Chapter 181 Craniovertebral Abnormalities and Their Neurosurgical Management
Craniovertebral junction abnormalities can be developmental, genetic, or acquired in origin.1–3 To effectively treat these disorders when they are symptomatic, the clinician must have a knowledge of the embryology and the functional anatomy of the area. A myriad of abnormal neurologic findings may be present that are secondary to compression or ischemia of neural tissue. The surgical management of these disorders depends on precise identification of the underlying pathophysiologic condition as determined by appropriate radiologic studies. The operative treatment includes anterior, lateral, and posterior approaches to the craniovertebral junction with and without bony fusion. Our database and experience with more than 6000 patients who had symptomatic craniovertebral abnormalities is the basis for management of these complex disorders. The disorders can be classified as listed in Table 181-1.
TABLE 181-1 Classification of Craniovertebral Junction Abnormalities
Developmental Anomalies of the Craniovertebral Junction |
Malformations of Occipital Bone |
Clivus segmentations |
Remnants around foramen magnum |
Basilar invagination |
Condylar hypoplasia |
Abnormal occipitoatlantal alignment |
Malformations of Atlas |
Failure of atlas segmentation from occiput (assimilation) |
Atlantoaxial fusion |
Aplasia of atlas arches |
Malformations of Axis |
Irregular atlantoaxial segmentation |
Dens dysplasias |
Ossiculum terminale persistens |
Os odontoideum |
Hypoplasia-aplasia |
Segmentation failure of C2-C3 |
Neural Dysgenesis |
Genetic and Acquired Abnormalities of Craniovertebral Junction |
Abnormalities at the Foramen Magnum |
Basilar impression (secondary basilar invagination) |
Foraminal stenosis |
Traumatic occipitoatlantal dislocation |
Os odontoideum |
Tumors |
Atlantoaxial Instability |
Errors of metabolism |
Down’s syndrome |
Infections |
Inflammatory |
Traumatic atlantoaxial dislocations |
Miscellaneous |
History
Subsequent to the first description of spontaneous atlantoaxial dislocation in 1830 by Bell,4 lesions affecting the cervicomedullary junction have emerged from being medical curiosities to being conditions that can be effectively managed. Except for acute dislocations, the treatment of occipitoatlantoaxial joint pathologic entities was marked with failure before the era of skeletal traction. Subsequently, it was apparent that most acute and chronic dislocations could be reduced even years after the initial injury.
The early operative procedures were posterior decompression of the cervicomedullary junction with and without fusion for stabilization. Posterior decompression in patients with irreducible ventral compression of neural tissue at the craniovertebral area is often associated with a high operative risk and a low incidence of improvement; most patients are unchanged or have increased neurologic deficit.5
More recently, transpalatine-transoral1,2,6–11 and extrapharyngeal12–15 ventral operations were described for fractures, tumors,16 congenital abnormalities, infection, and inflammatory conditions at the craniocervical junction.17,18 Stabilization of the atlantoaxial occipital joints usually has been performed by fusing the spinal column posteriorly. No single anterior or posterior surgical procedure can be used for all patients with craniovertebral abnormalities; the operation or combination of operations must be selected on an individual basis to correct the pathologic process responsible for the neurologic deficit.1–319
Embryology and Anatomy
By definition, the craniovertebral junction includes the basiocciput, the foramen magnum, the atlas, and the axis vertebra. The occipital bone is formed by fusion of four sclerotomes. The proatlas is the most caudal of the sclerotomes and loses its identity in humans. The neural arch of the primitive proatlas divides into ventral rostral and dorsal caudal segments.20 The ventral rostral segment gives rise to the occipital condyles and the alar and cruciate ligaments. The dorsal caudal segment forms the posterior arch of the atlas and the lateral atlantal masses to help form its rostral articular facets. If the posterior segment of the proatlas remains separate, the atlas has bipartite cranial articular facets, a rare anomaly that can result in horizontal instability of this joint. Finally, the proatlas also gives rise to the apex of the dens.21 Synchondrosial growth between the four occipital sclerotomes occurs until age 18 years. During this time, the clivus elongates and the volume of the posterior fossa expands. Insufficient volume of the posterior fossa as a result of insufficient growth results in tonsillar ectopia as the hindbrain herniates through the foramen magnum. Therefore, extended follow-up is critical for children with craniocervical abnormalities while growth continues into adolescence.22
An occipital vertebra is a bony structure that is separate from the foramen magnum and incorporates the occipital condyles. The anterior arch may be partially or completely fused to the anterior margin of the foramen magnum, and the transverse process, if present, does not have a foramen for the vertebral artery. In contrast, an atlanto-occipital fusion is characterized by ankylosis between the atlas and the skull base, usually with persistence of the normal joints. 22
