Objectives
• Describe the functions of a temporary pacemaker and an implantable cardioverter-defibrillator.
• Identify the signs of reperfusion in a patient undergoing fibrinolytic therapy.
Be sure to check out the bonus material, including free self-assessment exercises, on the Evolve web site at http://evolve.elsevier.com/Urden/priorities/.
A wide variety of therapeutic interventions is employed in the management of the patient with cardiovascular dysfunction. This chapter focuses on the priority interventions used to manage acute cardiovascular disorders in the critical care setting.
Temporary Pacemakers
Pacemakers are electronic devices that can be used to initiate the heartbeat when the heart’s intrinsic electrical system cannot effectively generate a rate adequate to support cardiac output. Pacemakers may be used temporarily, either supportively or prophylactically, until the condition responsible for the rate or conduction disturbance resolves.1
Indications
The clinical indications for instituting temporary pacemaker therapy are similar regardless of the cause of the rhythm disturbance that necessitates the placement of a pacemaker. The causes range from drug toxicities and electrolyte imbalances to sequelae related to acute myocardial infarction (MI) or cardiac surgery.
The Pacemaker System
A pacemaker system is a simple electrical circuit consisting of a pulse generator and a pacing lead (an insulated electrical wire) with one, two, or three electrodes.
Pacing Pulse Generator
The pulse generator is designed to generate an electrical current that travels through the pacing lead and exits through an electrode (exposed portion of the wire) that is in direct contact with the heart. This electrical current initiates a myocardial depolarization. The current then seeks to return by one of several pathways to the pulse generator to complete the circuit. The power source for a temporary external pulse generator is a standard 9-volt alkaline battery inserted into the generator.
Pacing Lead Systems
The pacing lead used for temporary pacing may be bipolar or unipolar. In a bipolar system, two electrodes (positive and negative) are located within the heart, whereas in a unipolar system, only one electrode (negative) is in direct contact with the myocardium. In both systems, the current flows from the negative terminal of the pulse generator, down the pacing lead to the negative electrode, and into the heart. The current is then picked up by the positive electrode (ground) and flows back up the lead to the positive terminal of the pulse generator.
The bipolar lead used in transvenous pacing has two electrodes on one catheter (Figure 13-1). The distal, or negative, electrode is at the tip of the pacing lead and is in direct contact with the heart, usually inside the right atrium or ventricle. Approximately 1 cm from the negative electrode is a positive electrode. The negative electrode is attached to the negative terminal, and the positive electrode is attached to the positive terminal of the pulse generator, either directly or by means of a bridging cable (see Figure 13-1, B).
Pacing Routes
Several routes are available for temporary cardiac pacing. Permanent pacing usually is accomplished transvenously, although when a thoracotomy is otherwise indicated, as in cardiac surgery, the physician may elect to insert permanent epicardial pacing wires.
Transcutaneous Pacing
Transcutaneous cardiac pacing involves the use of two large skin electrodes, one placed anteriorly and the other posteriorly on the chest, connected to an external pulse generator. It is a rapid, noninvasive procedure that nurses can perform in the emergency setting and is recommended as a primary intervention in the advanced cardiac life support (ACLS) algorithm for the treatment of symptomatic bradycardia.2 Improved technology related to stimulus delivery and the development of large electrode pads that help disperse the energy have helped reduce the pain associated with cutaneous nerve and muscle stimulation. Discomfort may still be an issue for some patients, particularly when higher energy levels are required to achieve capture. This route is typically used as a short-term therapy until the situation resolves or another route of pacing can be established.
Epicardial Pacing
The insertion of temporary epicardial pacing wires has become a routine procedure during most cardiac surgical cases. Ventricular and, in many cases, atrial pacing wires are loosely sewn to the epicardium. The terminal pins of these wires are pulled through the skin before the chest is closed. If both chambers have pacing wires attached, the atrial wires exit subcostally to the right of the sternum and the ventricular wires exit in the same region but to the left of the sternum. These wires can be removed several days after surgery by gentle traction at the skin surface with minimal risk of bleeding.3
Transvenous Pacing
Temporary transvenous endocardial pacing is accomplished by advancing a pacing electrode wire through a vein, often the subclavian or internal jugular vein, and into the right atrium or right ventricle (RV). Insertion can be facilitated through direct visualization with fluoroscopy or by the use of the standard electrocardiogram (ECG). In some cases, the pacing wire is inserted through a special pulmonary artery catheter by means of a port that exits in the right atrium or right ventricle.
Codes and Modes
Three-Letter Pacemaker Code
The Inter-Society Commission for Heart Disease (ICHD) has a standardized code for describing the various pacing modes. A three-letter code is used to describe temporary pacing modes. The first letter refers to the cardiac chamber that is paced. The second letter designates which chamber is sensed, and the third letter indicates the pacemaker’s response to the sensed event.4
Five-Letter Pacemaker Code
The five-letter pacemaker code contains the three-letter code categories plus two sections that list additional programming functions (Table 13-1).4
TABLE 13-1
POSITION I: CHAMBERS PACED | POSITION II: CHAMBERS SENSED | POSITION III: RESPONSE TO SENSING | POSITION IV: RATE MODULATION | POSITION V: MULTISITE PACING |
0 = None | 0 = None | 0 = None | 0 = None | 0 = None |
A = Atrium | A = Atrium | T = Triggered | R = Rate Modulation | A = Atrium |
V = Ventricle | V = Ventricle | I = Inhibited | V = Ventricle | |
D = Dual (A + V) | D = Dual (A + V) | D = Dual (T + I) | D = Dual (A + V) |
Modified from Bernstein AD, et al: The Revised NASPE/BPEG generic code for antibradycardia, adaptive-rate and multisite pacing, Pacing Clin Electrophysiol 25(2):260, 2002.
Synchronous Pacing Modes
Synchrony implies that the pacemaker only delivers a stimulus when the heart’s intrinsic pacemaker fails to function at a predetermined rate. The most physiological of the synchronous modes are those in which the normal sequential relationship between atrial and ventricular depolarization and contraction is maintained. Atrioventricular (AV) synchrony increases the volume in the ventricle before contraction and thus improves cardiac output. When atrial-to-ventricular conduction is impaired, as during heart block, AV synchrony can be maintained through a dual-chamber (both atrial and ventricular) pacing mode.
DDD Pacing
The most physiological of the AV pacing modes is the DDD mode. In DDD pacing, atrial and ventricular leads are used for both pacing and sensing (Table 13-2). In response to sensed activity, the pacemaker inhibits the pacing stimulus. Therefore a sensed P wave in the atrium will inhibit the atrial pacing stimulus, whereas a sensed R wave in the ventricle will inhibit the ventricular pacing stimulus. DDD pacing is also described as “universal pacing” or “physiological pacing” because it most closely resembles the heart’s intrinsic conduction system.
