Figure 6.1 Fatal abuse in an eight-month-old with fractures of at least three different ages. (See same patient in Fig. 2.9.) A, AP portable supine radiograph obtained at the time of resuscitation shows fractures of the right sixth-to-ninth ribs without frank callus formation (arrows). There is a healing fracture of the right tenth rib (circle). There is a right tension pneumothorax. The child was pronounced dead and multiple fractures were noted on SS, including over 24 rib fractures. B, Frontal specimen radiograph shows a fracture of the left eighth rib (black arrows) with subtle SPNBF (white arrow). C, Corresponding axial specimen radiograph shows disruption of the inner rib cortex (black arrow). There is unilaminar (single-layered) SPNBF (white arrows). Note early woven bone, densest at the site of cortical disruption (*). D, Frontal specimen radiograph shows fractures of the right tenth (black arrows) and eleventh (curved arrow) ribs. Note that the relatively solid SPNBF (thin white arrows) blends with mature hard callus with trabecular matrix surrounding the fracture site (thick white arrows). The eleventh rib fracture shows no healing. E, Axial specimen radiograph of the right tenth rib shows the mature fracture callus (white arrows). There has been a refracture (confirmed pathologically) through the callus (black arrows) with displacement (*). The mother’s boyfriend pled guilty to beating her son to death and was sentenced to 14–16 years in prison.
Although most radiologists make assessments of fracture healing with accidental fractures on a daily basis, the evidence base for dating fractures, particularly in the abusive setting, remains incomplete (3, 5–7, 10, 11). This is remarkable when one considers the important implications of these radiologic assessments.
This chapter attempts to provide some guidelines for dating fractures in infants and children in whom the diagnosis of physical maltreatment is considered. Special attention is given to infants, who are most often affected by inflicted injury and are at greatest risk for a poor outcome (12). There are abundant examples of abusive appendicular and axial skeletal injuries in various stages shown elsewhere in this text (see Chapters 2–5, 17, and 21). The distinctive patterns of healing of the classic metaphyseal lesion (CML) are discussed in depth in Chapters 2–4. The difficulties of dating cranial and spinal injuries are covered in Chapters 17 and 21 respectively. The principles of dating skeletal injuries in this chapter are most applicable to the tubular bones. The foundations of this current chapter were set down in the earlier editions of this text (13, 14) by one of the current author’s (P. K. K.) esteemed former colleagues John F. O’Connor, an early pioneer in pediatric radiology, and Jonathon Cohen, an orthopedic surgeon with special expertise in histopathology. The current chapter builds on these observations with the incorporation of the recent literature and current concepts of fracture healing.
Pathophysiology
There are several important distinctions between fractures from abuse and those sustained through accidental trauma. An appreciation of the differences between these types of injuries and the way they come to medical attention is important in understanding the pathophysiology of and expected timetable for fracture healing in cases of abuse. A first important consideration is the nature of the clinical history available. In the setting of accidental trauma, a clear history of injury occurring at a specific time is nearly always provided, and this typically correlates well with findings noted on imaging studies. In cases of abuse, clinical history may be absent or misleading. This complicates interpretation of imaging findings and makes fracture dating particularly challenging.
A second consideration is the age group most commonly affected by inflicted injury. Inflicted skeletal injuries are more common in younger children, and are most frequent in infants (15–18). Fractures of abuse are unusual in children older than four years. There are inherent differences in the composition of the infant skeleton that dictate observed patterns of radiologic and histologic healing.
A third important consideration is the concept of repetitive injury. Some abusive fractures should be regarded not as single injuries, but rather as a sequence of traumatic events. This applies even when there has been a single abusive traumatic episode. There is often considerable delay between the initial injury and the diagnosis/treatment. During this delay of days to weeks, the lack of immobilization predisposes to repetitive microtrauma at the fracture site. When there have been multiple episodes of abuse, secondary abusive trauma to original fracture sites may substantially affect the healing process and complicate dating assessments. Repeated episodes of abusive trauma may not only disrupt whatever repair has taken place, but also provide a stimulus for a new cycle of osseous repair.