The atlas is derived from the first cervical sclerotome as well as the proatlas. The body of the atlas as such disappears and gives origin to the dens. The anterior arch of the atlas has one center of ossification, although at times two centers may be present. The atlas has two robust lateral masses situated at the anterolateral portion of the ring. Lateral to these is located the transverse foramen, which transmits the vertebral artery. The artery then courses within a groove along the posterior arch parallel to the first spinal nerve before piercing the dura. The lateral mass extends below the posterior arch to contact the axis. Normally, the ring of the atlas becomes ossified within the first 3 to 4 years of life. If the atlas does not fuse and a persistent bifid anterior and posterior arch persists, a condition similar to a Jefferson fracture can occur with lateral displacement of the lateral masses. In children younger than 3 years, use of a custom molded brace will allow fusion of the atlas, and stability can be restored with conservative management alone. If the atlas remains bifid after the age of 4 years with abnormal motion, arthrodesis is necessary.22
Failure of the segmentation between the proatlas and the first cervical sclerotome results in atlas assimilation. Often identified in patients with Klippel-Feil syndrome, this abnormality is commonly associated with segmentation failure at C2–3 as well, which places great stress on the atlantoaxial interface. In a review of 30 patients with occipitalization of the atlas, 57% were found to have atlantoaxial instability on dynamic imaging and 47% had an associated C2–3 fusion.23 Initially, a pannus develops around the odontoid. As the instability progresses, cranial settling occurs, causing the odontoid to migrate through the foramen magnum, resulting in basilar invagination. In the early stages, the lesion is still reducible. However, in severe cases, bone remodeling occurs, resulting in a shortened horizontal clivus and a flattened occiput. The posterior fossa volume is reduced and an acquired hindbrain herniation syndrome develops.22
The axis is developed from four primary ossification centers. The facets and posterior arch of the axis all originate from the second cervical sclerotome. The dens originates from three different sclerotomes. As discussed, the apex arises from the proatlas. The C1 sclerotome gives rise to the body of the odontoid process, and the inferior portion of the C2 body is formed by the second cervical sclerotome. At birth, a cartilaginous band persists between the odontoid and the inferior body of the axis, termed the neural central synchondrosis. By age 8 years, the neural central synchondrosis is no longer seen. The tip of the dens is not visible at birth and first appears at age 3 years as a separate ossification center. Typically, the odontoid becomes fully ossified by age 12 years.21 The complex embryologic origin of the odontoid process explains several important abnormalities resulting from dysgenesis of the dens. Failure of the proatlas and the dens to fuse results in ossiculum terminale. Os odontoideum occurs as a result of trauma between the ages of 1 and 4 years or in nontraumatic cases associated with Down’s24 and Morquio’s syndrome and is actually a fracture separation of the dens tip. Hypoplasia and agenesis of the dens is the result of developmental failure of the distal ossification centers. The common pathophysiology that produces neurologic deficit with agenesis or hypoplasia of the dens is instability between the first and second cervical vertebrae that results from ligamentous incompetence.22
To accurately assess the stability of the craniocervical junction, a working knowledge of the complex ligamentous anatomy is required and is demonstrated in Figure 181-1. The dens is approximated to the anterior arch of the atlas by the transverse ligament, which is anchored to the tubercle on the mesial aspect of each lateral mass of the atlas. This ligament is responsible for the stability of the atlantoaxial joint. The axis is connected to the occiput by (1) the alar ligaments that course obliquely upward from the posterior lateral surface of the dens to the anterior and medial surface of the occipital condyles; (2) the apical dens ligament, which continues from the medial aspect of the foramen magnum to the tip of the dens; (3) the tectorial membrane (an extension of the deep layer of the posterior longitudinal ligament); and (4) the cruciate ligament, which consists of the transverse ligament plus triangular ascending and descending slips to the anterior rim of the foramen magnum and the axis, respectively (see Fig. 181-1A, B).25 Finally, the tectorial membrane is the rostral extension of the posterior longitudinal ligament and courses posterior to the cruciate ligament and inserts into the occiput. The integrity of the ligamentous structures, particularly the cruciate ligament, can be clearly identified on high-resolution magnetic resonance imaging (MRI) and helps determine the stability of the craniocervical junction.