TABLE 13-2
EXAMPLES OF TEMPORARY PACING MODES
PACING MODE | DESCRIPTION |
Asynchronous | |
AOO | Atrial pacing, no sensing |
VOO | Ventricular pacing, no sensing |
DOO | Atrial and ventricular pacing, no sensing |
Synchronous | |
AAI | Atrial pacing, atrial sensing, inhibited response to sensed P waves |
VVI | Ventricular pacing, ventricular sensing, inhibited response to sensed QRS complexes |
DVI | Atrial and ventricular pacing, ventricular sensing; both atrial and ventricular pacing are inhibited if a spontaneous ventricular depolarization is sensed |
Universal | |
DDD | Both chambers are paced and sensed; inhibited response of the pacing stimuli to sensed events in their respective chambers; triggered response to sensed atrial activity to allow for rate-responsive ventricular pacing |
VVI Pacing
The VVI mode is designed to pace the ventricle when the pacemaker does not sense an intrinsic (patient initiated) ventricular depolarization. Other names that are popularly used for this mode include “backup pacing” or “demand pacing” (see Table 13-2). VVI pacing is necessary in specific circumstances; the classic example is symptomatic bradycardia with atrial fibrillation. Because a fibrillating atrium is impossible to pace, one effective intervention is to use VVI pacing to maintain ventricular function.
Asynchronous Pacing Modes
Fixed-rate or asynchronous pacing modes ignore the patient’s intrinsic heartbeat. These modes are uncommon with the exception of two situations. Emergency DOO or VOO pacing may be used in asystole as a lifesaving measure. These modes are sometimes used in the operating room, where electromagnetic interference (EMI) from electrocautery and other electrical equipment can interfere with normal pacemaker function.
Pacemaker Settings
The controls on all external temporary pulse generators are similar. Their functions must be thoroughly understood so that pacing can be initiated quickly in an emergency situation and troubleshooting can be facilitated if problems with the pacemaker arise.
The rate control regulates the number of impulses that can be delivered to the heart per minute. The rate setting depends on the physiological needs of the patient, but it usually is maintained between 60 and 80 beats/min.
The output dial regulates the amount of electrical current, measured in milliamperes (mA), that is delivered to the heart to initiate depolarization. The point at which depolarization occurs, called threshold, is indicated by a myocardial response to the pacing stimulus (i.e., capture). Threshold can be determined by gradually decreasing the output setting until 1 : 1 capture is lost. The output setting is then slowly increased until 1 : 1 capture is reestablished; this threshold to pace is less than 1 mA with a properly positioned pacing electrode. The output is set two to three times higher than threshold, because thresholds tend to fluctuate over time. Box 13-1 details the procedure for measuring pacing thresholds. Separate output controls for atrium and ventricle are used with a dual-chamber pulse generator.
The sensitivity control regulates the ability of the pacemaker to detect the heart’s intrinsic electrical activity. Sensitivity is measured in millivolts (mV) and determines the size of the intracardiac signal that the generator will recognize. If the sensitivity is adjusted to its most sensitive setting—a setting of 0.5 to 1.0 mV—the pacemaker can respond even to low-amplitude electrical signals coming from the heart. Turning the sensitivity to its least sensitive setting (i.e., adjusting the dial to a setting of 20 mV or to the area labeled async) results in inability of the pacemaker to sense any intrinsic electrical activity and causes the pacemaker to function at a fixed rate. A sense indicator (often a light) on the pulse generator signals each time intrinsic cardiac electrical activity is sensed. A pulse generator may be designed to sense atrial activity, ventricular activity, or both. Box 13-2 describes the procedure for measuring sensitivity.
The AV interval control (available only on dual-chamber generators) regulates the time interval between the atrial and ventricular pacing stimuli. This interval is analogous to the PR interval that occurs in the intrinsic ECG. Proper adjustment of this interval to between 150 and 250 milliseconds (msec) preserves AV synchrony and permits maximal ventricular stroke volume and enhanced cardiac output.
Temporary DDD pacemakers have several other digital controls that are unique to this type of temporary pulse generator. The lower rate, or base rate, determines the rate at which the generator will pace when intrinsic activity falls below the set rate of the pacemaker. The upper rate determines the fastest ventricular rate the pacemaker will deliver in response to sensed atrial activity. This setting is needed to protect the patient’s heart from being paced in response to rapid atrial dysrhythmias. The pulse width, which can be adjusted from 0.05 to 2 msec, controls the length of time that the pacing stimulus is delivered to the heart. There is also an atrial refractory period, programmable from 150 to 500 msec, which regulates the length of time, after a sensed or paced ventricular event, during which the pacemaker cannot respond to another atrial stimulus. An emergency button is also available on most models to allow for rapid initiation of asynchronous (DOO) pacing during an emergency.
On all temporary pacemakers, an on/off switch is provided with a safety feature that prevents the accidental termination of pacing. Also a “lock” feature is used to prevent unintended changes to the prescribed settings.
Pacing Artifacts
All patients with temporary pacemakers require continuous ECG monitoring. The pacing artifact is the spike that is seen on the ECG tracing as the pacing stimulus is delivered to the heart. A P wave is visible after the pacing artifact if the atrium is being paced (Figure 13-2, A). Similarly, a QRS complex follows a ventricular pacing artifact (see Figure 13-2, B). With dual-chamber pacing, a pacing artifact precedes both the P wave and the QRS complex (see Figure 13-2, C).
Pacemaker Malfunctions
Pacing Abnormalities
Most pacemaker malfunctions can be categorized as abnormalities of either pacing or sensing. Immediate and accurate recognition of these malfunctions is critically important in a pacemaker-dependent individual.
Failure to Pace
Failure of the pacemaker to deliver the pacing stimulus results in the disappearance of the pacing artifact on the bedside ECG monitor, even though the patient’s intrinsic rate is less than the set rate on the pacemaker (Figure 13-3). This can occur either intermittently or continuously and can be attributed to failure of the pulse generator or its battery, a loose connection between the various components of the pacemaker system, broken lead wires, or stimulus inhibition as a result of EMI. Tightening connections, replacing the batteries or the pulse generator itself, or removing the source of EMI may restore pacemaker function.

A, Patient with a transvenous pacemaker is turned onto the left side. Immediately, there is a failure to pace (i.e., loss of pacer artifacts on the electrocardiogram). The patient’s heart rate is extremely low without pacemaker support. B, The nurse turns the patient onto the right side, the transvenous electrode floats into contact with the right ventricular wall, and pacing is resumed. (From Kesten KS, Norton CK: Pacemakers: patient care, troubleshooting, rhythm analysis, Baltimore, 1985, Resource Applications.)