The customary approach to the study of the healing process of inflicted injuries is to draw upon the existing knowledge base of the biomechanics, imaging, and histopathology of accidental injury. While this is somewhat applicable, the essential differences that pertain to abusive fractures must be considered. Each of these deserves separate attention.
Clinical history
Direct communication between the radiologist and the clinician eliciting the clinical history and examining the patient is vital in cases of suspected abuse. Consultation between the radiologist and clinician, often a member of the Child Protection Team (CPT), should occur before a formal interpretation of the imaging study is rendered. Details surrounding history and physical examination findings may inform the radiologist as he or she attempts to accurately define fracture age ranges and place them in clinical context.
Patient age
Based upon experience with accidental fractures of known age, as well as patterns of healing following the recognition of abusive injuries, it is evident that fractures in infants generally progress more rapidly through all radiographic phases of healing than injuries in older children. The appearance of SPNBF, the elaboration of callus, solidification of callus, and bony remodeling are usually all seen earlier in young infants. The general precept has been that with occasional exceptions SPNBF will occur in infants and young children at about 7–10 days after injury, but the usual time interval in older children and adults tends to be later at 10–14 days (Figs. 6.1, 6.2).
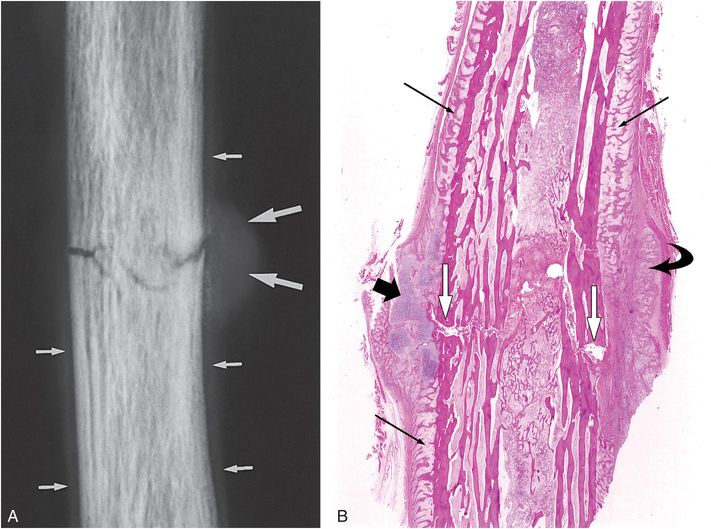
Figure 6.2 Early healing radial shaft fracture. A, Specimen radiograph of the distal radius in a 20-month-old infant who died unexpectedly demonstrates a transverse diaphyseal fracture. The fracture line is relatively sharply defined. There is early diffuse SPNBF (small arrows). Increased radiodensity projecting into the soft tissues adjacent to the fracture margin is noted, consistent with early (soft) callus formation (large arrows). B, A corresponding low power histologic section shows the fracture line (white arrows). Note that the fracture margins are relatively distinct. There is diffuse SPNBF (long black arrows) and fibrous, cartilaginous (short black arrow), and osseous (curved arrow) callus.
Histologists are also aware of differences between infants and children with respect to tissue changes after fracture. The fact that infant fractures evolve more rapidly through all histologic phases of healing when compared to older children is particularly notable with respect to the production of fibrocartilaginous matrix in the callus. Many variables will affect other earlier elements of histologic healing, such as the production of hemorrhage and inflammatory change at the injury site. These variables include the severity of injury(s), the degree of displacement of fracture fragments, and the effectiveness of immobilization. The multiplicity of variables has caused histologists to rely substantially on experimental evidence from animal studies rather than evidence from studies on human specimens. This evidence as it relates to the study of differences between infants and older individuals is not only limited, but is also inherently qualified by assumptions based on presumed parallels between species.
One source of reliable information that is relevant in the study of abuse fracture healing is the neonatal obstetric injury (see Chapter 11). With this fracture, the nature of the trauma and the timing of the injury are known. Two of the variables affecting abuse-related fractures, lack of immobilization and repetitive trauma, do not generally pertain to these obstetric injuries. In this setting, sequential radiologic examinations may lend valuable information regarding the chronology of repair. It should be noted that inflicted injuries, even in the neonate, might differ from obstetric injuries due to variables already mentioned. In contrast to the gross humeral and femoral shaft fractures and epiphyseal separations occurring with obstetric injury, extremity fractures in young, seriously abused infants are often CMLs, injuries that are generally subtle and associated with modest radiologic alterations, even in the setting of healing (see Chapter 2).
