediatric guidelines [Report of Special Task Force, 1987] have been employed, with general agreement regarding their usefulness [Banasiak and Lister, 2003; Farrell and Levine, 1993; Kaufman, 1989], although criticisms about their validity and specificity, particularly in young infants, have been raised [Freeman and Ferry, 1988; Shewmon, 1988; Volpe, 1987]. These guidelines have recently been revised and will be described in detail in this chapter [Nakagawa et al., 2011].
Historical Perspective
A state beyond coma, or coma dépassé, was proposed in 1959 by Mollaret and Goulon to describe a premorbid clinical condition with loss of sensation, motor activity, consciousness, and vegetative functions [Mollaret and Goulon, 1959]. In 1968, an ad hoc committee of the Harvard Medical School faculty recommended clinical guidelines that subsequently shaped development of brain death concepts [Beecher, 1968]. The committee proposed that brain death could be defined by coma, apnea, lack of spontaneous or purposeful movements, and loss of selective cranial nerve functions, including the corneal reflex, pupillary response to light, pharyngeal gag and swallowing, yawning, vocalization, and failure of eye deviation to caloric stimulation of the tympanic membranes. Failure of improvement, sustained over 24 hours of observation, established a diagnosis of brain death. Two caveats were that the patient was normothermic and that drugs capable of maintaining coma were excluded. Two isoelectric electroencephalograms (EEGs) performed 24 hours apart were considered confirmatory, but not essential, in the declaration of brain death. In 1971, Mohandas and Chou emphasized the importance of determining the etiology of coma and documenting the persistence of apnea [Mohandas and Chou, 1971].
The Conference of Medical Royal College and Faculties in the United Kingdom proposed the following definition in 1976: “Permanent functional death of the brainstem constitutes brain death” [Conference, 1976]. Irreversible structural brainstem damage was defined carefully and believed to be clinically diagnosable without confirmatory EEG recordings. A follow-up report in 1979 recommended that brainstem death be considered death [Conference, 1979]. Subsequently a working group convened by the Royal College of Physicians further defined the criteria of brainstem death [Criteria, 1995]. In 1980, the National Institute of Neurologic and Communicative Disorders and Stroke (NINCDS) Collaborative Study of Brain Death reported on the outcome of 503 adult comatose and apneic patients, including neuropathologic brain studies and EEGs [NINCDS, 1980]. The combination of loss of pupillary light reflex, corneal reflex, oculocephalic reflex (doll’s-eye phenomenon), and oculovestibular reflex (caloric eye deviation) was highly predictive of death. Apnea, coma, and absence of brainstem reflexes, combined with an electrocerebral silent EEG, were highly associated with pathology in a brain that had experienced long-term respirator exposure (“respirator brain”). Exceptions were rarely noted (i.e., presence of respirator brains in patients with persistent biologic EEG activity and, conversely, lack of this pathology in patients with electrocerebral silence). EEG use was recommended in confirming brain death when the clinical neurologic examination was equivocal. An isoelectric EEG, despite its rare failure always to diagnose brain destruction, was predictive of a fatal outcome, except in two patients with drug intoxication. The report emphasized that a patient was not considered brain-dead if the EEG was not isoelectric. About 30 percent of the population had drug toxicity, despite the absence of a history of ingestion; 28 percent had measurable amounts of drugs that would suppress EEG activity. The report concluded that drug screening and EEG monitoring were mandatory before declaring brain death. Subsequent reports in adults [Grigg et al., 1987] and children [Ashwal and Schneider, 1979] documented that EEG activity could persist in unequivocally brain-dead patients.
In 1981, a U.S. presidential commission was convened to establish guidelines for the determination of brain death [President’s Commission, 1981]. These guidelines emphasized diagnosis based on the irreversible cessation of function of the entire brain. This report recognized that adult criteria might not be applicable to children because of developmental factors, a possible greater tolerance to asphyxia, and the recognition that infants and children occasionally exhibited significant recovery despite prolonged coma. The age of 5 years was selected as the earliest age suitable for the application of adult criteria, although this age selection reflected no known biologic phenomena.
