30 Blood and Fluid Management during Cardiac Surgery
Transfusion guidelines

Rationale for Guidelines
Clinical guidelines are a ubiquitous part of medicine that are “systematically developed statements to assist practitioner and patient decisions about appropriate healthcare for specific clinical circumstances.”1 The U.S. National Guideline Clearinghouse (http://www.guideline.gov) includes more than 2480 recently generated guidelines. Guidelines are valuable methods for reducing practice variation, errors, and to ensure efficient use of healthcare resources.2 Despite the availability of well-constructed guidelines, clinicians often are reluctant to implement guidelines in daily practice. Several studies have shown that guidelines may do little to change practice behavior.3
Formulation of Guidelines
Groups developing guidelines require support from a national or international society that can endorse and disseminate guidelines. Members of the guideline committee should be recognized experts in the field and be assisted by people with expertise in guideline preparation. American Heart Association guidelines are the model for well-crafted guidelines, developed using well-conducted peer-reviewed studies as their evidence base (Table 30-1).
TABLE 30-1 Requirements for Successful Guideline Development and Implementation
Guideline Writing Group Formation |
Support at a societal level |
Perception of need to define practice standards |
Senior leadership for guideline preparation |
Statistical support for examination of evidence |
Guideline Preparation |
Sufficient evidence base for guideline preparation |
Sufficient expertise for guideline preparation |
Dedicated time and effort for guideline generation |
Dissemination to content and structure experts for commentary |
Guideline Dissemination |
Wide dissemination using an authoritative journal within the field |
Wide dissemination using traditional and nontraditional mechanisms such as Web sites, conferences, and special interest groups |
Guideline Conformity at the local institutional level |
Senior leadership within the institution |
Incorporation of guidelines into the local context of clinical practice |
Providing practitioners with the resources to conform to the guidelines |
Providing practitioners with timely, accurate, and pertinent feedback on measured conformity with guidelines |
Guidelines can be based on a spectrum of evidence from case reports, series without control groups, or randomized, controlled trials. The Society of Thoracic Surgeons/Society of Cardiovascular Anesthesiologists Transfusion Guidelines published in 20074 are a good example of generally well-crafted guidelines, but based on limited available evidence. There were 57 recommendations in the guidelines. For level of evidence, only 13 were level A (best level of evidence), 27 were level B (limited evidence), and 17 were level C (very limited evidence). For class of recommendation, only 7 were class I (benefit strongly outweighs risk), 18 were Class IIa (benefit outweighs risk), 23 were Class IIb (benefit may outweigh risk), and 10 were class III (risk outweighs benefit).5 The relatively low level of evidence for the guidelines likely is reflected by a lack of wide implementation by clinicians.

Implementation of Guidelines
To be effective, guidelines should be widely disseminated using commercial marketing tools. Anesthesia and surgical societies have been comparatively slow in adopting guidelines. Although the recently announced Society of Thoracic Surgeons/Society of Cardiovascular Anesthesiologists Transfusion Guidelines were widely distributed throughout both societies and to the perfusion community, many practitioners were not aware of the content of the guidelines. Several other studies have found similar lack of awareness of other guidelines.6,7
A number of other factors may hinder effective implementation of guidelines by practitioners such as guidelines that are too complicated and difficult to implement or lack rigorous scientific evidence as a basis. In other fields, such as general medicine practices, several studies have demonstrated low rates of change in response to guidelines.8–10 Furthermore, in the surgical environment that relies on multispecialty teams, there is a need for the entire team to understand the rationale for practice guideline implementation. However, it is equally important to realize that “guidelines need to remain flexible enough to permit a degree of patient-specific departures from specified prevention, diagnostic, and treatment protocols.”11
Other key components for successful implementation of guidelines lie in senior institution leadership and endorsement,12 as well as practice-specific feedback of performance. Implementation of guidelines in cardiac surgery previously has been reported to be poor,13,14 but focused implementation of guidelines at single institutions has been reported to be successful.15,16 Quality improvement initiatives used in industry such as Total Quality Management and Six Sigma, which appear applicable to surgical processes, are dependent on collection of verifiable data concerning processes.17,18 The notion that clinicians can improve cardiac surgical care and the performance of individual members of the team without proper timely feedback is not reasonable. In fact, real-time feedback has been demonstrated to be particularly effective.19 In summary, individuals involved in the management of cardiac surgical patients are obligated to provide the best possible clinical care, often guided by guidelines. Societies have a key role in endorsement, dissemination, and use of guidelines to improve care.