Changes in the developing skeleton that occur with age are important to consider (see Chapter 1). The skeleton of a neonate differs considerably from that of an infant aged six months or more (19, 20). The sequences of enchondral ossification in the neonate are much more irregular (21). Bone is cancellous in the neonate, and porosity decreases markedly even in the early stages of infancy. Eventually, compact bone with haversian systems will predominate. The normal changes in bony composition that occur with growth will dictate the radiologic and histologic appearance of the fracture in the age group under review.
An additional phenomenon of the developing skeleton relevant to the dating of inflicted injury is the presence of growth recovery (arrest) lines in the bones of an infant or child that has been affected by a serious illness, severe trauma, or malnourishment (22). Although highly nonspecific for any single etiologic agent, the presence of growth recovery lines in an abused child may contribute evidence regarding the chronicity of abuse, and perhaps the time interval since the injury.
Repetitive injuries
The term repetitive injury in this context is used in two scenarios: (1) two or more distinct inflicted injuries to the same area of a bone; and (2) fractures which sustain repetitive injury (microtrauma) due to lack of immobilization, but without repeat assault to the same site (Fig. 6.3). It is usually difficult to make a distinction between these two types of repetitive injury on radiographic grounds. Although passage of a fracture line through mature callus/SPNBF may suggest a second discrete injury, extension of fracture lucency through organized callus is a regular occurrence with accidental fractures that continue to move despite efforts of immobilization. A grossly displaced fracture through organized callus is more likely to be due to a second injury (Fig. 6.1E). It is not unusual to see acute and early organizing hemorrhage pathologically in older fractures, and in the absence of gross morphologic features suggesting a second injury, these findings are usually attributable to motion and microtrauma of an immobilized fracture.
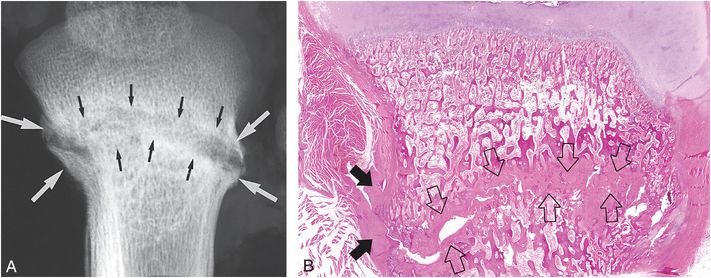
Figure 6.3 Advanced healing (chronic) fracture with evidence of repetitive injury in a 14-month-old infant who died from a fatal head injury. There was no history of a previous fracture, but a proximal tibial fracture was encountered at postmortem SS. A, AP specimen radiograph of the right proximal tibia demonstrates an oblique fracture through the proximal tibial metadiaphysis. There is sclerosis along the fracture margins (black arrows) and thick hard callus (white arrows), which buttresses the cortical surfaces. Note the widening of the fracture line. B, A corresponding low power histologic section shows extensive mineralized matrix and new bone (open arrows) along the fracture site, as well as the endosteal and periosteal (solid arrows) surfaces of the fracture. Despite the evidence of advanced healing, there is no gross osseous continuity across the fracture site. These findings suggest repetitive injury to this neglected fracture.
The timing of repetitive injuries and the severity of each incident may be widely variable. If an infant sustains an inflicted fracture of the femur and the process is repeated within the days immediately following the injury, the hematoma around the fracture margins would be expected to amplify considerably. However, if no significant displacement of fracture fragments occurred with either incident, the repair sequence that followed would be similar to that seen with a single injury. In contrast, if the repetitive trauma were inflicted one week after the first episode, there would be more hemorrhage, in addition to tearing of newly formed thin-walled vessels and injury to the collagenous fibers that had stabilized the fracture fragments. There would also be additional devitalization of soft tissue and bone at the fracture site, including some of the cells involved in inflammation and repair processes.