In 1995, the American Academy of Neurology published criteria for the determination of brain death in adults [Practice Parameter, 1995]. These criteria were recently revised, recommending that only one examination be required, that ancillary testing was not necessary, and that the diagnosis could be made solely on clinical criteria [Wijdicks et al., 2010]. It was suggested, however, that if the examination was equivocal, additional neurodiagnostic testing could be done. In both the 1995 and 2010 parameters, it was stated that these recommendations were for individuals older than age 18 years. Box 79-1 summarizes the recommendations section of the 2010 American Academy of Neurology parameter that provided practical (non-evidenced-based) guidance for determination of brain death in adults.
Box 79-1 2010 American Academy of Neurology Recommendations for the Diagnosis of Brain Death in Adults
The determination of brain death can be considered to consist of four steps:
II The Clinical Evaluation (Neurologic Assessment)
B Absence of Brainstem Reflexes


C Apnea

Procedure






III Ancillary Tests


IV Documentation

(From Wijdicks et al. Evidence-based guideline update: determining brain death in adults: report of the Quality Standards Subcommittee of the American Academy of Neurology. Neurology 2010 Jun 8;74[23]:1911–1918.)
Seeking to remedy the lack of criteria for infants and children, multiple professional neurologic and pediatric societies convened a task force in 1987 to propose a pediatric standard for brain death determination [Report of Special Task Force, 1987]. A history of the etiology and reversibility of coma was emphasized, as was the loss of brainstem function and apnea. Age-dependent observation periods of 12–48 hours were recommended. Ancillary EEG or radionuclide scanning was recommended in children younger than 1 year of age. Infants less than 7 days of age were excluded because of a lack of supportive literature. Later studies confirmed the validity of applying these specific guidelines in newborns of greater than 34 weeks of conceptual life [Ashwal and Schneider, 1989]. More recently, the Society of Critical Care Medicine, the American Academy of Pediatrics, and the Child Neurology Society convened a committee to re-evaluate and revise pediatric brain death criteria. These revised recommendations for the diagnosis of brain death in infants and children were published in 2011 (Box 79-2 and Box 79-3).
1 Determination of Brain Death

2 Prerequisites for Initiating a Brain Death Evaluation
3 Number of Examinations, Examiners, and Observation Periods
4 Apnea Testing
5 Ancillary Studies
6 Declaration of Death
(Nakagawa TA et al. Guidelines for the determination of brain death in infants and children: an update of the 1987 Task Force recommendations. Crit Care Med 2011; in press.)
Box 79-3 Neurologic Examination Criteria for Brain Death in Infants and Children from the 2011 Guidelines
Legal Definition of Brain Death
The American Medical Association and the American Bar Association supported universal enactment of the Uniform Determination of Death Act published in 1980 [Uniform, 1980]. The Act defined death, stating that “an individual who has sustained either (1) irreversible cessation of circulatory and respiratory functions; or (2) irreversible cessation of all functions of the brain including the brainstem is dead.” Subsequently, all states of the United States have enacted legislation accepting death by this definition. Brain death can be declared despite heart-lung ventilator dependence.
Epidemiology
Incidence of Brain Death
The overall incidence of brain death in children is unknown. In the United States, data are available concerning the number of brain-dead children who become organ donors (see United Network Organ Sharing [UNOS] website at http://www.unos.org/), and more recent studies have suggested that approximately 55 percent of children declared brain-dead become organ donors [Sheehy et al., 2003]. Combining such data suggests there are approximately 1800 children per year in the United States declared brain-dead, with most being between 11 and 17 years of age (Figure 79-1). Accurate data for neonates and infants younger than 1 year of age are not available. There also was a decrease between 1993 and 2003 in the number of brain-dead children who were organ donors (from 1137 to 886 [22 percent]), suggesting that the total number of children who are annually being declared brain-dead has decreased (see UNOS website). This decrease likely is due to the 26 percent decline in the total number of pediatric deaths since the 1990s (data from the National Center for Health Statistics [website http://www.cdc.gov/nchs/]).
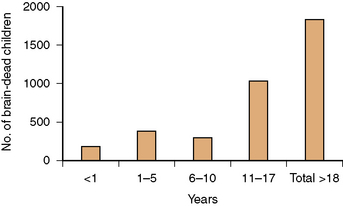
Fig. 79-1 Number of children declared brain-dead by age in the United States.
(Data from the United Network Organ Sharing website: http://www.unos.org/.)
