Chapter 146 Charged-Particle Irradiation of Uveal Melanoma
Introduction
Radiotherapy is currently the most widely used method for treating intraocular melanomas, having replaced enucleation as the standard of care for most tumors. It offers the advantage of eye salvage and retention of vision in many cases, although a survival advantage has not been demonstrated.1
There are two major radiotherapeutic techniques for the treatment of uveal melanomas: radioactive plaques2,3 sutured on the sclera over the area of the tumor, and external beam irradiation using charged particles such as protons,4 helium ions5 and the gamma knife.6,7 The advantages of charged-particle irradiation are based on the physical characteristics of particles, which make possible highly localized dose distributions,8–10 and provide highly attractive depth-dose distribution patterns.11 Although effective, helium ion irradiation5 is no longer in use due to its high cost. The dose distributions achieved with stereotactic radiosurgery using the gamma knife6,7 do not appear to be as attractive as those of proton irradiation.7 Additional experience and long-term follow-up are necessary to better evaluate its efficacy as most published studies to date have reported short-term results in small groups of patients.
Protons are positive, singly charged particles that have minimal scatter and a well-defined, finite, and energy-dependent tissue range. Proton beams can be collimated to deliver maximum density of ionization in a sharply focused, localized volume because of the inherent Bragg peak at the end of the beam path. The Bragg peak can be broadened to cover a tumor at any depth (Fig. 146.1). The uniform dose of radiation delivered to the whole tumor and the sharp reduction of the dose outside the treated area allow tumors located near critical structures to be treated with the possibility of retaining visual acuity. Larger tumors can be treated because the overall irradiated volume is reduced. These properties should improve the therapeutic ratio of local control versus complications.
Early experimental work in monkey eyes, followed by clinical and histopathological studies in humans provide evidence of the advantageous properties of protons. The Bragg peak of small-diameter collimated beams positioned on the fundus by stereotactic radiography produced lesions confined to the intended radiation field.12 Normal retinal architecture as close as 1 mm from the edge of radiation-induced lesions was seen in irradiated monkey eyes more than 3.5 years after proton treatment.13 Similar data on humans substantiate the favorable effects obtained with protons. Using mathematical superimposition of fundus photographs, computer treatment plans, and visual fields, correlation between patterns of visual loss and radiation isodose calculations was established.14,15 Histologic examination of irradiated tumors revealed vessel thrombosis exclusively in retina overlying the tumor,16 and reduced or absent choroidal vasculature and RPE beneath and above the tumor but intact tissue adjacent to it.17
Treatment
Operative technique
The conjunctiva is incised, and the tumor is localized by transillumination, indirect ophthalmoscopy, or both. The episcleral tissues over the area of the tumor are examined carefully for evidence of extrascleral extension. The edges of the tumor are marked with a surgical marking pen, and four tantalum rings, 2.5 mm in diameter, are sutured to the sclera at the margins of the tumor. Ring to limbus distances and distances between the rings are measured. Highly elevated tumors can cast a variable shadow during transillumination, depending on the angle of illumination, which may result in an overestimate of the dimensions of the tumor. Therefore, the angle of illumination in the most precise shadow must be carefully chosen. For tumors that extend into the ciliary body and iris, rings are placed at the posterior margin of the tumor and accurate measurements are made of the distance from the rings to the anterior margin of the lesion. If tumors are in contact with the optic nerve, rings are placed only at the anterior and lateral margins of the lesion, and the distance from the rings to the posterior margin is estimated from fundus photographs. The tumor is transilluminated again after suturing of the rings (Fig. 146.2), the distances from the rings to the edges of the tumor are measured, and careful drawings of the tumor margins in relation to the rings are made. No operation is necessary for lesions confined to the ciliary body. The margins of the tumor in relation to anatomic landmarks of the iris and conjunctiva are defined by transillumination on the ocular surface.
Treatment planning
An interactive three-dimensional treatment planning computer program (EYEPLAN, Martin Sheen, Clatterbridge Centre for Oncology, Bebington, UK) facilitates the selection of the appropriate fixation angle, which is chosen to minimize irradiation of the lens, optic disc, and fovea.18 Such a program helps to select the best direction of gaze relative to the proton beam line; design the shape of the field-defining aperture; determine the proton range modulation necessary to encompass the target volume; determine the extent to which important structures are included within (or excluded from) the beam; and indicate the relative positions of the tumor-defining rings, beam aperture, and crosshairs that should be observed in the alignment film when the patient is correctly aligned. The treatment planning program creates a spherical model of a globe that is scaled to the ultrasonically determined length of the patient’s eye and the position of the tantalum rings previously sutured to the sclera, determined by orthogonal X-rays taken of the patient in the treatment position (gazing in three different directions). The structures of the eye are added to the model, including the anterior chamber depth and of the lens obtained ultrasonographically. The program then superimposes on this globe a three-dimensional model of the tumor based on fundus pictures and ultrasonograms. The fundus photographs are especially useful for very posterior tumors and tumors abutting the optic nerve where it is impossible to surround the tumor with marker rings. Two tumors can be created if needed, e.g., if there is a tumor with an irregular shape. Tumors in the iris or ciliary body, for which placement of marker rings is unnecessary, are drawn from clinical and ultrasound information.