The occipitoatlantoaxial joints are complex, both anatomically and kinematically.26,27 Anatomically, two occipitoatlantal articulations exist. There are four atlantoaxial joints, with a common synovial lining between the dens and the anterior arch of the atlas, the dens, and the transverse ligament, and between the four lateral masses. Flexion-extension occurs at both the occipitoatlantal and atlantoaxial articulations and accounts for approximately 25% of the flexion and extension movement in the neck. During flexion, the cruciate ligament limits the drift of the odontoid away from the anterior arch of C1 (predental space) to less than 5 mm in children and 3 mm in adults. If the cruciate ligament is disrupted, the alar and apical ligaments ultimately fail and instability ensues. The atlantoaxial joints are responsible for the largest degree of rotation in the cervical spine. Total rotation of the neck is up to 90 degrees, with up to 30 degrees being accounted for at C1–2. With rotation beyond 30 degrees at C1–2, stretching and compression of the contralateral vertebral artery can occur.25
The development of the neck musculature is inadequate to supplement joint stability until the age of 8 years. Before this age, laxity of the ligamentous tissue permits excessive movement of the occipitoatlantoaxial articulations.28,29 Forward gliding of the skull in relation to the spine occurs if hypoplastic occipital condyles are present. This is the mechanism for the development of neurologic deficit in children who have spondyloepiphyseal dysplasia, Conradi’s syndrome, or Morquio’s syndrome. Further, several disorders are associated with even greater ligamentous laxity such as Down’s syndrome,24 juvenile rheumatoid arthritis, and Klippel-Feil syndrome, which place these children at risk for severe injuries after seemingly insignificant trauma.
The lymphatic drainage to the occipitoatlantoaxial joints is through retropharyngeal glands to the deep cervical lymphatic chain. In children, nasopharyngeal infections can cause an inflammatory reaction to the synovial joints of the craniovertebral junction and weaken the ligaments within the craniocervical junction. Because the neck musculature is not fully developed and is unable to compensate for the ligamentous laxity, C1–2 subluxation can develop and has been referred to as Grisel’s syndrome.30
In the osseoligamentous destruction caused by rheumatoid arthritis, the synovial bursa and associated ligaments that surround the odontoid process are damaged and result in loss of stability.31 Subluxation can occur secondary to the atlas moving anteriorly on the axis (caused by insufficiency of the cruciate ligament or fracture of the odontoid process), secondary to the atlas moving posteriorly on the axis (from erosion or fracture of the odontoid process), or by telescoping of the skull on the axis (from destruction of the axis lateral masses or apophyseal joints).31 Chronic subluxation often results in ligamentous hypertrophy and the accumulation of granulation tissue behind the odontoid process from the hypertrophied soft tissue. Even though normal bone alignment is present on roentgenograms, there may be ventral compression of the cervicomedullary junction by soft tissue.