Studies from pediatric intensive care units in the 1990s reported that the incidence of brain death in older infants and children ranges from 0.65 to 1.2 percent of admissions [Ashwal and Schneider, 1999]. In one study, the mortality rate in the pediatric intensive care unit was 8.7 percent, with 22 percent of these children declared brain-dead [Ryan et al., 1993]. The mortality rate in the neonatal intensive care unit was 5.6 percent, with none of the infants declared brain-dead. Another study reported the percentage of brain deaths in relation to the number of overall deaths to be 31.4 percent in children older than 1 month of age and 6.3 percent in neonates [Parker et al., 1995]. Similar findings from other countries have been reported [Goh et al., 1999; Gotay-Cruz and Fernandez-Sein, 2002; Lopez-Herce et al., 2000; Martinot et al., 1998]. Data from Loma Linda University Children’s Hospital indicate that the percentage of brain deaths in relation to the number of overall deaths is 28 percent and 2.1 percent in the pediatric and neonatal intensive care units, respectively. In some pediatric intensive care units, the percentage of patients diagnosed as brain-dead, compared with all deaths, is even higher (37 percent to 38 percent) [Martinot et al., 1995; Mejia and Pollack, 1995].
Etiologies of Brain Death
Table 79-1 provides information on the age distribution of infants and children in whom brain death has been diagnosed and characterization by disease categories that were responsible for the cerebral insult. Brain death most commonly occurs in adolescents and less so in infants younger than 1 year of age. Closed head injury was the most common clinical presentation leading to brain death (50 percent), followed by intracranial hemorrhage and stroke (12 percent). Asphyxial injury usually occurred as a complication of septic or hemorrhagic shock, with unexplained out-of-hospital cardiac arrest, or from strangulation or suffocation. Sudden infant death syndrome was a rare cause of brain death. Brain death secondary to meningitis was seen in patients who developed massive cerebral edema with herniation within 2–24 hours of admission. Miscellaneous causes of brain death involve rare metabolic diseases, perioperative insults to the central nervous system, acute hydrocephalus, and a variety of other rare disorders that ultimately affect the brain.
Outcome after Diagnosis of Brain Death
Most children are removed from life support or undergo organ donation within a 2-day time period when the diagnosis of brain death is confirmed [Ashwal and Schneider, 1987]. Some children are continued on ventilator support until cardiac arrest occurs, and their “survival” has averaged about 17 days. In rare cases, children have been maintained on ventilator support for periods ranging from 6 months to 5 years [Shewmon, 1998]. There have been no reports of children recovering neurologic function who met adult brain-death criteria on neurologic examination [Moshe and Alvarez, 1986; Rowland et al., 1983]. These criteria include an absence of spontaneous activity; specific cranial nerve dysfunction; and cardiac response to ocular compression (vagal bradycardia response), decerebrate or decorticate posturing, and apnea.
Neurologic Evaluation
Reports indicate that a marked variability exists in the knowledge and use of the 1987 pediatric brain-death guidelines [Ashwal, 1991; Mejia and Pollack, 1995]. A national survey of 16 pediatric intensive care units found that apnea testing either was not performed (25 percent) or was deemed “controversial” (22 percent) in children diagnosed as brain-dead, and in many of these infants and children, appropriate confirmatory testing was not done [Mejia and Pollack, 1995]. Similar findings were noted in a survey of neurosurgeons and the criteria they employed in evaluating children with head injury for brain death [Chang et al., 2003]. Likewise, a survey of pediatric attending physicians and residents in the United States found a high percentage (39–58 percent) who could not define brain death correctly or recognize that brain death could be diagnosed without confirmatory testing in children older than 1 year of age [Harrison and Botkin, 1999].
A more recent study has also documented the wide variability in clinical practice for determining brain death [Mathur et al., 2008]. Of 142 of 277 children referred to OneLegacy who became organ donors, the number of brain-death examinations performed was 0 (4 patients), 2 (122 patients), 3 (14 patients), or 4 (2 patients). Recommended intervals between examinations were followed for 18 percent of patients greater than 1 year of age and for no younger patients. A mean of only 5.5 of 14 examination elements was completed by neurologists and pediatric intensivists, and 5.8 by neurosurgeons. No apnea testing was recorded in 60 percent of patients, and an inadequate increase in PaCO2 levels occurred in more than half the patients. EEG was performed as a confirmatory test in only 26 percent of patients, and cerebral blood flow (CBF) determination was performed in 74 percent. This study clearly demonstrated the wide variability in clinical practice for following the 1987 guidelines, and that documentation is incomplete for most patients.
