Chapter 11 Breathing filters, humidifiers and nebulizers
Gasses supplied from cylinders or pipelines need to be very dry to reduce the risk of corrosion, condensation and frost forming in cylinders, pipes and valves. Gasses delivered to the patient’s trachea, therefore, need to be artificially warmed, humidified and filtered to prevent damage to the patient’s airways,1,2 to maintain the effectiveness of the mucociliary elevator3 and to reduce the incidence of infection.4 This chapter deals with devices that fulfill these functions.
Breathing system filters
Filtration and mechanisms of filtration
Filtration is the removal of particles from either a gas or a liquid suspension. Filters are used to remove particles from gasses delivered to patients, to prevent microbes from patients cross-infecting other patients and staff, and to reduce the contamination of equipment. In addition, sputum expectorated by a patient and condensation in breathing systems may harbour pathogens, and filters can be used to reduce the risk of liquid-borne cross-infection.5
Mechanisms of filtration of gas-borne particles
Filter material generally consists of fibres formed into a non-woven wad or sheet. There are five main mechanisms by which the filter material removes particles from a flow of gas (Fig. 11.1):
b Inertial impaction
Particles have mass and are, therefore, not always able to follow a gas streamline around a fibre due to their inertia. The particles may, therefore, strike the fibre, even though the gas streamline is more than one particle radius from the fibre.
Most penetrating particle size
The relative efficiencies of the five mechanisms of filtration vary with the size of the particle (Fig. 11.2). In particular, particles of a certain size, typically in the range 0.05–0.5 µm, pass through the filter more easily than others. This size is known as the most penetrating particle size. Particles of this size are too small to be directly intercepted by fibres and too large to undergo substantial Brownian motion.
Types of filter
There are two main types of filter material used in breathing system filters:
Glass fibre filters
This filter material consists of a sheet of resin-bonded glass fibres. The fibres are packed densely (Fig. 11.3A) and hence the sheet has a high resistance to gas flow per unit area. A sheet with a large surface area is used to reduce the resistance to gas flow to an acceptable level. The sheet is then pleated to minimize the required volume, and hence dead space, for the housing. This type of filter material is hydrophobic and under normal clinical conditions, does not absorb water.
Electrostatic filters
1 Tribocharged filters
An electrostatic charge can be induced on two dissimilar fibres by rubbing them together during the manufacturing process, so that one type becomes positively charged and the other type negatively charged (tribocharging). One such filter material is made from fibres of polypropylene and modacrylic which can then be converted into a non-woven felt (Fig. 11.3B).
2 Fibrillated coronal-charged filters
An electrostatic charge can be applied to a sheet of polypropylene by using a point electrode emitting ions (corona charging). An opposite charge can be induced on the rear of the sheet. This type of material is often called an electret. If the sheet of polypropylene is now stretched, the strength of the molecular bonds is enhanced in the direction of the stretching, but reduced in a direction perpendicular to it. The sheet can then be split into fibres – a process called fibrillation – and made into a non-woven filter wad (Fig. 11.3C).
Measuring the performance of breathing system filters
The filtration efficiency of a filter is determined by measuring the number of particles passing through the filter as a percentage of the number of particles in an aerosol challenge to the filter. This percentage is the penetration value for the filter. Although challenges of microbes can be used,6 the standard for breathing system filters specifies that the challenge should consist of a particular quantity of an aerosol containing sodium chloride particles having diameters close to the most penetrating particle size.7 The filter is challenged at a flow of 15 or 30 L min−1 for filters intended for use with paediatric or adult patients, respectively. Typical penetration values for filters are shown in Fig. 11.4.
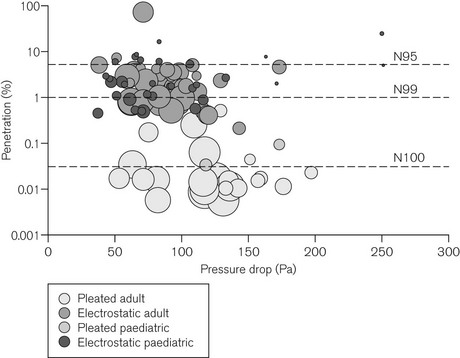
Figure 11.4 Penetration through 104 different filters when challenged with an aerosol of sodium chloride particles at the most penetrating particle size against pressure drop measured across the filter (100 Pa ≈ 1 cm H2O). Filters were tested at flows of 15 l min−1 (paediatric) or 30 l min−1 (adult), respectively. The size of each bubble is proportional to the internal volume of the filter. An ideal filter would have low values for penetration, pressure drop and internal volume (a small ‘bubble’ in the lower left hand side of the figure). However, filters with low penetration (high filtration efficiency) and low-pressure drop tend to be large filters; smaller filters tend to have higher pressure drops and higher penetration values (low filtration performance). N95, N99 and N100 refer to filtration efficiencies: namely <5%, <1% and <0.03% penetration respectively.
Adapted from Wilkes AR. Heat and moisture exchangers and breathing system filters: their use in anaesthesia and intensive care. Part 1 – history, principles and efficiency. Anaesthesia 2011;66:31-39 with permission from Wiley-Blackwell.
Humidifiers
Humidity
Humidity is used to describe the amount of water vapour in air or gas. The mass of water vapour in the gas is the absolute humidity (g m−3). The maximum amount of humidity that gas can contain is limited by temperature (Fig. 11.5). At the maximum humidity for a particular temperature, the gas is said to be saturated with water vapour, and the level of humidity is the humidity at saturation. The relative humidity (RH; %) is the absolute humidity of the gas at a particular temperature as a percentage of the humidity at saturation at the same temperature.
Room air at 22°C typically has an absolute humidity level of approximately 10 g m−3. The humidity at saturation of air at 22°C is approximately 20 g m−3, so that the room air has a relative humidity of approximately 50% RH. If the air is cooled, a point is reached at about 11°C when the absolute humidity level equals the humidity at saturation, and hence the relative humidity is 100%. If the air cools to an even lower temperature, condensation will occur. If room air is inspired, the air is warmed to 37°C by the upper air passages by the time it reaches the lungs, and the humidity is increased from 10 to 44 g m−3 (BTPS conditions: body temperature and pressure, saturated). The difference between the two (−34 g m−3) is the humidity deficit: humidity must be added by the airways to reduce this deficit to 0 g m−3. If the room air is warmed from 22 to 37°C without any humidification, the relative humidity will fall to 100 × (10 ÷ 44) = 23%. The massic enthalpy (latent heat) of evaporation of water is 2.4 kJ g−1. To saturate inspired gasses, which have a low level of humidity, a considerable proportion of the body’s heat production must be used (up to one-third for a neonate). This can then lead to a fall in the patient’s core temperature of more than 1°C.