An understanding of the complex pathophysiologic processes engendered by repetitive injuries must play a role in the assessment of an abuse-related fracture by the clinician, radiologist, and pathologist. It should be emphasized that owing to lack of immobilization, all long bone fractures from abuse are subject to repetitive trauma. Rib fractures and fractures about the shoulder girdle are subject to repetitive injury not only prior to detection, but also following diagnosis, since these injuries are usually not well immobilized.
Chronic and remote fractures
It is often crucial for forensic reasons to estimate when chronic or remote fractures may have occurred. Radiologically, an older chronic fracture will show callus at the fracture site, and possibly residual SPNBF separated from the bony cortex. SPNBF will incorporate into the underlying cortex in 1–3 months. Eventually the remodeling process will restore the region of repair to a more normal appearance; radiodense areas will recede and the bony architecture will approach or return to its original morphology.
The course of remodeling will vary with patient age and the type/severity of injury. At one end of the spectrum is a single instance of injury in a neonate with a long bone fracture involving the cancellous metaphysis with no significant displacement of fracture fragments. After two months, there will generally be no radiologic sign of fracture except for variable sclerosis at a site further from the physis than the original injury – a difference that reflects the amount of longitudinal growth that has occurred since the fracture. At the other end of the spectrum, a displaced and angulated fracture through the cortex of a long bone in an older child may never exhibit enough remodeling to result in the correction of deformity. A Salter–Harris (SH) injury (see below) at any age may result in significant and persistent deformity due to a physeal growth disturbance.
Histologic phases of fracture healing
Induction
The stage of induction is defined as the time interval between the instant of injury and the first appearance of new bone at the fracture site. The duration of the stage of induction for long bone fractures varies according to the site of injury, the severity of the trauma, and the patient age. The stage of induction involves both a destructive phase, with the removal of hemorrhage and damaged tissue, and a constructive phase, with the formation of osteoid and bone. These phases overlap spatially as well as chronologically. With injury, there is disruption of blood vessels in bone and soft tissue, with resulting hemorrhage at the fracture site. Hemorrhage may recur whenever fracture fragments are moved. Thus, it may occur as a single episode over several minutes immediately following an injury, or may be cyclic in nature with recurrence over several days following the initial fracture. As active hemorrhage ceases, a hematoma is formed.
Next, the processes of inflammation and resorption of nonviable tissues are initiated. Inflammation begins soon after injury occurs and involves widespread soft tissue edema at the fracture site. The duration of acute inflammation is typically a few days, and is considerably shorter in infants and children than in adults. As the hematoma and edema stabilize the soft tissues, the margins of the fracture become necrotic and the soft tissues become devitalized. Resorption of these tissues occurs in preparation for the constructive phase of repair. Ongoing inflammation and removal of nonviable tissues lasts for several days at a minimum, but more often up to 3–4 weeks.
The most important variable that governs the duration of the stage of induction is the development of a significant amount of granulation tissue and its metamorphosis to produce osteoid. Capillaries, precursor cells, and strands of collagen must be formed in the damaged tissue before the definitive elements of osteoid and bone are produced. Notably, the ingrowth of new capillaries is required throughout the induction phase for the transport of inflammatory cells, precursor cells, fluid, macrophages, and osteoclasts to accomplish the work of repair. Histologically, osteoclastic activity is usually detected within 4–7 days of the original injury.
Subperiosteal new bone formation
Histologic evidence of SPNBF is present before radiographic changes are apparent and can be seen as early as several days following injury in young infants – somewhat later in older children and adults (Fig. 6.2B). The periosteum consists of two layers, an outer fibrous layer and an inner osteogenic (cambium) layer adjacent to the bony cortex (see Chapter 1). In the setting of fracture, the periosteum is stripped away from the underlying cortical bone and contained hemorrhage dissects along the bone beneath the osteogenic layer of the periosteum. Hemorrhage is ultimately replaced by fibrovascular tissue. There is active proliferation of osteoblasts within the osteogenic layer of the periosteum and new subperiosteal woven bone is deposited with increasing laminated trabecular density over the ensuing weeks.