Signs and Symptoms
Craniocervical junction abnormalities produce a myriad of symptoms and signs, including an abnormal general physical appearance; myelopathy; brain stem, cranial nerve, and cervical root dysfunction; vascular insufficiency; or any combination of these.1–3,32,33 The head may be tilted to one side as a result of rotatory subluxation of the atlas on the axis. Patients with Klippel-Feil syndrome classically present with a triad of findings including limited neck movement, a short neck, and a low posterior hairline. In addition, patients with various types of dwarfism, including spondyloepiphyseal dysplasia and achondroplasia, have an increased incidence of craniovertebral disorders.34,35
Neck and occipital pain is the most common complaint, occurring in 85% of affected children. The pain is classically described as originating in the suboccipital area and extending up toward the vertex in the sensory distribution of the greater occipital nerve. The dysesthesias are from irritation of the second cervical nerve as it traverses the lateral atlantoaxial joint capsule. In those with basilar invagination, a “basilar migraine” is a common complaint occurring in up to 25% of these patients. Fortunately, these headaches often regress after surgery.36
Myelopathy was the most common neurologic deficit in our series, occurring in 98% of the patients. The initial symptoms, such as lack of physical endurance, may be subtle, particularly in younger patients. The severity of myelopathy is variable and can manifest as different degrees of weakness in the upper or lower extremities. False localizing signs were common, and motor deficits included monoparesis, hemiparesis, paraparesis, tetraparesis, and quadriparesis. A myelopathy mimicking the central cord syndrome was often present in patients with basilar invagination.36 The pathophysiology of motor myelopathy has been attributed to repetitive trauma on the pyramidal tracts secondary to chronic compression.37 In addition, instability at the craniocervical junction can lead to repeated compression to the anterior spinal artery, leading to spasm and occlusion. The false localizing signs have been attributed to stagnant hypoxia of the cervical spinal cord from venous stasis.38
Cranial nerve deficits are also often observed. Loss of the gag reflex, unilaterally or bilaterally, is often observed and can lead to aspiration pneumonia. Respiratory arrest, sleep apnea, and snoring were associated with both anterior and posterior compression of the cervicomedullary junction,39 and they often resolve after decompression. Basilar invagination can also lead to internuclear ophthalmoplegia. Nystagmus to lateral gaze is not uncommon, and downbeat nystagmus is associated with hindbrain herniation syndrome.40 Interestingly, hearing loss, tinnitus, or both, was observed in 23% of patients and was particularly common in those with Klippel-Feil syndrome.
Diagnostic Investigations
Several reference lines are used to evaluate plain roentgenograms in the assessment of the cervicobasilar relationships.41 McRae’s line measures the sagittal diameter of the foramen magnum from its anterior margin to its posterior margin (average, 35 mm). Towne’s projection is useful for determining the transverse diameter of the foramen magnum (35 mm ± 4 mm). Chamberlain’s line is a diagonal from the hard palate to the posterior margin of the foramen magnum (Fig. 181-2). The odontoid process should not extend more than one third of its length above this line or no more than 5 mm. Wackenheim’s clivus-canal line is drawn along the posterior surface of the clivus, and basilar invagination is present if this line is intersected by the odontoid process (see Fig. 181-2). The clivus-canal line consists of an angle formed by Wackenheim’s line and a line along the posterior margin of the dens. Normally, this angle is 120 to 140 degrees, and ventral compression can occur at more-acute angles. Fishgold’s digastric line is measured on the frontal projection and connects the digastric grooves. The line is normally 11 mm ± 4 mm above the atlantoidooccipital junction. The digastric line is the upper limit of position for the odontoid tip.42 Patients with abnormalities of the craniovertebral junction become symptomatic when the effective diameter of the spinal canal at the foramen magnum (from the posterior surface of the odontoid process to the posterior margin of the foramen magnum) is less than 19 mm.43
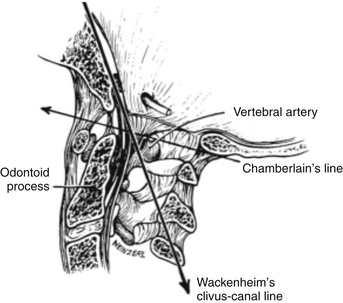
FIGURE 181-2 A midsagittal section of the craniovertebral junction illustrating Chamberlain’s and Wackenheim’s clivus-canal lines.