Clinical Examination
Cerebral Unresponsivity
The neurologic evaluation for brain death is difficult in a comatose patient, particularly in infants and children. It is necessary to standardize the basal conditions under which the patient is examined. The patient should be normothermic, and pharmacologic agents capable of producing coma or neuromuscular blocking agents must be excluded [Kennedy and Kiloh, 1996; Tobin, 1996]. Assessment for cerebral unresponsivity, loss of cranial nerve function, and determination of apnea (tested with a hypercapneic stimulus) must be performed and documented. Confounding circumstances always increase the complexity of the evaluation. Cerebral unresponsivity or coma can be quantitated using the Glasgow Coma Scale score, which ranges from 3 (no response) to 15 (normal) using assigned scores for eye opening, motor activity, and vocalization (see Chapter 73) [Jennett and Bond, 1975]. The scale should be modified, substituting socialization for verbalization for infants and children who are too young to have developed speech. The predictive value of the duration of unresponsivity in infants and young children may be less trustworthy than in adults. In young children, recovery may occur when unresponsiveness has occurred for prolonged periods, and even when serious structural nervous system abnormalities are present [Booth et al., 2004; Haque et al., 2008; Mandel et al., 2002; Carter and Butt, 2005]. If the neurologic evaluation is uncertain or inconsistent in children younger than 1 year of age, supportive laboratory documentation, including EEG and cerebral blood flow determinations, should be considered.
Brainstem Examination
Cessation of brainstem reflexes is generally accepted as the hallmark of brain failure. The NINCDS reported, however, that 141 of 459 comatose patients who died retained one or more brainstem reflexes [NINCDS, 1980]. No single preserved brainstem reflex could discriminate the preservation of any other brainstem reflex. The combination of pupillary light response and oculocephalic and oculovestibular reflexes had the greatest specificity, but this combination included 4 percent of patients who retained other brainstem reflexes. Box 79-4 outlines the procedures used to assess the cold caloric (oculovestibular) response. The 2011 pediatric guidelines recommends that doing cold caloric testing is sufficient and that oculocephalic testing (head turning) is not necessary; they also state that, because some individuals could have spinal cord injury, such testing might pose additional risk [Nakagawa et al., 2011].
Premature infants of less than 32 weeks’ conceptual age do not have completely developed cranial nerve function. Clinicians examining these infants should be aware of the development of the different cranial nerve reflexes during gestation (Table 79-2). Newborns often present obstacles to examination. Ear canals are frequently small and may be plugged, pupils are small, adhesive tape obscures the face and extremities, and neuromuscular blocking agents alter the examination. The 2011 pediatric guidelines include recommendations for term infants from 37 weeks’ gestation. Recommendations for preterm infants younger than 37 weeks were not made because of insufficient evidence. Fortunately, brain death rarely occurs in preterm infants, so from a practical clinical standpoint, clinicians will likely not have to confront the issue of trying to diagnose brain death in this population.
Developmental Reflex | Gestational Age (Weeks) when Reflex is Elicitable |
---|---|
Suck, root, gag | 32–34 |
Auditory response | 30–32 |
Pupillary response to light | 30–32 |
Oculocephalic response | 28–32 |
Corneal response | 28–32 |
Moro response | 28–32 |
Grasp response (palmar) | 30–32 |
Alertness | 30–32 |
Apnea response to PaCO2 stimulus | 33 |
(Some data from Fanaroff et al. The respiratory system. In: Fanaroff A, Martin RJ, eds. Neonatal-perinatal medicine: Diseases of the fetus and newborn. St. Louis, Mosby, 1987.)