Failure to Capture
If the pacing stimulus fires but fails to initiate a myocardial depolarization, a pacing artifact will be present but will not be followed by the expected P wave or QRS complex, depending on the chamber being paced (Figure 13-4). This “loss of capture” most often can be attributed either to displacement of the pacing electrode or to an increase in the threshold (electrical stimulus necessary to elicit a myocardial depolarization) as a result of medications, metabolic disorders, electrolyte imbalances, or fibrosis or myocardial ischemia at the site of electrode placement. In many cases, increasing the output (mA) may elicit capture. For transvenous leads, repositioning the patient to the left side may improve lead contact and restore capture.1
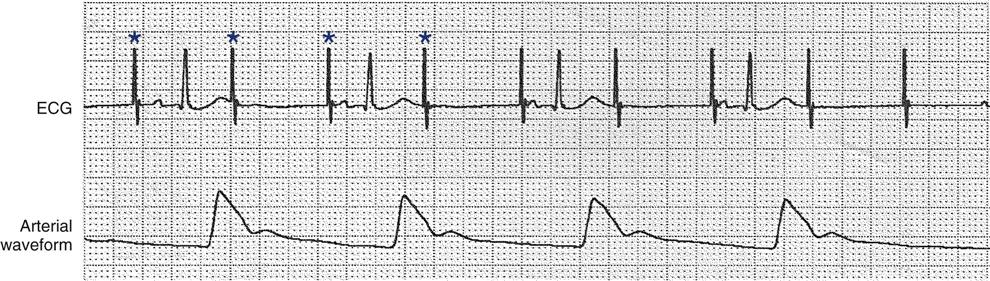
Atrial pacing and capture occur after pacer artifacts (spikes) 1, 3, 5, and 7. The remaining pacer artifacts fail to capture the tissue, resulting in loss of the P wave, no conduction to the ventricles, and no arterial waveform. Each asterisk represents a pacemaker impulse.
Pacing can occur at inappropriate rates. For example, impending battery failure in a permanent pacemaker can result in a gradual decrease in the paced rate, also referred to as rate drift. Inappropriate stimuli from a pacemaker may result in a pacemaker-mediated tachycardia. This usually is caused by sensing of inappropriate signals in a dual-chamber pacemaker that is in a trigger mode, such as DDD. Placing a magnet over the generator to transiently suspend sensing can terminate the tachycardia.1
Sensing Abnormalities
Sensing abnormalities include both undersensing and oversensing.
Undersensing
Undersensing is the inability of the pacemaker to sense spontaneous myocardial depolarizations. Undersensing results in competition between paced complexes and the heart’s intrinsic rhythm. This malfunction is manifested on the ECG by pacing artifacts that occur after or are unrelated to spontaneous complexes (Figure 13-5). Undersensing can result in the delivery of pacing stimuli into a relative refractory period of the cardiac depolarization cycle. A ventricular pacing stimulus delivered into the downslope of the T wave (R-on-T phenomenon) is a real danger with this type of pacer aberration, because it may precipitate a lethal dysrhythmia. The nurse must act quickly to determine the cause and initiate appropriate interventions. Frequently the cause can be attributed to inadequate wave amplitude (height of the P or R wave). If this is the case, the situation can be promptly remedied by increasing the sensitivity by moving the sensitivity dial toward its lowest setting. Other possible causes include inappropriate (asynchronous) mode selection, lead displacement or fracture, loose cable connections, and pulse generator failure.
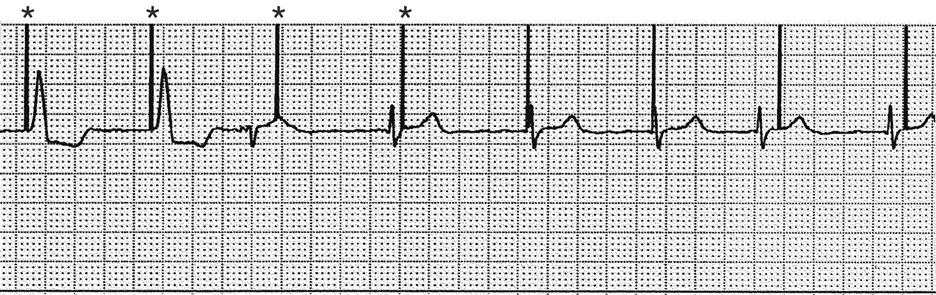
After the first two paced beats, a series of intrinsic beats occur; the pacemaker unit fails to sense these intrinsic QRS complexes. These pacer artifacts do not capture the ventricle because they occur during the refractory period of the cardiac cycle. Each asterisk represents a pacemaker impulse.
Oversensing
Oversensing occurs as a result of inappropriate sensing of extraneous electrical signals that leads to unnecessary triggering or inhibition of stimulus output, depending on the pacer mode. The source of these electrical signals can range from tall peaked T waves to EMI in the critical care environment. Because most temporary pulse generators are programmed in demand modes, oversensing results in unexplained pauses in the ECG tracing as the extraneous signals are sensed and inhibit pacing. Often, moving the sensitivity dial toward 20 mV (less sensitive) stops the pauses. With permanent pacemakers, a magnet may be placed over the generator to restore pacing in an asynchronous mode until appropriate changes in the generator settings can be programmed.
Medical Management
The physician determines the pacing route based on the patient’s clinical situation. Transcutaneous pacing typically is used in emergent situations until a transvenous lead can be secured. If the patient is undergoing heart surgery, epicardial leads may be electively placed at the end of the operation. The physician places the transvenous or epicardial pacing lead or leads, repositioning them as needed to obtain adequate pacing and sensing thresholds. Decisions regarding lead placement may later limit the pacing modes available to the clinician. For example, to perform dual-chamber pacing, both atrial and ventricular leads must be placed. In an emergency, however, interventions are focused on establishing ventricular pacing, and atrial lead placement may not be feasible. After lead placement, the initial settings for output and sensitivity are determined, the pacing rate and mode are selected, and the patient’s response to pacing is evaluated.
Nursing Management
Nursing priorities in the care of a patient with a temporary pacemaker can be combined into four primary areas: (1) preventing pacemaker malfunction, (2) protecting against microshock, (3) monitoring for complications, and (4) providing patient education.
Preventing Pacemaker Malfunction
Continuous ECG monitoring is essential to facilitate prompt recognition of and appropriate intervention for pacemaker malfunction. Proper care of the pacing system can prevent pacing abnormalities.
The temporary pacing lead and bridging cable must be properly secured to the body with tape to prevent accidental displacement of the electrode, which can result in failure to pace or sense. The external pulse generator can be secured to the patient’s waist with a strap or placed in a telemetry bag for the mobile patient. If the patient is on a regimen of bed rest, the pulse generator can be suspended with twill tape from an IV pole mounted overhead on the ceiling. This prevents tension on the lead while the patient is moved (given adequate length of bridging cable) and alleviates the possibility of accidental disconnection or dropping of the pulse generator.