The program automatically designs an aperture that gives a 3 mm margin around the tumor, at which the dose falls to 50%. This margin may be reduced to 2.5 mm for a tumor border at the limbus or increased to 4 mm for a patient without surgical markers. The program calculates the maximum and minimum depths of the tumor and allows the user to choose proximal and distal margins to give the needed beam range and modulation. The program calculates dose distributions on the fundus displayed in the geometry of a wide-angle fundus photograph (Fig. 146.3) and in any plane through the eye (Fig. 146.4). It also calculates dose–volume histograms for the tumor and many structures of the eye.
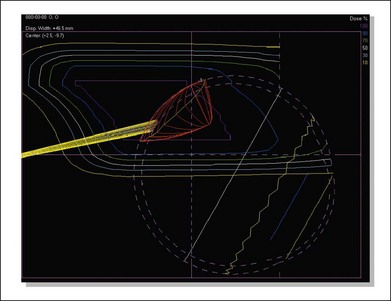
Fig. 146.4 Isodose curves in a vertical plane through the eye parallel to the beam direction for the case shown in Figure 146.3. The tumor is shown in red and the yellow cone represents the optic nerve. The innermost magenta line corresponds to the area receiving 100% of the dose (70 CGE) and the outermost yellow line corresponds to the area receiving 10% of the dose (7 CGE).
Treatment techniques
The patient is seated in a specially designed chair, and his or her head is immobilized with a bite-block made of a dental impression compound and fastened to the headholder. Individually contoured plastic masks mounted into a frame are also used for head fixation (Fig. 146.5). Orientation of the patient’s eye is established by voluntary fixation of the eye to be treated (the other eye is covered) on a small light that is attached to the collimator. If the vision is poor in the eye to be treated, the other eye can be used for fixation. The eyelids are held open with a lid speculum.
The eye is monitored using a high-magnification closed-circuit television system with its effective viewing point on the beam axis. This system provides a magnified image of 10 times the size of the patient’s eye and permits continuous monitoring of the eye’s position throughout the procedure. The alignment of the proton beam is achieved with orthogonal X-rays. The lesion, defined spatially by the radiopaque tantalum rings, can be positioned by translating the headholder until it is in the desired position relative to the beam axis. For tumors treated without surgical localization, a light beam coaxial with the central axis of the proton beam is used to position the tumor relative to the beam during treatment.8,19,20 Positioning is achieved with a fluoroscopic system that provides a virtually instantaneous picture held on an image-storage device. This system hastens alignment and assists in confirming eye immobilization during treatment. The alignment procedure lasts approximately 15 minutes. The patient is asked to fixate, and the eye’s position is observed on the television monitor in the control area. The treatment field is checked with a beam-simulation field light, and if the position and fixation are satisfactory, the treatment begins. If eye movement of more than 0.5 mm is observed, the treatment is halted immediately. Each treatment takes approximately 1–2 minutes, most without interruption.
