Cardiovascular Anatomy and Physiology
Anatomy
Macroscopic Structure
Structures of the Heart
The heart is situated in the anterior thoracic cavity, just behind the sternum (Fig. 12-1). Several important structures, including the esophagus, the aorta, the vena cava, and the vertebral column, are located behind the heart. The position of the heart within the chest cavity is such that the chambers normally described as “right” and “left” are, in fact, anterior and posterior.1 The right ventricle constitutes the majority of the anterior surface (closest to the chest wall) and also the inferior surface (directly above the diaphragm). The left ventricle makes up the anterolateral (front and side) and posterior surfaces. The base of the heart is superior (atrial and great vessel level), and the tip (apex) is inferior (ventricular level), above the diaphragm. The base of the heart includes not only the superior portion of the heart itself but also the roots of the aorta, the vena cava, and pulmonary vessels.
The increasing use of thoracic computed tomography in critical care has highlighted the anatomic inaccuracy of the terms right ventricle and left ventricle because these terms do not relate to the position of the heart in the chest when described in the standard anatomic position (i.e., upright and facing the observer).1–3 Despite discussion of these differences, the impetus to change the traditional nomenclature is lacking.
Layers of the Heart
Pericardium.
The heart and the origins of the great vessels are surrounded and enclosed by the pericardium. The outermost fibrous pericardium is a thick envelope that is tough and inelastic. Ligaments anchor the outer pericardium to the diaphragm and the great vessels such that the heart is maintained in a fixed position within the thoracic cavity. The pericardium also provides a physical barrier to infection. Inside the fibrous layer is an inner, double-walled membrane described as the serous pericardium.4 The two layers are labeled “parietal” and “visceral.” The parietal pericardium adheres to the tough, outer pericardium. The visceral pericardium is flexible, adheres directly to the heart, and folds with the surface contours of the heart. The pericardial cavity is the potential space between the visceral and parietal layers.4,5 The space between these two layers normally contains a small amount of pericardial fluid, which is secreted, resorbed, and serves as a lubricant between the layers so that the heart can move without friction.5 The fibrous, outer pericardial sac is noncompliant and unable to adapt to rapid increases in pericardial fluid.5 For example, blood can collect in this sac in cardiac tamponade, or serum can collect in pericardial effusion. If the fluid collection in the sac impinges on ventricular filling, ventricular ejection, or coronary artery perfusion, removal of the excess pericardial fluid may be necessary, a procedure known as pericardiocentesis.5 The phrenic nerve provides sensory fibers to the pericardium. The pain that accompanies pericardial effusion and cardiac tamponade is sensed via phrenic nerve innervation.5
Epicardial Fat.
In adults, a layer of adipose tissue is typically present beneath the visceral pericardium and may surround the heart. This epicardial fat accumulates along the routes of the major coronary arteries and veins. Autopsy data indicate that epicardial fat increases until age 20 to 40 years, but thereafter, the quantity does not depend on age.6 Epicardial fat covers 80% of the surface of the heart and constitutes about 20% of the total weight of the heart, with the largest quantity positioned over the right ventricle.6 If a person is overweight or obese with a large quantity of visceral and subcutaneous adipose tissue, usually more epicardial fat is present.7 Because obesity is endemic in modern society, epicardial fat is receiving more attention. Epicardial fat is hypothesized to increase the risk of coronary artery disease (CAD) because it contains smaller adipocytes compared with other fat deposits, has a different fatty acid composition, and has a higher protein content. This composition facilitates the infiltration of free fatty acids and adipokines into coronary arteries.8 The fibrous, outer pericardium does not contain any fat deposits.
Myocardium.
The next layer of the heart is the myocardium, a thick, muscular layer. This layer includes all of the atrial and ventricular muscle fibers necessary for contraction. The fibers of the myocardium do not have the same thickness throughout the ventricular walls. The left ventricle is much thicker than the right ventricle or the atria. The fibers are organized in such a manner that the force of contraction is most efficient in ejecting blood toward the outflow tracts in a wringing motion from the apex toward the base (Fig. 12-2).9 The myocardium is the muscle that is damaged by a “heart attack” or transmural myocardial infarction (MI).