The nurse inspects for loose connections between the leads and pulse generator on a regular basis.5 Replacement batteries and pulse generators must always be available on the unit. Although the battery has an anticipated life span of 1 month, it probably is sound practice to change the battery if the pacemaker has been operating continually for several days. Newer generators provide a low-battery signal 24 hours before complete loss of battery function occurs to prevent inadvertent interruptions in pacing. The pulse generator must always be labeled with the date on which the battery was replaced.
Protecting against Microshock
It is important to be aware of all sources of EMI within the critical care environment that may interfere with the pacemaker’s function. Sources of EMI in the clinical area include electrocautery, defibrillation current, radiation therapy, magnetic resonance imaging devices, and transcutaneous electrical nerve stimulation (TENS) units.3 In most cases, if EMI is suspected of precipitating pacemaker malfunction, conversion to the asynchronous mode (fixed rate) can maintain pacing until the cause of the EMI is removed.
Because the pacing electrode provides a direct, low-resistance path to the heart, the nurse takes special care while handling the external components of the pacing system to avoid conducting stray electrical current from other equipment. Even a small amount of stray current transmitted through the pacing lead could precipitate a lethal dysrhythmia. The possibility of microshock can be minimized by wearing rubber gloves when handling the pacing wires and by proper insulation of terminal pins of pacing wires when they are not in use. The latter precaution can be accomplished by the use of caps provided by the manufacturer or by improvising with a plastic syringe or section of disposable rubber glove. The wires are taped securely to the patient’s chest to prevent accidental electrode displacement. Additional safety measures include using a nonelectric or a properly grounded electric bed, keeping all electrical equipment away from the bed, and permitting the use of only rechargeable electric razors.
Monitoring for Complications
Infection at the lead insertion site is a rare but serious complication associated with temporary pacemakers. The site is carefully inspected for purulent drainage, erythema, and edema, and the patient is observed for signs of systemic infection. Site care is performed according to the institution’s policies and procedures. Although most infections remain localized, endocarditis can occur in patients with endocardial pacing leads.
During transvenous pacing insertion, a balloon at the tip of the catheter is inflated with air to facilitate transvenous insertion. Once the tip of the pacing catheter is correctly positioned within the heart chamber, the balloon is deflated. It is important that the external balloon port is labeled to avoid inadvertent access.5 A rare complication associated with transvenous pacing is myocardial perforation, which can result in rhythmic hiccoughs or cardiac tamponade.
Providing Patient Education
Patient teaching for the person with a temporary pacemaker emphasizes the prevention of complications. The patient is instructed not to handle any exposed portion of the lead wire and to notify the nurse if the dressing over the insertion site becomes soiled, wet, or dislodged. When epicardial pacemaker wires are removed following cardiac surgery, explain that a pulling sensation may be experienced.6 The patient is advised not to use any electrical devices brought in from home that could interfere with pacemaker functioning.
Patients with temporary transvenous pacemakers need to be taught to restrict movement of the affected extremity to prevent lead displacement.
Permanent Pacemakers
More than 195,000 permanent pacemakers are implanted annually in the United States, and critical care nurses are likely to encounter these devices in their clinical practice.7 These pacemakers were originally designed to provide an adequate ventricular rate in patients with symptomatic bradycardia. Today, the goal of pacemaker therapy is to simulate, as much as possible, normal physiological cardiac depolarization and conduction. Sophisticated generators permit rate-responsive pacing, affecting responses to sensed atrial activity (DDD) or to a variety of physiological sensors (body motion, QT interval, minute ventilation). For patients who do not have a functional sinus node that can increase their heart rate, rate-responsive pacemakers have been shown to improve exercise capacity and quality of life.8 Table 13-3 describes the types of rate-responsive pacing modes in clinical use.
TABLE 13-3
PERMANENT PACEMAKER RATE-RESPONSE PACING MODES
PULSE GENERATOR | DESCRIPTION |
AAIR | AAI features plus rate-responsive pacing; used for patients with a symptomatic bradycardia who have a paceable atrium and intact AV conduction |
VVIR | VVI features plus rate-responsive pacing; used for patients with an atrium that is unpaceable as a result of chronic atrial fibrillation or other atrial dysrhythmia |
DDDR | DDD features plus rate-responsive pacing; used for patients with symptomatic bradycardia in which the atrium is paceable but AV conduction is, or may become, unreliable |
The concept of physiological pacing continues to evolve, because studies have indicated that pacing initiated from the RV apex—even in a dual-chamber mode—may promote heart failure in patients with permanent pacemakers.1 This has prompted further research to identify alternative sites for pacing and modes that can maximize intrinsic AV conduction and minimize ventricular pacing.8
Technological advances in the computer industry have had a major impact on today’s permanent pacemakers. Microprocessors have allowed for the development of increasingly smaller generators despite the incorporation of more complex features. Today’s pacemaker generators are smaller, more energy-efficient, and more reliable than previous models. A new trend in permanent pacemakers is to use these devices as nonpharmacological therapy in treatment of heart failure.
Cardiac Resynchronization Therapy
About one third of patients with severe heart failure have ventricular conduction delays (prolonged QRS duration or bundle branch block). These conduction delays have been shown to create a lack of synchrony between the contractions of the LV and RV. The hemodynamic consequences of this dyssynchrony include impaired ventricular filling with decreased ejection fraction, cardiac output, and mean arterial pressure.9 Cardiac resynchronization therapy (CRT) uses atrial pacing plus stimulation of both the LV and RV (biventricular pacing), in an attempt to optimize atrial and ventricular mechanical activity. The CRT device uses three pacing leads, one each in the right atrium and the RV and a specially designed transvenous lead that is inserted through the coronary sinus to pace the LV.9 Because many patients with heart failure are also at risk for sudden cardiac death, biventricular pacing is available on some implantable cardioverter defibrillators (ICDs). A number of clinical trials have shown symptomatic and structural cardiac improvement with this new therapy.10
Atrial Arrhythmia Suppression
There is a growing incidence of atrial fibrillation, and atrial pacing has been proposed as a possible preventative therapy for this dysrhythmia in selected patients. Atrial pacing in patients with bradycardia has been shown to lower the recurrence of atrial fibrillation, especially compared with ventricular pacing.11 Most pacemakers can be programmed to mode switch to a non-P wave tracking mode if rapid atrial rates are sensed.1 Strategies for patients with paroxysmal atrial fibrillation, which include pacing both atriums (bi-atrial pacing) and transiently pacing the atrium at a rate higher than the patient’s intrinsic sinus rate, require further study.12
Medical Management
Permanent pacemakers may be implanted with the patient under local anesthesia in the operating room or in the cardiac catheterization laboratory. Transvenous leads usually are inserted through the cephalic or subclavian vein and positioned in the right atrium or RV, or both, with fluoroscopic guidance. Satisfactory lead placement is determined by testing the stimulation and sensitivity thresholds with a pacing system analyzer. The leads are then attached to the generator, which is inserted into a surgically created pocket in the subcutaneous tissue below the clavicle.