Radiation dose
The standard dose administered for most tumors is 70 cobalt Gy equivalents (CGE) delivered in five equal fractions in 5 days (63.6 proton Gy times 1.1 relative biologic effectiveness equals 70 CGE). Dose fractionation increases the radiosensitivity of tumor tissue, as increased oxygenation of hypoxic tumor cells occurs between fractions. We selected large fractions based on favorable clinical results demonstrated with the use of a small number of relatively large dose fractions10,21 in patients with skin melanomas. At the prescribed dose of 70 CGE, we estimate that the optic nerve and macula receive the full dose when the tumor is less than 1 mm from these visual structures, half the dose (35 CGE) when the tumor is located 3 mm from these structures, and a small dose (≤15 CGE) when the tumor is peripheral (beyond 9 mm). As part of a randomized clinical trial to establish safety and efficacy of a dose reduction, 94 patients received a lower dose of 50 CGE.22 No significant differences were found between patients who received the standard dose and those who received the lower dose with regard to tumor control and ocular complications. Thus, the optimal dose level to achieve tumor control and minimize ocular morbidity has not been established. Nevertheless, in select patients, i.e., those with small- or medium-sized tumors located near the optic nerve or fovea, a dose of 50 CGE is chosen in an effort to reduce vision-threatening treatment complications. At several facilities in Europe, a total dose of 60 CGE is given in four equal fractions over 4 days.23–25
Clinical findings in treated patients
To date, more than 3500 patients with uveal melanomas have been treated at Massachusetts Eye and Ear Infirmary and Massachusetts General Hospital. The mean age of patients at the time of diagnosis was 61 years. Diagnosis before age 40 was uncommon, representing 12% of treated patients. Bilateral involvement and extrascleral extension were rare (approximately 1% and 4%, respectively). The median basal diameter of treated patients was 13.2 mm (range: 5.0–28.0 mm) and median tumor height was 5.3 mm (range: 0.6–17.1 mm). Approximately 26% of patients had small tumors (≤10 mm greatest diameter and 2.5 mm in height), and approximately half had medium-sized tumors (>10 mm and ≤16 mm in diameter and/or >2.5 mm and ≤10 mm in height). Nearly 20% had large tumors (>16 mm in diameter and >10 mm in height). More than two-thirds of the treated tumors were located within 3 mm of the optic nerve or macula.26 Approximately 20% of the patients had 20/20 or better visual acuity at presentation, and less than 10% had visual acuity of counting fingers or worse.
Results
Tumor regression
The majority of treated tumors show some regression after the first 6 months of treatment, with a usual range between 1 and 24 months.27 Disappearance of the lesion, or formation of a flat scar is observed in 15% of eyes. Resolution of a secondary serous retinal detachment is usually the earliest finding. Detachments can transiently increase in size during the first few months after treatment, but most eventually resolve. Continuous regression of the tumor over several years is frequently observed. Tumor regression is thought to be due primarily to direct killing of tumor cells by irradiation, and secondarily to the effect of radiotherapy on the tumor vasculature. Cell death from irradiation is achieved by damage to chromosomal DNA, with subsequent loss of proliferative potential. Damage to DNA is lethal when the cell enters mitosis. Delayed regression, observed in some irradiated tumors, is probably due to prolonged intermitotic phases of melanoma cells. This protracted pattern of tumor regression has been supported by histologic studies of tumors enucleated at varying times after proton therapy, which showed a greater decline of mitotic figures with longer periods between irradiation and enucleation. Tumors enucleated more than 30 months after irradiation had no mitotic figures.28 Rapid regression of tumors has been associated with higher rates of metastasis in both proton-irradiated29 and plaque-treated patients,30 suggesting that more aggressive tumors, due to more rapidly dividing cells, are more radiosensitive.
Visual outcomes
Visual acuity after proton beam irradiation depends on the size of the tumor and its location relative to the fovea and the optic nerve31 (Fig. 146.6). In eyes with tumors located farther than 3 mm from these structures, tumor destruction usually occurs without functionally significant radiation vasculopathy.32 In a study of 558 patients who had undergone proton therapy for small- to moderate-sized tumors located within 4 disc diameters of the optic nerve or macula, the 5-year rate of vision loss to worse than 20/200 was 68%. Risk factors for vision loss included dose to the macula, tumor height, poorer baseline vision and a history of diabetes.33 In a multivariate regression model,26 distance of the tumor from the optic nerve and macula, tumor height, baseline visual acuity, degree of retinal detachment, history of diabetes, and tumor diameter were found to be associated with vision loss. Coefficients derived from this model were used to calculate risk scores, which were then used to estimate probabilities of vision loss. The probability of developing significant vision loss (worse than 20/200) by 10 years after proton therapy varied between 16% for patients in the “low-risk” category (Fig. 146.7) to 99% for patients in the “high-risk” group.
Visual prognosis after proton irradiation for patients with peripapillary and parapapillary tumors is poor, with a 5-year rate of vision loss to worse than 20/200 of 80%. On the other hand, 56% retain vision of counting fingers or better at this time point, indicating that proton irradiation is a viable alternative to enucleation for these tumors, which are often unsuitable for plaque radiotherapy (Fig. 146.8).