Callus
In the early stages of callus formation, nearly all of the hematoma and much of the inflammatory tissue at the fracture site are resorbed. Osteoclasts remain active in the removal of necrotic trabeculae and bony cortex. Removed tissue is gradually replaced by fiber bone, and subsequently converted to woven bone. Callus develops around the ends of a fractured bone by cellular organization within the fracture hematoma, and ultimately unites the fracture fragments. Fibrovascular tissue replaces the hematoma with collagen fibers and matrix elements, which become mineralized and form the woven bone of the primary callus. This process begins near the fracture margins and proceeds centrifugally away from the injury site. Cartilage formation occurs predominantly at the periphery of the callus, and conversion to bone proceeds through enchondral ossification.
The time interval from injury to consolidation of the callus is highly variable based on age. In an adult, the average elapsed time from injury to consolidation in a single well-immobilized simple long bone fracture is 2–3 months (19, 20, 23). In children, and particularly in infants, this process is considerably faster. Salter noted that fractures of the femoral shaft might serve as an example of this phenomenon. A femoral shaft fracture occurring at birth is typically united by three weeks. A comparable fracture at the age of 8 years will be united at 8 weeks, and at the age of 12 years, it will be united at 12 weeks. From the age of 20 years on, this process will take approximately 20 weeks (24). The duration of time to callus consolidation is affected by other previously noted variables occurring with abusive injuries including the lack of immobilization of fracture fragments and a higher likelihood of repetitive injury.
Remodeling
Woven bone is gradually converted to lamellar bone and deformity is corrected with smoothing of callus surrounding the fracture. Single-layered SPNBF becomes solid/multilayered and incorporated into the cortex. When the medullary cavity is reconstituted and the cortex approximates the configuration of the adjacent uninjured bone or the contralateral counterpart, remodeling is complete. Complete remodeling may occur even in the setting of significant displacement and/or angulation of fracture fragments. This may take place over a period as long as one to two years following the initial injury. Some authors argue that remodeling may occur throughout the period of growth and may even continue beyond skeletal maturity (19, 21, 23).
Physeal injury
Available knowledge on the histology of healing physeal injuries is primarily derived from experimental studies or autopsy material in children who have sustained trauma of known cause (25–31). The response to physeal injury is much quicker than that to fractures of cancellous or cortical bone. Physeal injuries as classically described usually occur through the zone of cartilage proliferation, and often involve the immediately subjacent metaphyseal bone, constituting SH type II injuries (see Chapter 2). Thus, physeal fractures heal through the process of enchondral ossification. When the fracture occurs through the zone of resting or proliferative cartilage, there is minimal hematoma, which is rapidly replaced by granulation tissue. Uninjured cartilage cells proliferate, with a relatively rapid increase in the number of chondrocytes per column. This results in widening of the physis. Resorption of devitalized tissue occurs as in other locations. As healing occurs, there is gradual resumption of normal enchondral ossification. The conversion of cartilage to bone occurs simultaneously with the ossification of fibrous callus. Provisional calcification and invasion of cartilage by metaphyseal vessels proceed normally.
With the classically described pattern of physeal injury, the periosteum is easily torn on one side and stripped from the metaphysis and diaphysis on the contralateral side. The healing process produces SPNBF of variable thickness and extent around the metaphyseal cortex, often extending to the metadiaphysis. SH type III and IV injuries are rarely seen in the setting of abuse.
With physeal injury, there is the possibility of disordered physeal growth and in some instances a bony physeal bar. This can result in uniform or asymmetric growth arrest and related deformity. Since most inflicted skeletal injuries spare the physis, this complication is fortunately relatively uncommon in the abusive setting.