Nursing Management
Nursing management for patients after permanent pacemaker implantation includes monitoring for complications related to insertion and for pacemaker malfunction. Postoperative complications are rare but include cardiac perforation and tamponade, pneumothorax, hematoma, lead displacement, and infection.13,14
Identification of permanent pacemaker malfunction is the same as that described previously for temporary pacemakers. To evaluate pacemaker function, the nurse must know at least the pacemaker’s programmed mode of pacing and the lower rate setting. With permanent pacemakers, settings are adjusted noninvasively through a specialized programmer that uses pulsed magnetic fields or an RF signal. If a pacemaker problem is suspected, ECG strips are obtained, and the physician is notified so that the pacemaker settings can be reprogrammed as needed. If the patient experiences symptoms of decreased cardiac output, he or she may require support with temporary transcutaneous pacing until the problem is corrected.
Critical care nurses also may be involved in monitoring patients with permanent pacemakers after discharge. Some units are equipped with transtelephonic monitoring equipment that allows patients to transmit information over the telephone from a monitoring device in their home. Transmission of the patient’s ECG can provide information to confirm proper pacemaker function (capture and sensing) and to determine battery status (rate). Newer technology that uses an Internet-based remote monitoring system for pacemaker follow-up may soon replace standard transtelephonic ECG evaluation.15
The foregoing discussion provides an introduction to the basic concepts of pacemaker therapy. However, the nurse who cares for patients with permanent or temporary pacemakers must be familiar with even the most sophisticated modes of pacemaker function. Only by keeping pace with current technology can the nurse accurately interpret pacer function and thereby safely and effectively care for patients with pacemakers.
Implantable Cardioverter Defibrillators
An implantable cardioverter defibrillator (ICD) is an electronic device that is used in the treatment of tachydysrhythmias. The ICD is capable of identifying and terminating life-threatening ventricular dysrhythmias; 114,000 are implanted annually in the U.S.7 Initially, an ICD was recommended only for patients who had survived an episode of cardiac arrest caused by ventricular fibrillation (VF) or ventricular tachycardia (VT). Later, a number of clinical trials compared ICD therapy for such secondary prevention of sudden cardiac death with antidysrhythmic drug therapy and found improved survival with the ICD.1 As a result, ICD use was expanded to include primary prevention of sudden cardiac death in patients with coronary artery disease (CAD), previous MI, or LV dysfunction in whom VT or VF was inducible during EPS. Trials have shown improved survival with ICD implantation in high-risk patients (i.e., those with previous MI and an ejection fraction <35%) even without evidence of VT or VF on an EPS.10 These results have further increased the number of patients who receive an ICD.
The ICD System
The ICD system contains (1) sensing electrodes to recognize the dysrhythmia and (2) defibrillation electrodes or patches that are in contact with the heart and can deliver a shock. These electrodes are connected to a generator that is surgically placed in the subcutaneous tissue of the pectoral region in the upper chest (Figure 13-6). The early-model generators could defibrillate or cardiovert only lethal dysrhythmias. The current generation of devices delivers a tiered therapy, with options for programmable antitachycardia pacing, bradycardia backup pacing, low-energy cardioversion, and high-energy defibrillation. With tiered therapy, antitachycardia pacing is used as the first line of treatment in some cases of VT. If the VT can be pace-terminated successfully, the patient will not receive a shock from the generator and may not even realize that the ICD terminated the dysrhythmia. If programmed bursts of pacing do not terminate the VT, the ICD will cardiovert the rhythm. If the dysrhythmia deteriorates into VF, the ICD is programmed to defibrillate at a higher energy. If the dysrhythmia terminates spontaneously, the device will not discharge. Occasionally, the electrical rhythm may deteriorate to asystole or a slow idioventricular rhythm; in such cases, the bradycardia backup pacing function is activated.
ICDs are dual-chamber devices with leads in both atria and ventricles. The atrial leads allows for dual-chamber pacing to optimize hemodynamic performance and atrial sensing to more accurately discriminate between atrial and ventricular tachycardias and to decrease the incidence of inappropriate shocks. Implantable defibrillators with atrial capabilities may also be used to deliver therapies such as cardioversion or antitachycardia pacing to patients with atrial tachydysrhythmias, but their use may be limited because of the painful nature of the shocks.12 ICDs may also incorporate triple-lead systems (leads in one atrium and both ventricles) to allow for CRT and defibrillation in one device. Other developments in ICD technology include improved diagnostic and telemetry functions, such as the ability to provide real-time electrograms obtained from the ICD electrodes or the ability to perform remote device interrogation by telephone or Internet.16
ICD Insertion
The ICD has progressed in both programmable functions and insertion design. Transvenous electrode leads are inserted into the subclavian vein and advanced into the right side of the heart, where contact with the endocardium is achieved. The endocardial leads are used for sensing, pacing, cardioversion, and defibrillation, and are connected to the generator by tunneling through the subcutaneous tissue. Technical advances and the development of smaller ICDs have made it feasible to implant these devices in the pectoral position, similar to permanent pacemakers.
Medical Management
Medical management in the ICD patient begins before implantation with a thorough evaluation of the patient’s dysrhythmia and underlying cardiac function. Patients at risk for sudden cardiac death (SCD) undergo an electrophysiology study to determine the origin of the dysrhythmia and the effect of antidysrhythmic agents in suppressing or altering the rate of the dysrhythmia. Further assessment of cardiac status is made to determine whether additional interventions (e.g., cardiac surgery, angioplasty or stent) are indicated to improve cardiac function and decrease SCD risk.
ICD Programming
An electrophysiologist performs the initial programming of the device at the time of implantation. During implantation, defibrillation threshold measurements are obtained. This involves inducing the dysrhythmia and then evaluating the device’s ability to terminate it. After it has been determined that the ICD functions appropriately, further follow-up is conducted on an outpatient basis to monitor the number of discharges and the battery life of the device.
Nursing Management
It is important to know the type of ICD implanted, how the device functions, and whether it is activated (i.e., on). If the patient experiences a shockable rhythm, the nurse should be prepared to defibrillate in the rare event that the device fails. During external defibrillation, the paddles or patches should not be placed directly over the ICD generator. Standard paddle placement may need to be altered in patients with ICDs to achieve successful defibrillation.2 Most patients continue to take antidysrhythmic medications to decrease the number of shocks required and to slow the rate of the tachycardia. Complications associated with the permanent ICD include infection from the implanted system,14 broken leads, and sensing of supraventricular tachydysrhythmias resulting in unneeded discharges.