Cardiac Chambers
The human heart has four chambers: the left and right atria and the left and right ventricles. The atria are thin-walled and normally low-pressure chambers. They function to receive blood from the vena cavae and pulmonary arteries and to pump blood into their respective ventricles. Atrial contraction, also known as atrial kick, contributes approximately 20% of blood flow to ventricular filling; the other 80% occurs passively during diastole. The ventricles are the primary pumping chambers of the heart. The healthy left ventricle is about 10 to 13 mm thick, and the interior chamber appears round in cross-section.10 The healthy right ventricle is approximately 3 mm thick, appears to have a triangular shape when viewed from the side, and has a crescent shape when viewed in cross-section (Fig. 12-3). The right ventricle pumps blood into the low-pressured pulmonary circulation, which has a normal mean pressure of approximately 15 mm Hg. The left ventricle must generate tremendous force to eject blood into the aorta (normal mean pressure of approximately 100 mm Hg). Because of left ventricular wall thickness and the large force it must generate, the left ventricle is considered the major pump of the heart. When the left ventricular muscle is damaged from cardiomyopathy or infarction, the effective pumping pressure is diminished, leading to increased left atrial pressure, pulmonary vasculature congestion, and ultimately, systemic venous congestion.
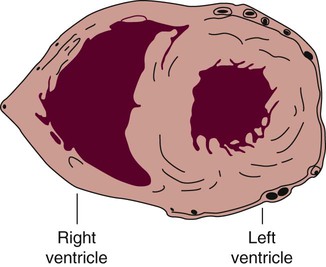
The right ventricle forms the greater part of the anterior surface of the heart, and the wall of the left ventricle is three times as thick as the wall of the right ventricle. (From Quaal S. Comprehensive Intraaortic Balloon Pumping. 2nd ed. St. Louis, MO: Mosby; 1993.)
Cardiac Valves
Cardiac valves are composed of flexible, fibrous tissue. A normal valve has the translucent appearance of a rose petal. The valve structure allows blood to flow in only one direction. The opening and closing of the valves depends on the relative pressure gradients on either side of the valve. The four cardiac valves lie in an oblique plane of collagen described as the fibrous skeleton. Four adjacent rings of connective tissue contain and support the cardiac valves (Fig. 12-4).
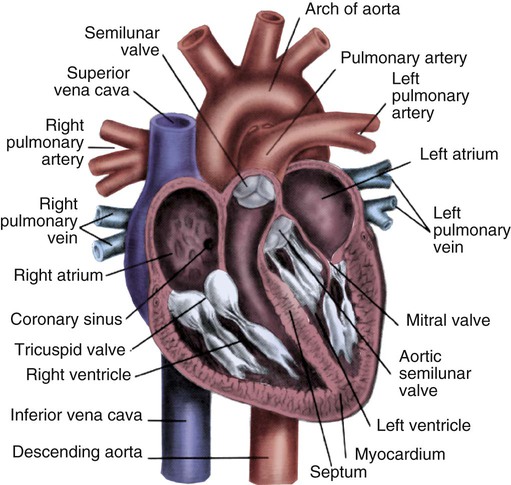
Note the position of the four cardiac valves. (From Thompson JM, et al. Mosby’s Clinical Nursing. 5th ed. St. Louis, MO: Mosby; 2002.)
Atrioventricular Valves.
The two atrioventricular (AV) valves are named for their location between the atria and the ventricles. These are the tricuspid valve on the right and the mitral valve on the left. The mitral valve is described as having two leaflets, although the “leaflets” are a continuous, noninterrupted structure.11 The AV valves are open during ventricular diastole (filling) and prevent backflow of blood into the atria during ventricular systole (contraction). The chordae tendineae and papillary muscles, which attach to the tricuspid and mitral valves, give the valves stability and prevent valve leaflet eversion during systole (Fig. 12-5). Papillary muscles arise from the ventricular myocardium and derive their blood supply from the coronary arteries. Each papillary muscle gives rise to approximately 4 to 10 main chordae tendineae, which divide into increasingly finer cords as they approach and attach to the valve leaflets. The chordae tendineae are fibrous, avascular structures covered by a thin layer of endocardium. Dysfunction of the chordae tendineae or of a papillary muscle may cause incomplete closure of an AV valve, which results in backflow of blood into the atrium. Degenerative disease of the valve leaflets is another cause of regurgitation of blood into the atrium.12 Valvular regurgitation produces a murmur that can be auscultated with a stethoscope.