Blood groups and transfusion

ABO Blood Groups
ABO and Rh blood groups are the most well-known of more than 30 antigen-based classifications of human blood types. The ABO blood group system is based on identification of A and B antigens present on RBCs and was originally described by Janský and Landsteiner.20 Wide variation exists in the frequency of the ABO groups across different populations, with group B being the most common in Asians but comparatively rare in whites.21
ABO blood grouping is defined by presence or absence of surface antigens on the RBC membrane. The ABO gene is located on chromosome 9q34 and has three principal isoforms (A, B, and O) that are determined by single nucleotide polymorphisms (SNPs) and single-base deletions within the ABO gene (Table 30-2). Four missense SNPs determine the structural and functional differences between the A and B transferases. The different transferases result from four amino acid substitutions at amino acid positions (codons) 176, 235, 266, and 268 and nearly always occur together as a group. Two of these SNPs (L266M and G268A) change the substrate specificity of the ABO enzyme for galactose. The protein produced by the ABO gene is not the antigen. Rather, O, A, and B antigens are formed by the action of three different glycosyltransferases (isoforms) encoded by the ABO gene that modifies a cell membrane glycoprotein (H antigen).22
The O blood group is caused by a single base deletion (261delG) in exon 6 of the ABO gene that results in a frameshift mutation and translation of a truncated ABO protein, the O isoform, with no enzymatic activity; therefore, the A and B antigens are not present (the H antigen is unmodified).23
In the A transferase, the amino acids are leucine (L) and glycine (G) at codons 266 and 268, respectively. The A isoform (L266 and G268) encodes a glycosyltransferase (A transferase) that bonds α-N-acetylgalactosamine to the H antigen, producing the A antigen of the ABO blood group system. Individuals who exclusively synthesize A isoforms are blood group A and have the genotype AA (homozygotes: same A allele on both chromosomes) or AO (heterozygotes: A allele only present on one chromosome).23
In the B transferase, the amino acids are methionine (M) and alanine (A) at the same codons 266 and 268, respectively. The B isoform (M266 and A268) encodes a glycosyltransferase (B transferase) that joins α-d-galactose to the H antigen, creating the B antigen. Blood group B individuals have the genotype BB (homozygotes) or BO (heterozygotes). Individuals who express both A and B isoforms of the ABO gene (an A allele on one chromosome 9 and a B allele on the other chromosome 9) are blood group AB.23
Other variations in the ABO gene that create functionally similar antigens also exist. For example, the A(2) isoform, comprising only 20% of group A individuals, is caused by deletion of a protein coding termination point, thus extending the enzyme by 21 extra amino acids and altering its specificity. These structural differences reflect the different catalytic activities of the enzymes encoded by the A(1) and A(2) alleles and result in different antigenic properties of A(1) and A(2) antigens.24 Different molecular mechanisms may be responsible for seemingly identical ABO blood groups. For example, the B3 phenotype may be caused by either a missense mutation (D291N), a splicing mutation (B303), or the combination of a missense mutation and a single nucleotide deletion (V277M and 1060delC).