Providing Patient Education
To facilitate a positive psychological adjustment to the ICD, patient education about the device is vital.17,18 Preoperative teaching for the ICD patient includes information about how the device works and what to expect during the implantation procedure. After implantation, education is focused on aspects of living with an ICD. Patients need information pertaining to scheduled device follow-up and instructions about what to do if they experience a shock. Many institutions have successfully used family support groups for this patient population.
Fibrinolytic Therapy
Fibrinolytic therapy is an important clinical intervention for the patient experiencing acute ST-elevation myocardial infarction (STEMI). Before the introduction of fibrinolytic agents, medical management of acute MI was focused on decreasing myocardial oxygen demands to minimize myocardial necrosis and preserve ventricular function. Today, efforts to limit the size of the infarction are directed toward timely reperfusion of the jeopardized myocardium through restoration of blood flow in the culprit vessel (the open artery theory). Two options are available for opening the artery—fibrinolytics and mechanical intervention. Although mechanical catheter-based intervention has been proven to yield better outcomes when performed in a timely fashion, only 25% of U.S. hospitals are estimated to have this capability.19 For this reason, fibrinolytic therapy continues to play a major role in the treatment of acute MI.
The use of fibrinolytic therapy is predicated on the theory that the significant event in acute coronary syndromes (e.g., unstable angina, acute MI) is the rupture of an atherosclerotic plaque with thrombus formation (Figure 13-7). The thrombus, which is composed of aggregated platelets bound together with fibrin strands, occludes the coronary artery, depriving the myocardium of oxygen previously supplied by that artery. The administration of a fibrinolytic agent results in lysis of the acute thrombus, resulting in recanalization, or opening, of the obstructed coronary artery and restoration of blood flow to the affected tissue. After perfusion is restored, adjunctive measures are taken to prevent further clot formation and repeat occlusion.
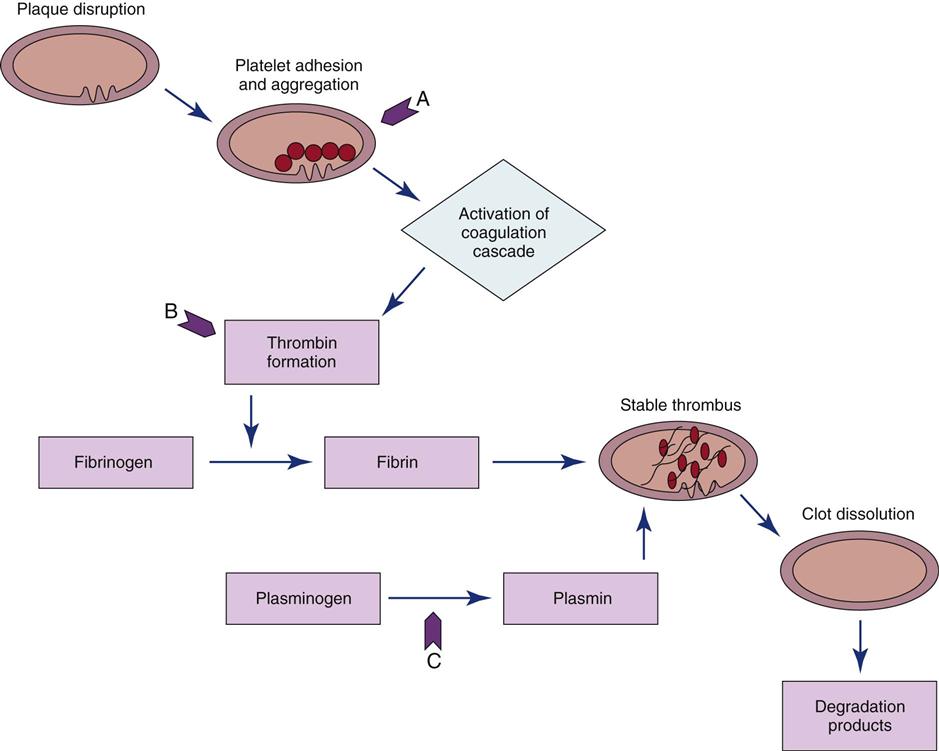
A, Site of action of antiplatelet agents such as aspirin and glycoprotein IIb/IIIa inhibitors. B, Heparin bonds with antithrombin III and thrombin to create an inactive complex. C, Fibrinolytic agents convert plasminogen to plasmin, an enzyme responsible for degradation of fibrin clots.
Eligibility Criteria
Inclusion Criteria
Certain criteria have been developed, based on research findings, to determine the patient population that would most likely benefit from the administration of fibrinolytic therapy. Patients with recent onset of chest pain (<12 hours’ duration) and persistent ST elevation (>0.1 mV in two or more contiguous leads) are considered candidates for fibrinolytic therapy.20 Patients who present with bundle branch blocks that may obscure ST-segment analysis and a history suggesting an acute MI are also considered candidates for therapy. The goal of therapy is to administer fibrinolytic therapy within 30 minutes after presentation at the hospital, described as “door-to-needle” time. Time is crucial because early reperfusion yields the greatest benefit.21
Exclusion criteria are usually based on the increased risk of bleeding incurred from the use of fibrinolytics. Patients who have stable clots that might be disrupted by fibrinolytic therapy (recent surgery, trauma, or stroke) usually are not considered candidates for fibrinolytic therapy. Other selection criteria for the use of fibrinolytic therapy are presented in Box 13-3.
Exclusion Criteria
Currently, fibrinolytic therapy is not indicated for patients with unstable angina or non-ST-elevation myocardial infarction (NSTEMIs). It is believed that these conditions result from plaque rupture with the formation of an only partially occlusive thrombus. Fibrinolysis breaks up the clot and releases thrombin, and this can paradoxically increase the material necessary for further thrombosis.22 Instead, these patients are treated with antiplatelet agents (e.g., aspirin, clopidogrel, glycoprotein IIb/IIIa inhibitors) and antithrombin drugs (e.g., heparin).
Fibrinolytic Agents
Four fibrinolytic agents are currently available for intravenous treatment of acute STEMI. All of these agents stimulate lysis of the clot by converting inactive plasminogen to plasmin, an enzyme responsible for degradation of fibrin. Streptokinase (SK) and urokinase were the first generation of fibrinolytic agents and had their primary effect on circulating plasminogen. Urokinase availability has been limited because of manufacturing problems. Newer fibrinolytic agents (e.g., alteplase, reteplase, tenecteplase) have a greater effect on clot plasminogen than on circulating plasminogen and are described as clot selective. A comparison of currently approved fibrinolytic agents is provided in Table 13-4.