Number of examinations, examiners and observation periods
Number of Examinations and Examiners
The revised 2011 guidelines have tried to address issues related to the duration of time for which a pediatric patient should be observed before the official declaration of brain death, as well as the number of examinations needed and who should be doing the examination [Nakagawa et al., 2011]. The 1987 guidelines recommended observation periods between brain-death examinations based upon age and the results of neurodiagnostic testing [Report of Special Task Force, 1987]. Two examinations and EEGs separated by at least 48 hours were recommended for infants of 7 days to 2 months. Two examinations and EEGs separated by at least 24 hours were recommended for children of 2 months to 1 year. A repeat EEG was not necessary if a cerebral radionuclide scan or cerebral angiography demonstrated no flow or visualization of the cerebral arteries. For children older than 1 year, an observation period of 12 hours was recommended and ancillary testing was not required when an irreversible cause existed. The observation period in this age group could be decreased if there was documentation of electrocerebral silence or absent CBF. The general consensus was that the younger the child, the longer the waiting period should be, unless ancillary studies supported the clinical diagnosis of brain death; if so, the observation period could be shortened.
Whereas the recently revised 2011 guidelines for declaring brain death in adults (see Box 79-1) recommended only one examination, the revised pediatric guideline recommend continued performance of two examinations separated by an observation period. In addition, the adult guidelines also stated that, because recommendations as to the length of observation periods have varied extensively throughout the world and the United States, and because no detailed studies on serial examinations in adult patients who have been declared brain-dead have been done, there was insufficient evidence to determine the minimally acceptable observation period to ensure that neurologic functions have ceased irreversibly.
Duration of Observation Periods
A literature review of 171 children diagnosed as brain-dead found that 47 percent had ventilator support withdrawn, on average, 1.7 days after the diagnosis of brain death was made [Ashwal and Schneider, 1987]. Seventy-nine children (46 percent) in whom support was continued after declaration of brain death suffered a cardiac arrest an average of 22.7 days later. The remaining children died by an unknown mechanism (5 percent), or made an incomplete (1 percent) or complete recovery (0.5 percent). The age range of the children in this study included preterm and term neonates, and older infants and children up to age 18 years. These data and the reports of more recent studies [Ashwal and Schneider, 1987; Parker et al., 1995] suggest that there is likely no biological justification for using different durations of observation to diagnose brain death in infants greater than 1 month of age. In fact, there are no reports of children recovering neurologic function after meeting adult brain-death criteria based on neurologic examination findings [Ashwal, 2001]. Although some authors have reported apparent reversibility of brain death, further review of these cases reveals that these children would not have fulfilled brain-death criteria by currently accepted U.S. medical standards [Joffe et al., 2009].
Based on the above data, currently available literature, and clinical experience, the 2011 committee recommended that the observation period between examinations should be 24 hours for neonates (37 weeks up to 30 days), and 12 hours for infants and children (>30 days to 18 years). The first examination determines that the child has met neurologic examination criteria for brain death. The second examination confirms brain death, based on an unchanged and irreversible condition. Timing of the first clinical brain-death examination, reduction of the duration of the observation period, and use of ancillary studies are discussed in separate sections of this guideline [Nakagawa et al., 2011].
Apnea Testing
Documentation of apnea, under controlled conditions, is the most important determination in clinically evaluating brain death. Virtually all protocols recommend a period of unassisted ventilation, allowing hypercapnia maximally to stimulate the respiratory effort. The first formal observation period (3 minutes) was recommended by the Ad Hoc Harvard Medical School Faculty [Beecher, 1968]. The Conference of Royal Colleges and Faculties suggested administering a mixture of 5 percent carbon dioxide and 95 percent oxygen for 5 minutes while withholding respirator support, and then supplying 100 percent tracheal oxygen [Conference, 1976]. The President’s Commission recommended 100 percent oxygen ventilation for 10 minutes, followed by passive 100 percent tracheal oxygen for a period long enough to achieve a PCO2 of at least 60 mmHg [President’s Commission, 1981].
The normal physiologic apneic threshold (minimum PCO2 at which respiration begins) depends on many factors, and can be altered by anesthetic agents, narcotics, sedatives, and certain disease states. Schafer and Caronna described three patients, suspected of being brain-dead, who developed spontaneous respiration at PCO2 levels between 45 mmHg and 50 mmHg, which suggested a maximal end point of 60 mmHg [Schafer and Caronna, 1978]. Ropper and co-workers studied seven brain-dead patients and reported a PCO2 of 44 mmHg as a maximal excitatory end point [Ropper et al., 1981], but this has not been studied in children.