ABO blood groups also could be measured by genotyping the variants in the ABO gene instead of measuring the presence or absence of the A and B antigens. The ABO antigens are also expressed on the surface of many other cells types, indicating the importance of ABO cross-matching of organ transplants. Several other rare variants of the ABO gene that change activity and/or specificity of the enzyme have been identified and generate several rare blood groups.25
Anti-A and anti-B antibodies (isohemagglutinins) are IgM antibodies that appear in the first years of life. Early in the postnatal period, the immune system generates IgM antibodies against ABO antigen(s) even when they are absent from the individual’s RBCs. The IgM antibodies, if present in the fetus, are too large to cross the placenta, and thus are not a relevant cause of hemolytic disease of the newborn. The antibodies are thought to be produced in response to infantile exposure to influenza virus and gram-negative bacteria. Antibodies usually are not generated against the H antigen. Therefore, an individual with blood group A will make IgM antibodies against the B antigen. An individual with blood group B will make IgM antibodies against the A antigen. An individual with blood group AB will not make IgM antibodies to the A and B antigens. Finally, an individual with blood group O will make IgM antibodies against both the A and B antigens.23
If an individual of blood group A receives group B RBCs, anti-B IgM isohemagglutinins in the recipient plasma bind to the B antigen on donor RBCs, generating a locus for complement-mediated lysis of transfused donor RBCs and generation of a hemolytic transfusion reaction. Similar events occur in group O and B individuals receiving RBCs containing non–self-antigens. However, blood group AB individuals do not generate anti-A and anti-B antibodies. Therefore, they can receive RBCs from all groups and are universal RBC recipients.23

Rhesus Blood Groups
The rhesus (Rh) blood group system consists of ~50 blood group antigens among which five antigens—D, C, c, E, and e—are the most important. The proteins that carry the Rh antigens form a transmembrane transporter complex that resembles NH3 and CO2 transporters of evolutionary origin.26 The proteins are encoded by two adjacent genes on chromosome 1p36.13-p34.3: the RHD gene that encodes the RhD protein with the frequent D antigen and the RHCE gene that encodes the RhCE protein with the C, E, c, and e antigens.27 The term Rh factor refers only to the D antigen that is normally present. Unlike the ABO system, the absence of the normally present D antigen is called the d antigen, but there is no protein corresponding to the d antigen. Lowercase “d” indicates the absence of the D antigen, often as a result of the deletion of the gene or other variants that prevent expression of the antigenic protein on RBCs.27 To be Rh negative, the individual must have the gene absent on both chromosomes.
The frequency of Rh-negative individuals ranges from ~16% in white populations to less than 1% in Asian populations.28 Rh incompatibility of an RhD-negative mother and RhD-positive fetus is the predominant cause of hemolytic disease of the newborn, which occurs when maternal IgG anti-RhD antibodies pass through the placenta into the fetal circulation and cause hemolysis of RhD antigen-positive fetal RBCs.29

Other Blood Groups
At least 30 other antigens are expressed on the RBC surface including the Kell–Cellano, MNS, and Lewis blood groups. These blood groups are capable of causing transfusion reactions, though most are less common and produce less severe transfusion reactions than ABO incompatibility. Several are capable of producing hemolytic disease of the newborn.29
The Kell–Cellano antigens are variants of a transmembrane glycoprotein (ET3) encoded on chromosome 7q33. In contrast with many other blood groups, the function of the enzyme is known and is responsible for producing endothelin-3, a potent bioactive peptide with multiple biologic roles. There are several variants of the gene, of which K1 (Kell) and K2 (Cellano) are the most common and result from an SNP generating a Thr193(K2) from the more frequent Met193(K1) isoform. Kell incompatibility is second to Rh incompatibility for generation of hemolytic disease of the newborn.29