TABLE 13-4
PHARMACOLOGICAL MANAGEMENT: FIBRINOLYTIC AGENTS FOR USE IN ACUTE MYOCARDIAL INFARCTION
DRUG | DOSAGE | MECHANISM OF ACTION | SPECIAL CONSIDERATIONS |
Clot-Specific | |||
tPA (alteplase) | IV: 100 mg over 90 min with the first 15 mg given as a bolus | Binds to fibrin at the clot and promotes activation of plasminogen to plasmin | Short half-life, so heparin is usually given with the drug as a bolus and afterward as an infusion. Aspirin is begun with administration of the drug and continued daily. |
rPA (reteplase) | IV: 10 units given as a bolus over 2 min, repeated in 30 min | Binds to fibrin at the clot and promotes activation of plasminogen to plasmin | Heparin is started with administration of the drug and continued for 24 hours. Aspirin is begun with administration of the drug and continued daily. |
TNKase (tenecteplase) | IV: 30-50 mg based on body weight, given as a single bolus | Binds to fibrin at the clot and promotes activation of plasminogen to plasmin | Heparin is started with administration of the drug. Aspirin is begun with administration of the drug and continued daily. |
Non-Clot-Specific | |||
SK (streptokinase) | IV: 1.5 million units given over 60 min | Catalyzes the conversion of plasminogen to plasmin, which causes lysis of fibrin; has systemic lytic effects | May cause allergic reactions and hypotension. Heparin may be administered IV or SQ. Aspirin is begun with administration of the drug and continued daily. |
Because patients with an area of plaque disruption are still at risk for clot formation and reocclusion, fibrinolytic therapy is used in conjunction with anticoagulants and antiplatelet agents. Current guidelines recommend that heparin be administered for a minimum of 48 hours after fibrinolytic therapy. Low-molecular-weight heparin (LMWH) is considered an acceptable alternative in patients younger than 75 years who have adequate kidney function. Antiplatelet therapy with clopidogrel is recommended for 14 days, and aspirin should be continued indefinitely20 (Table 13-5).
TABLE 13-5
PHARMACOLOGICAL MANAGEMENT: ANTICOAGULANTS
Outcomes of Fibrinolytic Therapy
The benefit of fibrinolytic therapy correlates with the degree of restoration of normal blood flow in the infarct-related artery. Coronary artery patency is defined by angiographic perfusion grades developed by the Thrombolysis in Myocardial Infarction (TIMI) study group in 1985 (Box 13-4).23 Achievement of TIMI grade 3 flow is associated with the best long-term survival. Studies also indicate that rapid restoration of normal blood flow, within 90 minutes after treatment, results in improved LV function and reduced mortality. The three fibrin-specific fibrinolytics have been shown to achieve TIMI 3 flow in 54% to 63% of patients at 90 minutes.24
The area of fibrinolytic therapy continues to evolve, and drug dose ranges and regimens are subject to change when research findings are updated. Whereas fibrinolytic agents target the fibrin portion of the clot, other treatment strategies are focusing on the platelet portion of the clot (see Figure 13-7). Clinical trials evaluating the combination of glycoprotein IIb/IIIa receptor antagonists with fibrinolytic agents (at half-dose) found outcomes equivalent to those of fibrinolytics alone, but with an increased risk for bleeding.25 There was also speculation that a planned strategy for administering fibrinolytics or glycoprotein IIb/IIIa receptor antagonists, or both, to patients who must be transported to another facility for percutaneous intervention would improve outcomes. Although promising in theory, this facilitated approach to revascularization has not been shown to be beneficial and may increase the risk for bleeding complications in some patients.26
Evidence of Reperfusion
In the cardiac catheterization laboratory, the blood flow of a vessel that has been occluded by a thrombus and then opened by fibrinolytic therapy can be observed directly under fluoroscopy. The adequacy of the flow of blood through the coronary artery is described using the standardized thrombolysis in myocardial infarction (TIMI) scale (Box 13-4).
Nursing Management
Nursing priorities for the patient receiving fibrinolytic therapy are directed toward (1) identifying candidates for reperfusion therapy, (2) observing for signs of reperfusion, (3) monitoring for signs of bleeding, and (4) providing patient education.
Identifying Candidates for Reperfusion Therapy
Nursing management of the patient undergoing fibrinolytic therapy begins with identifying potential candidates. In many institutions, checklists are used to facilitate the rapid identification of patients who are candidates for fibrinolytics. The nurse prepares the patient for fibrinolytic therapy by starting intravenous lines and obtaining baseline laboratory values and vital signs.
Observing for Signs of Reperfusion
Reperfusion of the ischemic myocardium may be observed noninvasively by several means, as follows:
Monitoring for Signs of Bleeding
The most common complication related to thrombolysis is bleeding, from the fibrinolytic therapy itself and also because patients routinely receive anticoagulation therapy for several days to minimize the possibility of rethrombosis. The nurse must continually monitor for clinical manifestations of bleeding. Mild gingival bleeding and oozing around venipuncture sites is common and not a cause of concern. Should serious bleeding occur, such as intracranial or internal bleeding, all fibrinolytic and heparin therapies are discontinued and volume expanders or coagulation factors, or both, are administered.
In addition to accurate assessment of the patient for evidence of bleeding, nursing management includes preventive measures to minimize the potential for bleeding. For example, patient handling is limited, injections are avoided if at all possible, and additional pressure is provided to ensure hemostasis at venipuncture and arterial puncture sites. Intravenous lines are placed before lytic therapy is administered. A heparin lock may be used for obtaining laboratory specimens during treatment.
Providing Patient Education
Education for the patient receiving fibrinolytic therapy includes information regarding the actions of fibrinolytic agents, with emphasis on precautions to minimize bleeding. For example, the patient is cautioned against vigorous tooth brushing and told to refrain from using straight-edge razors. Information is provided regarding ongoing risk-factor management in the prevention of atherosclerotic CAD.
Catheter Interventions for Coronary Artery Disease
During the past three decades, the use of catheter procedures to open coronary arteries blocked or narrowed by CAD has expanded dramatically. These procedures are grouped by the term percutaneous coronary intervention (PCI). Today, PCI includes percutaneous transluminal coronary angioplasty (PTCA), atherectomy, and stent implantation. Advances in device technology, along with more effective anticoagulant and antiplatelet regimens, have reduced complication rates and improved procedural outcomes.28
Indications for Catheter-Based Interventions
Indications for catheter-based interventions have been considerably broadened since the initial application of balloon angioplasty. Whereas only patients with single-vessel CAD were once considered for PTCA, patients with multivessel disease, even those who have previously undergone saphenous vein grafting, internal mammary artery (IMA) grafting, or fibrinolytic therapy for acute MI, may now be candidates for catheter intervention. Previously seen as a rescue procedure to reduce a severe stenosis that persisted after fibrinolytic therapy, PCI is now preferred as the initial method of treatment for acute MI (primary PCI) when this therapy is available in the hospital.29 See “Coronary Artery Disease” in Chapter 12.
