Chapter 89 Colloids and blood products
Colloids are plasma expanders used to expand the blood volume. Hydrostatic and osmotic forces dictate movement of fluid between the different compartments of the body across semipermeable membranes (Starling’s forces).
Colloid osmotic pressure (COP) is the osmotic pressure exerted by the macromolecules (the colloid molecules).1,2 This can be measured using a membrane transducer system in which the membrane is freely permeable to small ions and water but largely impermeable to the colloid molecules.3 The membrane pore size and the molecular size distribution of the colloid being studied will dictate the measured value (see Figures 89.2 and 89.3).2,3 Capillary walls are made up of endothelial cells, which are freely permeable to small ions such as Na+ and Cl−, but are relatively impermeable to larger molecules such as colloids (Figure 89.3). A colloid is a homogeneous non-crystalline substance consisting of large molecules or ultramicroscopic particles of one substance dispersed through a second substance – the particles do not settle and cannot be separated out by ordinary filtering or centrifuging like those of a suspension such as blood.2
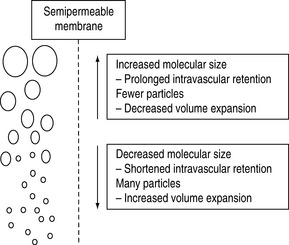
Figure 89.2 Polydispersity of colloid molecular size.
(Reproduced with permission from Grocott MPW, Mythen MG. Fluid therapy. In: Goldhill DR, Strunin L (eds) Clinical Anaesthesiology. London: Baillière Tindall; 1999: 363–81.)
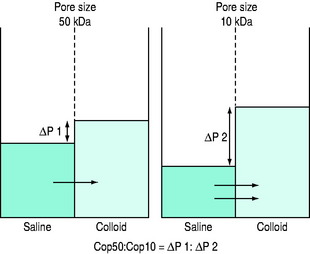
Figure 89.3 Schematic of technique for measurement of the COP50:COP10 ratio.
(Reproduced with permission from Grocott MPW, Mythen MG. Fluid therapy. In: Goldhill DR, Strunin L (eds) Clinical Anaesthesiology. London: Baillière Tindall; 1999: 363–81.)
In practical terms, it is a fluid that when infused into the intravascular space should expand the blood volume by the volume infused.3,4 Crystalloids have larger volumes of distribution dependent on their composition (Figure 89.1 and Table 89.1). Colloids or plasma substitutes have molecular weights (MW) > 35 kDa, and infusion results in an initial increase in blood osmotic pressure. The ideal properties of a colloid are:
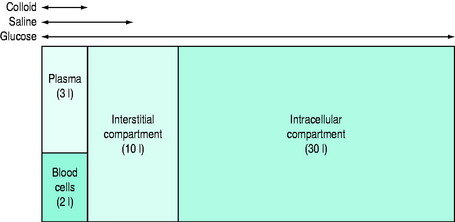
Figure 89.1 Simplified theoretical volumes of distribution of infused isotonic solutions of an ideal colloid, saline and glucose.
(Reproduced with permission from Grocott MPW, Mythen MG. Fluid therapy. In: Goldhill DR, Strunin L (eds) Clinical Anaesthesiology. London: Baillière Tindall; 1999: 363–81.)
Table 89.1 Comparison of contents, osmolarity and pH of crystalloid solutions for intravenous administration
COLLOID SOLUTIONS
There are two major groups of colloids – plasma derivatives or semisynthetics.5 Plasma derivatives include human albumin solutions, plasma protein fraction, fresh frozen plasma and immunoglobulin solutions. Three principal types of semisynthetic colloid molecule are commonly used in intravenous solutions – gelatins, dextrans and hydroxyethyl starches. All colloids are presented dissolved in a crystalloid solution. Isotonic saline is still the most commonly used carrier solution but isotonic glucose, hypertonic saline and isotonic balanced electrolyte solutions are also used.
Colloids often do not consist of uniform sized molecules. Human albumin solution contains more than 95% albumin with a uniform molecular size. This is described as monodisperse. Conversely, all the semisynthetic colloids have a distribution of molecular sizes and are described as polydisperse.1,2 The size–weight relationship is relatively constant although some colloids can have equivalent MW with different molecular size – succinylated and urea-linked gelatins have almost identical molecular weights but the succinylated product undergoes conformational change due to an increase in negative charge and is physically larger.2,3
HUMAN ALBUMIN SOLUTION
Human albumin is thought of as an ideal colloid and is commonly the reference solution against which other colloids are judged. Human albumin is a naturally occurring monodisperse colloid. Solutions (5% and 20%) are prepared from human plasma and heat treated to ensure that neither hepatitis nor HIV can be transmitted. It has a relatively short (∼1 year) shelf life at room temperature, but a 5-year shelf life at 2–8 °C. Human albumin 5% is used for the treatment of hypovolaemia in a wide variety of clinical conditions. Concentrated salt-poor 20% human albumin is used for the treatment of hypoalbuminaemia in the presence of salt and water overload (e.g. hepatic failure with ascites). Albumin has putative advantages that include limiting free radical-mediated damage but the importance of a normal serum albumin level remains uncertain.6–8
Human-derived colloid has a number of significant disadvantages. These are expensive products and concerns have been raised about transmission of infectious agents, such as new variant Creutzfeldt–Jakob disease (nvCJD) associated with bovine spongiform encephalopathy (BSE), that are not removed by currently available techniques. Concerns have also been raised by the conclusions of a highly controversial meta-analysis suggesting that the use of human albumin solution may be associated with increased mortality in the critically ill. These conclusions were probably unjustified,8,9 but there is certainly no evidence that the use of human albumin has any advantages over less expensive semisynthetic alternatives10,11 or indeed crystalloids. However, the latter applies to all colloids.12–14
GELATINS
Gelatins are prepared by hydrolysis of bovine collagen.15 The commonly available preparations are succinylated gelatin (Gelofusine) and urea-linked gelatin–polygeline (Haemaccel).1,2 Because of the significant calcium content of Haemaccel, citrated blood should not be infused through a giving set that has been previously used for this product; however, this does not apply to SAGM (saline–adenine–glucose–mannitol) blood. Gelatins are relatively inexpensive and stable, with long shelf lives. The gelatins’ plasma volume expanding effect only lasts about 90–120 minutes.3,4 They are mainly eliminated by renal excretion.
DEXTRANS
Dextrans are polysaccharides biosynthesised commercially from sucrose by the bacterium Leuconostoc mesenteroides using the enzyme dextran sucrase.1,2 This produces a high molecular weight dextran that is then cleaved by acid hydrolysis and separated by repeated ethanol fractionation to produce a final product with a relatively narrow molecular weight range. The products in current clinical use are described by their MW, Dextran 40 and Dextran 70 having MWs of 40 000 and 70 000 Da, respectively.