Complications
Surgical complications from the suturing of tantalum rings have been relatively few. Transient diplopia has been observed for a few weeks after the operation in only a small number of patients since disinsertion of extraocular muscles is never required. In patients with large tumors, intratumor hemorrhage may occasionally occur as a result of surgical manipulation. Lid dermatitis, madarosis, and epiphora from punctal occlusion have occurred in patients whose eyelids could not be retracted completely from the irradiation field. Corneal epitheliopathy has developed in a small number of cases with large ciliochoroidal melanomas and usually responds to artificial tears. Rubeosis iridis and neovascular glaucoma, the most serious complications because they may lead to enucleation, are observed in approximately 16% of treated eyes and are usually associated with large tumor volume. In one study of 127 patients treated by proton irradiation because their tumors were too large for plaque radiotherapy, 34% developed rubeosis during a median follow-up period of 36 months.34 At Massachusetts Eye and Ear Infirmary (MEEI), neovascular glaucoma was the primary reason for enucleation in close to one-half of patients (45.9%) with large tumors who required the procedure.
Radiation vasculopathy, consisting of capillary closure, telangiectasis, microaneurysm formation, hemorrhages, exudates, and vascular sheathing, is usually observed in the irradiated area. In macular and paramacular melanomas (<3 mm from the fovea), radiation maculopathy and papillopathy may develop leading to vision loss.9,32,35 The 5-year cumulative rates of maculopathy and papillopathy in patients with tumors within 4 disc diameters of the macula and/or optic nerve were 64% and 35%, respectively, with increased risk of these complications associated with increased dose to critical structures.33 The rate of papillopathy was 56.8% at 5 years after proton therapy for tumors located within 1 disc diameter of the optic nerve.36
In a recent study evaluating the natural history of radiation papillopathy, 93 patients with parapapillary melanomas (within 1 disc diameter of the optic nerve and at least 2 disc diameters from the fovea) were followed. By 5 years after proton therapy radiation papillopathy developed in 81% of cases in which the melanoma abutted the optic nerve and in 67% of cases in which the melanoma was 0.1–1 disc diameter from the optic nerve. Of those who developed papillopathy, 42% retained vision of counting fingers or better, and 31% (n = 13) experienced recovery of visual acuity after an initial loss.37 These findings challenge the view that irradiation of the optic nerve will inevitably lead to optic neuropathy and total loss of vision.
Significant cataracts may develop in patients with ciliary body involvement or in cases of highly elevated choroidal melanomas in which the lens receives substantial irradiation.38 The 5- and 10-year cumulative rates of posterior subcapsular cataract (PSC) were 30% and 35%, respectively in an analysis of more than 2000 patients treated with protons over a 20-year period. Rates were dependent upon the dose to the lens; at 5 years post-therapy, 53% developed cataract when >50% of the lens was irradiated compared with 19% when <10% of the lens was irradiated. For eyes with reasonable visual potential, cataract extraction with intraocular lens implantation may be performed. Approximately 50% of patients undergoing cataract extraction after proton beam irradiation for choroidal melanoma had visual acuity of 20/200 or better after surgery.39 There was no evidence that cataract extraction increased the risk of metastasis. Removal of a cataract for better observation of the tumor when there is no likelihood of visual improvement, typically due to underlying radiation maculopathy or papillopathy, is usually unnecessary, since these tumors can be followed by ultrasonography.
In an effort to reduce the ocular morbidity associated with proton therapy, particularly when tumors are located close to critical structures, a randomized, double-blind dose reduction trial22 was completed. The experimental dose selected for the trial was 50 CGE. Patients eligible for the trial were at high risk of radiation papillopathy and maculopathy because their tumors were located near the optic nerve or macula. Five years after proton therapy, lower rates of radiation vasculopathy and visual loss were not demonstrated. A modest beneficial effect of lowering the dose was suggested, with lower rates of visual field defects and radiation papillopathy observed in the 50 CGE dose group. For radiation papillopathy, this effect was observed only in the subgroup of patients with tumors located within one disc diameter of the optic disc. Long-term follow-up (10 years after enrollment) of study subjects did not reveal any late-emerging differences between the two dose groups. This level of dose reduction (28%) may be inadequate to produce appreciable improvement in functional outcomes but further reductions could compromise tumor control.
Recurrence
Local recurrence after charged-particle irradiation is observed in 2–5%.23–25,40 of patients. In one study26 of 2069 consecutive patients treated with proton beam irradiation, 45 tumors (2.9%) demonstrated definitive growth with the earliest occurrence observed 5 months and the latest 11 years after irradiation. Only 23 were marginal recurrences; in the case of anterior tumors involving the ciliary body, marginal recurrences may arise because the pigmented margins of the tumor may be difficult to distinguish from surrounding stroma. Six recurrences were ring melanomas, eight involved extrascleral extension of the tumors and nine showed vertical growth of the tumors. An additional 15 eyes were enucleated at other centers because tumor growth was suspected. The 15-year probability of local tumor control, based on confirmed and possible recurrences combined (n = 60), was 95% (95% CI: 93–96%). Similar control rates have been reported in another large series of patients (n = 2435) treated in Lausanne, Switzerland.23 Five-year control rates after brachytherapy have been reported to be between 92% and 87%.41–43 In some cases, eyes with local recurrence can be retreated successfully with repeat proton irradiation44 or – for cases in which the recurrence is marginal and flat – laser photocoagulation.