Semilunar Valves.
The semilunar valves are pulmonic and aortic valves. Each valve has three cuplike leaflets (Fig. 12-6). These valves separate the ventricles from their respective outflow arteries (Table 12-1). During ventricular systole (contraction), semilunar valves open, allowing blood to flow out of the ventricles. As systole ends and the pressure in the outflow arteries exceeds that of the ventricles, the semilunar valves close, preventing blood regurgitation back into the ventricles. In most individuals, the aortic valve has three leaflets. In less than 1% of the population, it is bicuspid (a two-leaflet valve), which increases susceptibility to aortic valve failure over time.13,14 Aortic valve dysfunction, from any cause, not only affects the valve leaflets but also pathologically alters the shape of the left ventricle.
TABLE 12-1
CARDIAC VALVES AND THEIR LOCATIONS
VALVE | TYPE | SITUATED BETWEEN |
Tricuspid | Atrioventricular | Right atrium and right ventricle |
Pulmonic | Semilunar | Right ventricle and pulmonary artery |
Mitral | Atrioventricular | Left atrium and left ventricle |
Aortic | Semilunar | Left ventricle and aorta |
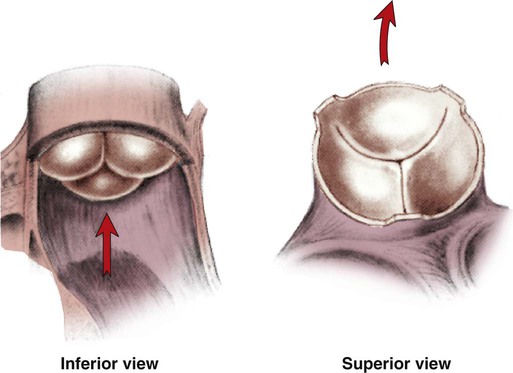
Conduction System
To analyze electrical activity within the heart, it is helpful to understand the three main areas of impulse propagation and conduction: 1) the sinoatrial (SA) node, 2) the AV node, and 3) the conduction fibers within the ventricle, specifically the bundle of His, the bundle branches, and the Purkinje fibers.15
Sinoatrial Node.
The SA node is considered the natural pacemaker of the heart because it has the highest degree of automaticity, producing the fastest intrinsic heart rate (Table 12-2). The node is a spindle-shaped structure located near the entrance of the superior vena cava, on the posterior aspect of the right atrium. Some normal variability in the position and shape of the node exists. The SA node is supplied from the right coronary system in 63% of people and from the left coronary system in 37%.16 The SA node contains two types of cells, the specialized pacemaker cells found in the node center and the border zone cells. Both cell types have inherent pacemaker properties (they automatically depolarize 60 to 100 times per minute). The cells in the nodal center are responsible for the pace making of the heart, whereas the intrinsic depolarization capability of the fibers in the border zone is depressed by surrounding atrial tissue.
TABLE 12-2
INTRINSIC PACEMAKER RATES OF CARDIAC CONDUCTION TISSUE
LOCATION | RATE (BEATS/MIN) |
Sinoatrial (SA) node | 60-100 |
Atrioventricular (AV) node | 40-60 |
Purkinje fibers | 15-40 |
Once the center nodal cells depolarize, the impulse is conducted through the nodal border zone toward the atrium. Atrial depolarization occurs cell to cell. Some anatomists postulate that specialized conduction pathways exit the SA node (Fig. 12-7A). These internodal pathways are directed to the AV node.17 An intra-atrial conduction pathway, known as Bachmann’s bundle, travels from the right atrium to the left atrium.18,19
Atrioventricular Node.
The AV node is located posteriorly on the right side of the interatrial septum, on the floor of the right atrium.20 The AV node receives its blood supply from the first posterior septal branch of the right (90%) or left (10%) coronary artery.16 Because the atria and ventricles are separated by nonconductive tissue, electrical impulses initiated in the atria are conducted to the ventricles only via the AV node. The AV node performs four essential functions to support cardiac conduction:
1. The AV node delays the conduction impulse from the atria (0.8 to 1.2 seconds) to provide time for the ventricles to fill during diastole.