Radiographic phases and dating of fractures
To date, there has been little scientific data available to guide radiologists in fracture dating in the setting of suspected abuse, and radiologists have traditionally relied on personal experience and conventional wisdom. While some scientific studies have been published on fracture dating in general, prior reports have shortcomings that limit the applicability of data to cases of abuse (3, 4, 6, 10, 32–34). Inflicted skeletal injuries are more common in younger age groups and most frequent in infants (15–18). Previous works have focused on patterns of fracture healing in older children, rather than the more relevant infant population (4, 10, 34). The timetable of fracture healing is known to be quite different in these age groups. Some studies have examined healing patterns in fractures that are immobilized (3, 4, 10, 33, 34). This treatment renders inferences drawn from these fractures less generalizable to cases of child abuse, since inflicted injuries are often initially untreated due to delay in diagnosis. In some studies, fractures were evaluated through cast material, a clear limitation to detailed evaluation of healing patterns (3, 4, 10, 33, 34). Other studies have focused exclusively on later stages of fracture healing such as callus formation, excluding earlier SPNBF from assessments (34). A reliable timetable outlining patterns of fracture healing should ideally include expected patterns of development of SPNBF as well as callus.
The authors’ group has recently published a study that builds on prior work in this area, but is unique in some respects (7). We studied birth-related clavicular fractures in a large sample of otherwise healthy infants between 0 and 3 months of age. The birth-related clavicular fracture was deemed an appealing surrogate for inflicted injury, as fracture age at the time of injury is known with reasonable certainty. This model affords the study of the patterns of fracture healing in young infants, the population at greatest risk for a poor outcome. Clavicular morphology is similar to that of other tubular bones, and therefore healing patterns may be generalizable to fractures involving the long bones and ribs in abused infants. Also, infant clavicular fractures are generally not immobilized (35), and therefore the spectrum of fracture healing may be similar to that of abusive fractures in which treatment is delayed and repetitive injury may occur. In the study we sought to define predictable patterns of fracture healing in infants to establish an evidence base that could be applied in the setting of suspected infant abuse.
In the following discussion we attempt to distill the published evidence on fracture healing and meld it with the clinical experience of the current (P. K. K., M. W.), as well as the previous authors (J. F. O., J. C.) of this chapter, and we aim to provide reasonable guidelines for assigning ages to abusive fractures in radiologic practice.
The soft tissues
The first principle in identifying an injury to the skeleton is based on knowledge of the normal relationships of the fat planes between muscles in aggregates and adjacent to bony or articular structures. Injury to the soft tissues, with or without bony injury, is followed by edema and hemorrhage in the soft tissues that usually displace or obliterate the normal relative radiolucency of the deep and/or superficial soft tissue planes. In the absence of associated osseous or articular injury, these changes are usually not extensive and typically resolve completely within a few days following the injury. When there is associated injury to bone or cartilage, the severity of swelling, its tendency to involve more of the surrounding soft tissues, and the delay in return of normal imaging of the soft tissue planes may suggest occult skeletal injury rather than isolated soft tissue contusion.
Subperiosteal new bone formation
Radiographically, subperiosteal new bone may appear as a hazy cortical margin or a thin layer of bone separated from the original cortex by a discrete lucent interval (Fig. 6.1B,C). In a large population of young infants, our group noted that SPNBF is highly unlikely in fractures less than 7 days old, and is most often present by 10 days (Fig. 6.4). Rarely SPNBF can be seen in less than a week in neonates sustaining birth injuries. Subperiosteal new bone thickness increases with fracture age, and its character changes from single-layered to solid/multilayered (Fig. 6.5).
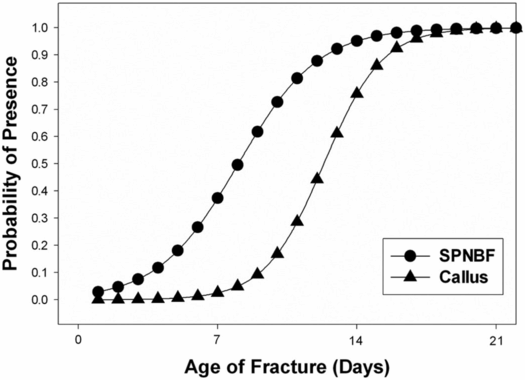
Figure 6.4 Logistic regression demonstrating the probability of presence of SPNBF and callus with fracture age. (With permission from Walters MM, Forbes P, Buonomo C, Kleinman PK. Healing patterns of clavicular birth injuries as a guide to fracture dating in cases of possible infant abuse. Pediatr Radiol. 2014;44(10):1224–9.)