A comparison of patients irradiated with iodine-125 plaques versus helium ions, revealed a recurrence rate in patients who received brachytherapy that was more than three times that of patients who received heavy particle irradiation (12-year cumulative rates were 19% and 5%, respectively).45 Suboptimal plaque positioning46 in posteriorly located tumors may be one explanation for these poorer outcomes. Proton irradiation may be a better choice of treatment for posteriorly located tumors. A recent study of long-term outcomes after proton irradiation for patients with tumors contiguous to the optic nerve (within 1 disc diameter) revealed a 5-year tumor recurrence rate of 3.3%, virtually identical to the rate seen in patients treated during the same period with tumors farther away from the nerve.36 In contrast, tumor recurrence was reported in 10% of patients who received brachytherapy for tumors overhanging the disc.47 The use of notched plaques and other advances in plaque design may improve tumor control in these cases.47–49
Achieving tumor control is of particular importance because local recurrence has been shown to elevate the risk of metastasis.23,25,50 Survival is poorer among patients with local recurrences; 10-year survival rates were 72.6% for patients who experienced tumor control and 47.5% for patients who had tumor regrowth.23 However, it is not clear if recurrence per se is actually a risk factor for metastasis or if it is just an indicator of a more aggressive tumor, which is more likely to metastasize.
Enucleation
The probability of retaining the eye 2 years after proton irradiation is 95%, and the probability is 90% after 5 years.51 The 10-year rate of eye retention was 89% in a group of 1541 patients treated by proton therapy and followed for a median of 8 years;50 137 patients underwent enucleation after radiotherapy for complications (n = 103) or tumor regrowth (n = 34). The complication most likely to result in enucleation is neovascular glaucoma. The leading risk factors for enucleation are tumor height,26,51 proximity of the tumor to critical structures (macula, fovea,51 optic nerve), tumor diameter, tumor pigmentation and tumor shape.26
Metastasis and survival
Annual rates of melanoma-related mortality are highest 3–6 years after irradiation. The 5-year cumulative probability of developing metastasis after proton irradiation is approximately 20%.25,52 The probability of metastatic death at 15 years after proton treatment, using individual risk scores to estimate rates, varies between 5% for patients having the lowest-risk characteristics and 63% for those with the highest-risk characteristics.26 The liver is primarily involved in 90% of patients with metastasis.52 In 145 patients who developed metastasis after proton irradiation, median time to metastasis was 2.4 years after irradiation, and most patients were found to be symptomatic before diagnosis.53 It remains unclear if early diagnosis of metastatic uveal melanoma has an impact on survival. A recent study compared patients who were diagnosed with metastatic uveal melanoma after proton irradiation incidentally or by routine surveillance to those who were diagnosed after developing symptoms. There were no differences between the two groups with regard to median time from diagnosis of primary tumor to metastatic death, suggesting that earlier diagnosis does not have a beneficial effect on survival.54
Adjuvant interferon therapy after primary treatment has not been successful in reducing the incidence of metastasis,55 and therapeutic results for visceral metastatic melanoma are poor. Less than 15% of patients survive to 1 year after the detection of metastasis.53 In studies of patients who have developed metastasis after undergoing any type of primary ocular therapy, median survival is 2–9 months.53,56,57 Significantly longer survival (from time of metastasis diagnosis) occurs among younger patients.53 Few remissions have been realized with chemoembolization of the liver and immunotherapy with interleukin-2, alone or in combination with other chemotherapeutics, and toxicity is high.56,58,59 Patients who undergo intrahepatic arterial perfusion have somewhat better outcomes,60 but an important limitation to such regional therapies is that extrahepatic disease may develop.
Results of nonrandomized studies comparing survival rates for patients with uveal melanoma after treatment with proton beam irradiation61 and other forms of radiotherapy62 with survival rates after enucleation suggest that treatment choice has little effect on survival. Results from the Collaborative Ocular Melanoma Study showed no differences in 5-year rates of death due to histopathologically confirmed metastatic melanoma between patients randomized to undergo enucleation and those who received 125-I brachytherapy (11% versus 9%, respectively).1
Conclusion
Proton irradiation of uveal melanomas is quite successful in achieving local tumor control. The dose distributions are particularly advantageous for the treatment of large tumors and tumors located near the optic disc or fovea, with many patients maintaining visual function in the treated eye. Nevertheless, there can be significant ocular morbidity associated with treatment of such cases. Outcomes after treatment of radiation complications with systemic corticosteroids and anticoagulation have been disappointing,63,64 but preliminary reports of intravitreal triamcinolone65 and bevacizumab66,67 suggest some efficacy for radiation maculopathy and papillopathy. Among several ongoing trials evaluating pharmacologic agents to reduce radiation complications is a masked, randomized trial evaluating two doses of intravitreal ranibizumab in patients with parapapillary or paramacular tumors treated by proton irradiation.