2. The AV node controls the number of impulses that are transmitted from the atria to the ventricles. This prevents rapid irregular atrial heart rhythms from destabilizing the ventricular rhythm.
3. The AV node acts as a backup pacemaker if the faster SA node fails. Normally, the intrinsic AV nodal rate is slower than the SA nodal rate (see Table 12-2). When an impulse from the SA node arrives at the AV node, AV nodal tissue becomes depolarized, and the AV nodal pacemaker timing is reset (see Fig. 12-7B). This prevents the AV node from initiating its own pacemaker impulse that would compete with the SA node.
4. The AV node can conduct retrograde (backward) impulses through the node. If the SA and AV pacemaker cells fail to fire, an electrical impulse may be initiated in the ventricles and conducted backward via the AV node. Retrograde conduction time is usually longer than antegrade (forward) conduction.
Bundle of His, Bundle Branches, and Purkinje Fibers.
Electrical impulses are conducted in the ventricles through the bundle of His, the bundle branches, and the Purkinje fibers (see Fig. 12-7C). These structures run through the subendocardium, down the right side of the interventricular septum. About 12 mm from the AV node, the bundle of His divides into the right and left bundle branches. The right bundle branch continues down the right side of the interventricular septum toward the right apex. The left bundle branch is thicker than the right and takes off from the bundle of His at an almost right angle. It traverses the septum to the subendocardial surface of the left interventricular wall, where it divides into a thin anterior branch and a thick posterior branch. Functionally, when one of the left branches is blocked, it is referred to as a hemiblock. All of the bundle branches are subject to conduction defects (bundle branch blocks) that give rise to characteristic changes in the 12-lead electrocardiogram (ECG).
The right bundle branch and the two divisions of the left bundle branch eventually divide into the Purkinje fibers, which have the fastest conduction velocity of all heart tissue. Purkinje fibers divide many times, terminating in the subendocardial surface of both ventricles. This is followed by ventricular muscle depolarization (see Fig. 12-7D).
Coronary Blood Supply
The coronary circulation consists of those vessels that supply the heart structures with oxygenated blood (coronary arteries) and then return the blood to the general circulation (coronary veins). The right and left coronary arteries arise at the base of the aorta, immediately above the aortic valve (Fig. 12-8).21 They then traverse the outside of the heart, above the epicardium, in the natural grooves (sulci) between the chambers. To perfuse the thick heart muscle, branches from these main arteries arise at acute angles, penetrating the muscular wall and eventually feeding the endocardium (Fig. 12-9).21
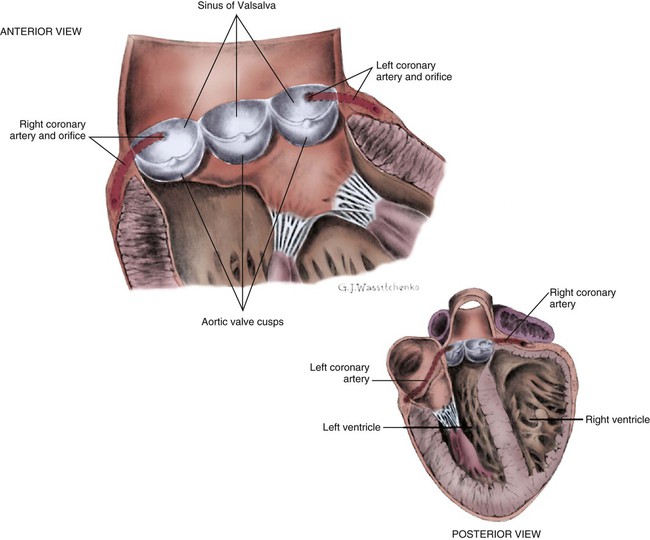
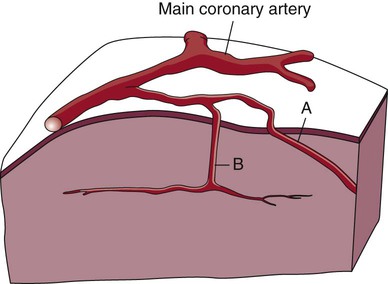
A, Epicardial arteries arise at acute angles from main coronary vessels to supply the epicardial surface of the heart. B, Smaller vessels branch at oblique angles from main coronary vessels that penetrate deeper into the myocardium and endocardium (intramural arteries). (Redrawn from Quaal S. Comprehensive Intraaortic Balloon Pumping. 2nd ed. St. Louis, MO: Mosby; 1993.)