Surgical Backup
Initially, most institutions required that patients preparing to undergo angioplasty be candidates for coronary artery bypass graft (CABG) surgery. Complications such as intimal dissection with abrupt closure of the vessel could arise during the procedure, requiring the patient to undergo emergency CABG. Today, most dissections are effectively treated with stent placement, with less than 1% of patients requiring emergency bypass surgery. As a result, most institutions use an informal surgical backup plan, such as the first available operating room. Nevertheless, the availability of cardiac surgical services on site is still recommended. The one exception is in institutions that offer PCI only for treatment of acute STEMI. In this setting, an organized plan for emergent transfer to a surgical center may be used in lieu of on-site surgical access.30
Angioplasty
PTCA involves the use of a balloon-tipped catheter that, when advanced through an atherosclerotic lesion (atheroma), can be inflated intermittently for the purpose of dilating the stenotic area and improving blood flow through it (Figure 13-8). The high inflation pressure of the balloon stretches the vessel wall, fractures the plaque, and enlarges the vessel lumen. After balloon deflation, the vessel exhibits some degree of elastic recoil, resulting in a residual stenosis of approximately 30%.31 A successful angioplasty procedure is one in which the stenosis is reduced to less than 50% of the vessel lumen diameter.30
Restenosis occurred in more than one third of patients who underwent PTCA as a solo procedure. Restenosis within the first 6 months was diagnosed when patients experienced a recurrence of anginal symptoms.32 Studies showed that restenosis was influenced by the final lumen diameter after PTCA, the severity of elastic recoil of the vessel walls in response to the balloon inflation, and the amount of intimal hyperplasia that occurred as the vessel healed over the treated area. Patient characteristics such as a history of diabetes or unstable angina were also found to increase the risk of restenosis.33 Today, PTCA is rarely used alone as an intervention, except to treat lesions in very small coronary arteries.31 Nevertheless, balloon angioplasty remains an essential technique in PCI for dilating vessels and for deploying intracoronary stents.
Atherectomy
Atherectomy is the excision and removal of the atherosclerotic plaque by cutting, shaving, or grinding. Specialized coronary catheters are used. Initially, atherectomy devices were used alone in an attempt to avoid the trauma to the vessel that was known to occur with balloon angioplasty and to more efficiently remove the atherosclerotic plaque, thereby decreasing the rate of restenosis. Later, as restenosis was also found to occur with these devices, balloon angioplasty was added as an adjunctive therapy to optimize the diameter of the vessel lumen and offset the intimal hyperplasia that occurred as a result of the procedure. Despite significant improvements in initial procedural success, atherectomy failed to significantly reduce the rate of restenosis.34 In the current era of stenting, atherectomy devices are used in less than 5% of procedures.31
Two atherectomy devices are used in PCI: directional coronary atherectomy (DCA) (Figure 13-9, A) and rotational ablation (Rotablator) (Figure 13-9, B). A randomized clinical trial showed no clinical benefit for the use of DCA in combination with stent, compared with stenting alone.35 As a result, DCA is currently used only for noncalcified lesions that involve a bifurcation of a major side branch or in the ostium of the left anterior descending (LAD) artery.28 Rotational atherectomy is used for chronic total occlusion and for calcified bifurcation lesions.28
Coronary Stents
A major development in the field of interventional cardiology has been the coronary stent prosthesis. A stent is a metal structure that is introduced into the coronary artery over a guidewire and expanded into the vessel wall at the site of the lesion (Figure 13-10). Bare metal stents were first used to treat acute or threatened vessel closure after failed PTCA.32 The stent acted as a scaffold to tack dissection flaps against the vessel wall and provided mechanical support to minimize elastic recoil. Multiple stents may be implanted sequentially within a vessel to fully cover the area of the lesion. Stents are currently the predominant form of PCI and are used in more than 90% of all interventional procedures.7 Numerous stent models are available. They are composed of various types of metal (stainless steel, titanium, cobalt chromium) and come in a variety of configurations (e.g., mesh, coil). Most stents are balloon expandable (see Figure 13-10).
Stent Thrombosis
Specific interventions are used to prevent subacute stent thrombosis. High-pressure balloon inflations within the stent are employed to fully open the stent within the vessel, so reduced anticoagulation is sufficient to maintain stent patency. Dual antiplatelet therapy (aspirin and thienopyridine) has been shown to be more important than anticoagulation in preventing stent thrombosis.36 These agents are administered before the procedure and continued at discharge.37 Two potent antiplatelet glycoprotein IIb/IIIa inhibitors, eptifibatide (Integrilin) and tirofiban (Aggrastat), also reduce the formation of intracoronary thrombosis and are used across the spectrum of interventional procedures, from PTCA to atherectomy to stenting.37 Indications and dosing of glycoprotein IIb/IIIa inhibitors are provided in Table 13-6.
TABLE 13-6
PHARMACOLOGICAL MANAGEMENT: GLYCOPROTEIN IIB/IIIA INHIBITORS
DRUG | INDICATIONS AND DOSAGE | SPECIAL CONSIDERATIONS |
Abciximab (ReoPro) | ACS: 0.25 mg/kg IVP, then 10 mcg/min until PCI | Used concomitantly with heparin and aspirin |
PCI: 0.25 mg/kg IVP, then 0.125 mcg/kg/min × 12 hr | May affect platelet function for up to 48 hours after infusion | |
Eptifibatide (Integrilin) | ACS: IV bolus of 180 mcg/kg followed by an infusion of 2 mcg/kg/min for up to 72 hours; if the patient undergoes PCI, the infusion should be continued up to hospital discharge, or for up to 18 to 24 hours after the procedure, whichever comes first, and continued for 24 hours after the procedure, for a total of 96 hours | Concomitant heparin and aspirin may be administered Platelet function returns to baseline within 6-8 hours Dosage should be reduced in patients with severe renal dysfunction |
PCI: 180 mcg/kg IVP immediately before PCI followed by a continuous infusion of 2.0 mcg/kg/min and a second 180-mcg/kg bolus 10 minutes after the first bolus. Infusion should be continued for a minimum of 12 hours | ||
Tirofiban (Aggrastat) | ACS (with or without PCI): 0.4 mcg/kg/min for 30 min, then continued at 0.1 mcg/kg/min for 48-108 hours after ACS or 12-24 hours post-procedure for patients undergoing PCI | Administered in combination with heparin for patients undergoing PCI Platelet function returns to baseline within 4-8 hours Dosage should be reduced in patients with severe kidney dysfunction |