The MNS antigens are variants of two genes, glycophorin A (containing the M and N alleles) and glycophorin B (containing the S and s alleles), adjacent to each other on chromosome 4q28-q31. The glycophorins are the most common sialoglycoproteins present on the RBC membrane, but their function is not clear. Unlike most other blood group systems in which individual SNPs or deletions create the antigens, the MNS antigens are created by complex rearrangements of the protein structure that generate antigens.30
The Lewis blood group antigens are structurally similar to the ABO and the H blood group systems. The antigens are generated by variants in the fucosyl transferase gene (FUT3), residing on chromosome 19p13.3, that acts on the Lewis antigens in a similar fashion to the galactose transferase function of the ABO gene acting on the H antigen. The Lewis antigens are not synthesized in erythrocyte progenitor cells like the ABO antigens, more likely in the gut. The Le-a or Le-b antigens circulate in plasma bound to serum lipoproteins and are adsorbed to circulating erythrocytes, usually only after birth.31

Cross-matching
The use of citrate, refrigeration, and a nascent understanding of blood incompatibility during the First World War enabled the development of blood banking.32 The first U.S. hospital blood bank was created at Cook County Hospital in 1937.32 About 16 million units of blood was transfused in the United States in 2008.33 Cross-matching of donor blood products to an individual recipient is a sequence of procedures performed to prevent transfusion reactions. American Association of Blood Banks’ Standards for Blood Banks and Transfusion Services has defined the procedures performed before blood is transfused to a recipient. The first laboratory component for the recipient blood specimen is a “type and screen,” which consists of two separate tests.34 First, the recipient’s ABO and RhD blood groups are determined (typed) by using commercially produced anti-A and anti-B antibodies that will react with the A or B antigens, if present, on the recipient’s RBCs, causing the RBCs to agglutinate. The RhD antigen is tested in the same manner, with commercially available anti-D antibodies mixed with the recipient’s RBCs.34
Second, antibody screening is to identify whether the recipient has formed antibodies to nonself blood groups, such as Duffy, MNS, Kell, Kidd, and P system antigens, using an updated version of the classic Coombs test, also called the indirect antiglobulin test (Figure 30-1). The recipient’s serum or plasma is mixed separately with three or more commercially available type O washed RBCs that are known to express ~20 of the most “clinically significant” RBC antigens, to detect unexpected RBC antibodies. If transfusion is required, the recipient sample that is already typed and screened is cross-matched with donor units. Provided recipient antibodies were not identified on the type and screen, it is possible to perform a cross-match either serologically, using an immediate spin cross-match, or by an electronic match. If clinically significant antibodies have been found on the type and screen, electronic cross-matching is not sufficient and antiglobulin cross-match must be performed.34 An electronic or computerized cross-match is performed by identifying donor units on hand that have appropriate ABO and RhD blood groups for the recipient.35 Electronic cross-matching only can be used if a patient has a negative antibody screen, which means that they do not have any active RBC atypical antibodies. It is assumed that the proper testing (type and screen) on the recipient and donor blood are sufficient to identify clinically important incompatibility and to identify matching donor blood.36
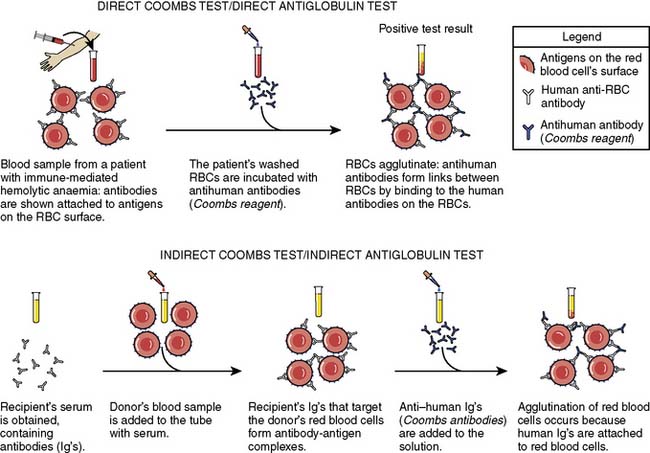
Figure 30-1 The indirect and direct Coombs (antiglobulin) tests.
(Modified from Wikipedia: Coombs test schematic. Available at: http://en.wikipedia.org/wiki/File:Coombs_test_schematic.png.)