1 Diener-West M, Earle JD, Fine SL, et al. The COMS randomized trial of iodine 125 brachytherapy for choroidal melanoma, III: initial mortality findings. COMS Report No. 18. Arch Ophthalmol. 2001;119:969–982.
2 Lommatzsch P. Beta-irradiation of choroidal melanoma with 106Ru/106Rh applicators. 16 years’ experience. Arch Ophthalmol. 1983;101:713–717.
3 Packer S, Rotman M. Radiotherapy of choroidal melanoma with iodine-125. Ophthalmology. 1980;87:582–590.
4 Gragoudas ES, Seddon J, Goitein M, et al. Current results of proton beam irradiation of uveal melanomas. Ophthalmology. 1985;92:284–291.
5 Char DH, Kroll SM, Castro J. Ten-year follow-up of helium ion therapy for uveal melanoma. Am J Ophthalmol. 1998;125:81–89.
6 Haas A, Pinter O, Papaefthymiou G, et al. Incidence of radiation retinopathy after high-dosage single-fraction gamma knife radiosurgery for choroidal melanoma. Ophthalmology. 2002;109:909–913.
7 Mueller AJ, Talies S, Schaller UC, et al. Stereotactic radiosurgery of large uveal melanomas with the gamma-knife. Ophthalmology. 2000;107:1381–1387. discussion 1387–1388
8 Gragoudas E, Goitein M, Koehler A, et al. Proton irradiation of choroidal melanomas: Preliminary results. Arch Ophthalmol. 1978;96:1583–1591.
9 Gragoudas E, Goitein M, Seddon J, et al. Preliminary results of proton beam irradiation of macular and paramacular melanomas. Br J Ophthalmol. 1984;68:479–485.
10 Gragoudas ES, Goitein M, Verhey L, et al. Proton beam irradiation of uveal melanomas. Results of 5 1/2-year study. Arch Ophthalmol. 1982;100:928–934.
11 Suit HD, Goitein M, Tepper J, et al. Explorotory study of proton radiation therapy using large field techniques and fractionated dose schedules. Cancer. 1975;35:1646–1657.
12 Constable I, Koehler A. Experimental ocular irradiation with accelerated protons. Investigative Ophthalmology. 1974;13:280–287.
13 Gragoudas ES, Zakov NZ, Albert DM, et al. Long-term observations of proton-irradiated monkey eyes. Arch Ophthalmol. 1979;97:2184–2191.
14 Meecham W, Char D, Chen G, et al. Correlation of visual field, treatment fields, and dose in helium ion irradiation of uveal melanoma. Am J Ophthalmol. 1985;100:658–665.
15 Park SS, Walsh SM, Gragoudas ES. Visual-field deficits associated with proton beam irradiation for parapapillary choroidal melanoma. Ophthalmology. 1996;103:110–116. [Erratum Ophthalmology 1996;103:699.]
16 Saornil M, Egan K, Gragoudas E, et al. Histopathology of proton beam-irradiated vs enucleated uveal melanomas. Arch Ophthalmol. 1992;110:1112–1118.
17 Seddon JM, Gragoudas ES, Albert DM. Ciliary body and choroidal melanomas treated by proton beam irradiation. Hisopathologic study of eyes. Arch Ophthalmol. 1983;101:1402–1408.
18 Goitein M, Miller T. Planning proton therapy of the eye. Med Phys. 1983;10:275–283.
19 Gragoudas ES, Goitein M, Koehler A, et al. Proton irradiation of malignant melanoma of the ciliary body. Br J Ophthalmol. 1979;63:135–139.
20 Gragoudas E, Goitein M, Verhey L, et al. Proton beam irradiation. An alternative to enucleation for intraocular melanomas. Ophthalmology. 1980;87:571–581.
21 Gragoudas E, Seddon J, Egan K, et al. Long-term result of proton beam irradiated uveal melanomas. Ophthalmology. 1987;94:349–353.
22 Gragoudas ES, Lane AM, Regan S, et al. A randomized controlled trial of varying radiation doses in the treatment of choroidal melanoma. Arch Ophthalmol. 2000;118:773–778.