Special radiologic procedures are necessary for the clarification of the etiology and pathophysiology of the craniovertebral abnormalities.1–3 These examinations of the cervicomedullary junction include MRI (Fig. 181-3A), computed tomography (CT), single- or three-dimensional CT (see Fig. 181-3B), and plain radiographs. These studies provide complementary information.
The most significant examination of the posterior fossa contents and spinal cord is provided by MRI, which demonstrates the medulla and cervical spinal cord with great clarity. Abnormality in size and position of the cervicomedullary junction, abnormality of cerebellar tonsil position, and presence or absence of hydromyelia are demonstrated without the use of ionizing radiation (see Fig. 181-3A). Diagnostic studies of the craniovertebral junction in the flexion-extension positions (with or without contrast material) can be performed with plain radiographic studies and with MRI. MRI protocols have also been developed to provide high-resolution imaging of the ligamentous structures of the craniocervical junction. The alar ligaments, transverse ligament, accessory atlantoaxial ligament, and posterior atlanto-occipital membrane can be clearly identified.44,45
• A predental space greater than 5 mm in children younger than 8 years or greater than 3 mm in patients older than 8 to 10 years is seen.
• On open-mouth odontoid views or coronal CT images, the lateral displacement of the two atlas lateral masses is greater than 6 mm.
• The vertical translation between the clivus and the odontoid is greater than 2 mm.
• Excessive motion on flexion and extension views of the craniocervical junction is observed.
• Disruption of the transverse cruciate ligament, alar ligament, or tectorial membrane and bony malalignment are seen on high-resolution MRI.
Careful preoperative evaluation of the bony relationships on static and dynamic imaging, as well as careful inspection of the ligamentous anatomy, can help determine the need for internal fixation.46
Treatment Algorithm for Craniovertebral Abnormalities
The following algorithm has been proposed (Fig. 181-4) to guide clinical decision making based on the etiology of the lesion, the reducibility of the deformity with traction, direction of encroachment, presence of abnormal ossification centers and growth plates, and stability of the craniocervical junction.47 Based on these criteria, all patients with abnormalities of the craniocervical junction can be divided into six surgical categories.46
The first determination to make for patients with compressive lesions at the craniocervical junction is if the lesion is reducible by positioning or traction. One should start with a low weight (5 to 7 lb) and slowly add additional weight as needed up to 12 to 15 lb (in adults). The alignment can be assessed with radiographs, and MRI during traction provides critical information regarding the need for additional decompression after adequate alignment is achieved. If the lesion is reducible and additional decompression is not required, stabilization alone is necessary to maintain alignment.
Certain situations require an initial conservative approach. For young infants with conditions such as spondyloepiphyseal dysplasia and Goldenhar’s syndrome, a custom-built orthosis, to allow continued growth, can be used when instability is identified. If adequate bone growth occurs over time, the brace can be gradually discontinued. However, if after 4 years sufficient bone growth has not occurred, and craniocervical instability still exists, arthrodesis is required. In children with Grisel’s syndrome, a firm cervical collar is required while the infection is being treated and until ligamentous laxity resolves. Our experience has confirmed that fusion is rarely required in these patients.46
Operative Techniques
Reducible Pathologic Conditions Requiring External Immobilization Only
We have purposely omitted from this discussion odontoid and atlantoaxial fractures that require fixation only because the causes and treatment of these entities are well documented elsewhere.48,49 Although some reducible pathologic conditions can be realigned by positioning alone, most require up to 12 to 15 lb of skeletal traction with a halo ring.