The right coronary artery (RCA) serves the right atrium and the right ventricle in most people. In 63% of the population, the sinus node artery, which supplies the SA node, arises from the RCA.16 The AV node is supplied via the RCA in 90% of the population.16 The term dominant coronary artery is used to describe the artery that supplies the posterior part of the heart. In most people, the RCA is dominant, supplying the posterior cardiac wall.21
The left coronary artery is a short but important artery that divides into two large arteries—the left anterior descending (LAD) and the circumflex (Cx) arteries. These vessels serve the left atrium and most of the left ventricle (Fig. 12-10). The LAD artery travels in the sulcus over the anterior intraventricular septum. Septal perforator branches branch off at right angles to provide blood supply to the intraventricular septum.21
Coronary Veins.
The cardiac (coronary) veins, which carry deoxygenated blood, are adjacent to the paths of the coronary arteries, with one significant difference. The coronary veins ultimately join together to become the coronary sinus (the largest cardiac vein), which primarily empties into the back of the right atrium. The coronary venous blood then mixes with the systemic venous blood in the right atrium.22
Physiologic Cardiac Shunts.
An example of an abnormal, or pathologic, intracardiac shunt is an opening in the ventricular septum, between the left and right sides of the heart. In the ventricle, this septal opening, called a ventricular septal defect (VSD), allows mixing of blood from both ventricles. The clinical impact depends on the size of the intracardiac shunt. A VSD is a congenital opening between the ventricles; a ventricular septal rupture (VSR) can occur as a complication of a large anterior-wall MI, as shown in Figure 15-15.
Major Cardiac Vessels
Aorta
The aorta is the largest artery in the body. It carries oxygenated blood from the left ventricle to the rest of the body. The aorta is separated from the left ventricle by the aortic valve. Just above the aortic valve are two small openings that represent the origins of the right and left coronary arterial systems (see Fig. 12-8). These opening are known by several names, including the coronary ostia and the sinus of Valsalva.
Pulmonary Veins
The four pulmonary veins return oxygenated blood from the lungs to the left atrium. These are the only veins in the body that carry oxygenated blood. The veins drain into the back wall of the left atrium (see Fig. 12-10). No valves inhibit the flow of blood into the left atrium. Blood flow is accomplished by simple hydrostatic pressure gradients. The pressure must be lower in the left atrium than in the pulmonary circulation for flow to occur in a forward direction.
Systemic Circulation
Arterial System.
Arteries are constructed of three layers (Fig. 12-11): 1) The adventitia, the outermost layer, is composed largely of a connective tissue coat to provide strength and shape to the vessel; 2) the media, or muscular middle layer, is made up of smooth muscle and elastic tissue. The muscular layer changes the lumen diameter when necessary; and 3) the innermost layer, or intima, consists of a thin lining of endothelium and a small amount of elastic tissue. The smooth endothelial lining decreases resistance to blood flow and minimizes opportunity for platelet aggregation.
The intimal and the adventitial layers remain relatively constant in the vascular system, whereas the elastin and smooth muscle in the media vary in proportion, depending on the size and type of the vessel. The aorta contains the greatest amount of elastic tissue. This is necessary because of the sudden shifts in pressure created by the left ventricle. The arterioles, or smaller arteries, and precapillary sphincters have more smooth muscle than do the larger arteries and aorta because they function to change the luminal diameter when regulating blood pressure and blood flow to tissues (Fig. 12-12).
Blood Flow and Blood Pressure.
The pulsatile nature of arterial flow is caused by intermittent cardiac ejection and the stretch of the ascending aorta. The pressure wave initiated by left ventricular ejection (Fig. 12-13) travels considerably faster than does blood itself. When an examiner palpates a pulse, it is the propagation of the pressure wave that is perceived.