23 Egger E, Schalenbourg A, Zografos L, et al. Maximizing local tumor control and survival after proton beam radiotherapy of uveal melanoma. Int J Radiat Oncol Biol Phys. 2001;51:138–147.
24 Damato B, Kacperek A, Chopra M, et al. Proton beam radiotherapy of choroidal melanoma: the Liverpool-Clatterbridge experience. Int J Radiat Oncol Biol Phys. 2005;62:1405–1411.
25 Dendale R, Lumbroso-Le Rouic L, Noel G, et al. Proton beam radiotherapy for uveal melanoma: results of Curie Institut-Orsay proton therapy center (ICPO). Int J Radiat Oncol Biol Phys. 2006;65:780–787.
26 Gragoudas E, Li W, Goitein M, et al. Evidence-based estimates of outcome in patients irradiated for intraocular melanoma. Arch Ophthalmol. 2002;120:1665–1671.
27 Wilkes SR, Gragoudas ES. Regression patterns of uveal melanoma after proton beam irradiation. Ophthalmology. 1982;89:840–844.
28 Gragoudas ES, Egan KM, Saornil MA, et al. The time course of irradiation changes in proton beam-treated uveal melanomas. Ophthalmology. 1993;100:1555–1559. discussion 1560
29 Glynn R, Seddon J, Gragoudas E, et al. Evaluation of tumor regression and other prognostic factors for early and late metastasis after proton irradiation of uveal melanoma. Ophthalmology. 1989;96:1566–1573.
30 Kaiserman I, Anteby I, Chowers I, et al. Post-brachytherapy initial tumour regression rate correlates with metastatic spread in posterior uveal melanoma. Br J Ophthalmol. 2004;88:892–895.
31 Seddon JM, Gragoudas ES, Polivogianis L, et al. Visual outcome after proton beam irradiation of uveal melanoma. Ophthalmology. 1986;93:666–674.
32 Seddon JM, Gragoudas ES, Egan KM, et al. Uveal melanomas near the optic disc or fovea. Visual results after proton beam irradiation. Ophthalmology. 1987;94:354–361.
33 Gragoudas ES, Li W, Lane AM, et al. Risk factors for radiation maculopathy and papillopathy after intraocular irradiation. Ophthalmology. 1999;106:1571–1578.
34 Foss AJ, Whelehan I, Hungerford JL, et al. Predictive factors for the development of rubeosis following proton beam radiotherapy for uveal melanoma. Br J Ophthalmol. 1997;81:748–754.
35 Guyer D, Mukai S, Egan K, et al. Radiation maculopathy after proton beam irradiation for choroidal melanoma. Ophthalmology. 1992;99:1278–1285.
36 Lane AM, Kim IK, Gragoudas ES. Proton irradiation for peripapillary and parapapillary melanomas. Arch Ophthalmol. 2011;129:1127–1130.
37 Kim IK, Lane AM, Egan KM, et al. Natural history of radiation papillopathy after proton beam irradiation of parapapillary melanoma. Ophthalmology. 2010;117:1617–1622.
38 Gragoudas ES, Egan KM, Walsh SM, et al. Lens changes after proton beam irradiation for uveal melanoma. Am J Ophthalmol. 1995;119:157–164.
39 Gragoudas ES, Egan KM, Arrigg PG, et al. Cataract extraction after proton beam irradiation for malignant melanoma of the eye. Arch Ophthalmol. 1992;110:475–479.
40 Gragoudas E, Egan K, Seddon J, et al. Intraocular recurrence of uveal melanoma after proton beam irradiation. Ophthalmology. 1992;99:760–766.
41 Char D, Quivey J, Castro J, et al. Helium ions versus iodine 125 brachytherapy in the management of uveal melanoma. A prospective, randomized, dynamically balanced trial. Ophthalmology. 1993;100:1547–1554.
42 Gunduz K, Shields CL, Shields JA, et al. Radiation complications and tumor control after plaque radiotherapy of choroidal melanoma with macular involvement. Am J Ophthalmol. 1999;127:579–589.
43 Jensen AW, Petersen IA, Kline RW, et al. Radiation complications and tumor control after 125I plaque brachytherapy for ocular melanoma. Int J Radiat Oncol Biol Phys. 2005;63:101–108.
44 Marucci L, Lane AM, Li W, et al. Conservation treatment of the eye: Conformal proton reirradiation for recurrent uveal melanoma. Int J Radiat Oncol Biol Phys. 2006;64:1018–1022.
45 Char DH, Kroll S, Phillips TL, et al. Late radiation failures after iodine 125 brachytherapy for uveal melanoma compared with charged-particle (proton or helium ion) therapy. Ophthalmology. 2002;109:1850–1854.
46 Harbour JW, Murray TG, Byrne SF, et al. Intraoperative echographic localization of iodine 125 episcleral radioactive plaques for posterior uveal melanoma. Retina. 1996;16:129–134.
47 Sagoo MS, Shields CL, Mashayekhi A, et al. Plaque radiotherapy for juxtapapillary choroidal melanoma overhanging the optic disc in 141 consecutive patients. Arch Ophthalmol. 2008;126:1515–1522.
48 Finger PT. Finger’s “slotted” eye plaque for radiation therapy: treatment of juxtapapillary and circumpapillary intraocular tumours. Br J Ophthalmol. 2007;91:891–894.
49 Sagoo MS, Shields CL, Mashayekhi A, et al. Plaque radiotherapy for choroidal melanoma encircling the optic disc (circumpapillary choroidal melanoma). Arch Ophthalmol. 2007;125:1202–1209.
50 Egan K, Ryan L, Gragoudas E. Survival implications of enucleation after definitive radiotherapy for choroidal melanoma: An example of regression on time-dependent covariates. Arch Ophthalmol. 1998;116:366–370.
51 Egan K, Gragoudas E, Seddon J, et al. The risk of enucleation after proton beam irradiation of uveal melanoma. Ophthalmology. 1989;96:1377–1383.
52 Gragoudas E, Seddon J, Egan K, et al. Metastasis from uveal melanoma after proton beam irradiation. Ophthalmology. 1988;95:992–999.
53 Gragoudas E, Egan K, Seddon J, et al. Survival of patients with metastases from uveal melanoma. Ophthalmology. 1991;98:383–390.
54 Kim IK, Lane AM, Gragoudas ES. Survival in patients with presymptomatic diagnosis of metastatic uveal melanoma. Arch Ophthalmol. 2010;128:871–875.
55 Lane AM, Egan KM, Harmon D, et al. Adjuvant interferon therapy for patients with uveal melanoma at high risk of metastasis. Ophthalmology. 2009;116:2206–2212.
56 Bedikian AY, Legha SS, Mavligit G, et al. Treatment of uveal melanoma metastatic to the liver: a review of the M. D. Anderson Cancer Center experience and prognostic factors. Cancer. 1995;76:1665–1670.
57 Eskelin S, Pyrhonen S, Hahka-Kemppinen M, et al. A prognostic model and staging for metastatic uveal melanoma. Cancer. 2003;97:465–475.
58 Proebstle TM, Scheibenbogen C, Sterry W, et al. A phase II study of dacarbazine, cisplatin, interferon-alpha and high-dose interleukin-2 in ‘poor-risk’ metastatic melanoma. Eur J Cancer. 1996;32A:1530–1533.
59 Flaherty LE, Unger JM, Liu PY, et al. Metastatic melanoma from intraocular primary tumors: the Southwest Oncology Group experience in phase II advanced melanoma clinical trials. Am J Clin Oncol. 1998;21:568–572.
60 Alexander HR, Jr., Libutti SK, Pingpank JF, et al. Hyperthermic isolated hepatic perfusion using melphalan for patients with ocular melanoma metastatic to liver. Clin Cancer Res. 2003;9:6343–6349.
61 Seddon JM, Gragoudas ES, Egan KM, et al. Relative survival rates after alternative therapies for uveal melanoma. Ophthalmology. 1990;97:769–777.
62 Augsburger J, Gamel J, Sardi V, et al. Enucleation vs cobalt plaque radiotherapy for malignant melanomas of the choroid and ciliary body. Arch Ophthalmol. 1986;104:655–661.
63 Danesh-Meyer HV, Savino PJ, Sergott RC. Visual loss despite anticoagulation in radiation-induced optic neuropathy. Clin Experiment Ophthalmol. 2004;32:333–335.
64 Girkin CA, Comey CH, Lunsford LD, et al. Radiation optic neuropathy after stereotactic radiosurgery. Ophthalmology. 1997;104:1634–1643.
65 Shields CL, Demirci H, Marr BP, et al. Intravitreal triamcinolone acetonide for acute radiation papillopathy. Retina. 2006;26:537–544.
66 Finger PT. Anti-VEGF bevacizumab (Avastin) for radiation optic neuropathy. Am J Ophthalmol. 2007;143:335–338.
67 Finger PT, Chin K. Anti-vascular endothelial growth factor bevacizumab (Avastin) for radiation retinopathy. Arch Ophthalmol. 2007;125:751–756.