When serologic cross-matching (immediate-spin cross-match) is performed, RBCs from an ABO and RhD-compatible RBC unit are mixed with the recipient’s plasma. The mixture is centrifuged and examined for hemolysis or agglutination. Agglutination is considered a positive reaction, indicating that the donor unit is incompatible for that specific patient. If both are absent, ABO compatibility is verified and the RBC unit issued. This procedure is repeated for each donor RBC unit. If agglutination or hemolysis occurs, additional screening of the recipient’s plasma is performed to identify the unexpected antibodies.36 Performing a serologic cross-match, over electronic cross-matching, before transfusing RBCs is preferred by some laboratories because it detects rare ABO errors and also detects most recipient IgM antibodies to antigens on donor RBCs.37 In an emergency, “uncross-matched blood” can be transfused and risk for a serious transfusion reaction minimized by administration of type O and RhD-negative RBCs.38
Components
Because plasma contains the anti-ABO, RhD, and other antibodies of the donor, only ABO and RhD-compatible units are transfused. Thus, the recipient and donor unit must undergo a type and screen; however, cross-matching is not performed. Platelets have ABO antigens on their surface. However, ABO compatibility for platelet transfusion is desirable but not required because of the relatively small volume of plasma present in a bag of platelets. If ABO-incompatible platelets are administered for operational reasons, the recipient subsequently may have a positive direct antiglobulin test result, but significant hemolysis is rare. Furthermore, a donor–recipient ABO mismatch may result in poor function of donor platelets after transfusion.39–41

Immediate Immune-Mediated Complications of Transfusion
Hemolytic transfusion reaction is the result of complement-mediated destruction of transfused RBCs, nearly always because of incompatibility of antigen on the transfused RBCs with antibody in the recipient’s circulation. The most common cause of acute hemolytic reactions is transfusion of ABO-incompatible blood; rarely, undetected serologic incompatibility is a cause of acute hemolysis.42
Transfusion has been associated with greater pulmonary morbidity after cardiac surgery.43 Transfusion-related acute lung injury (TRALI) occurs when increased permeability of the pulmonary endothelium causes pulmonary edema, usually within a few hours of transfusion. Hypotension and fever also may occur.44,45 TRALI constitutes the majority of transfusion-associated mortality in the United States.45,46 The specific cause of TRALI is unknown; however, in many cases, the occurrence of TRALI is associated with the presence of antibodies in donor plasma directed toward the recipient’s leukocyte or neutrophil antigens.45,46 These antibodies are seen more frequently in multiparous women and individuals with prior transfusion.47 Transfusion of blood components, especially products containing high volumes of plasma such as fresh-frozen plasma (FFP), have the greatest risk for TRALI.47 Thus, plasma from women is usually, but not exclusively, used for processed protein fractions rather than transfusion. No routinely available pretransfusion testing is available.
Immune-mediated platelet destruction is the result of recipient antibodies being present to human leukocyte or platelet-specific antigens present on transfused platelets. Most cases of immune-mediated platelet destruction occur in individuals who have had several prior platelet transfusions. In some patients, platelet matching may be required.41
Anaphylactoid reactions rarely occur in transfusion of IgA-containing plasma present in any blood product given to IgA-deficient patients who have anti-IgA antibodies. The reaction is characterized by hypotension, bronchospasm, and laryngeal edema.48
Febrile nonhemolytic reactions are relatively frequent and typically manifested by a temperature increase of 1° C or more occurring during or shortly after transfusion. They may result from antibodies to white blood cells or from the presence of high levels of cytokines in transfused blood products.49

Delayed Immune-Mediated Complications of Transfusion
Delayed hemolytic reactions occur in patients who have had previous exposure to incompatible blood but who do not have circulating antibodies. Re-exposure to the antigen provokes delayed production of antibody that reaches a significant circulating level while the transfused RBCs are still present in the circulation, usually 2 to 14 days after transfusion.50
Graft-versus-host disease (GVHD) is a rare condition that occurs when viable donor T lymphocytes in the transfused blood product successfully engraft in the recipient. Normally, donor T cells are recognized as foreign by the recipient’s immune system. However, in immunocompromised patients, and rarely when the donor is homozygous and the recipient is heterozygous for an HLA haplotype (as can occur in directed donations from first-degree relatives), the recipient’s immune system is not able to destroy the donor T cells. This can result in graft-versus-host disease. Irradiation of the donor unit prevents T-cell proliferation and graft-versus-host disease.51,52
Nonimmune Complications of Transfusion
Transmission of infectious disease may occur with transfusion despite standard blood-banking operational procedures. Routine testing of donor blood is performed for Chagas disease, hepatitis B and C, human immunodeficiency viruses types 1 and 2, human T-lymphotropic virus, syphilis, West Nile virus, and, in some situations, cytomegalovirus.33 Bacterial contamination occurs rarely and is manifested by fever and hemodynamic instability surrounding transfusion of the blood product.53 Circulatory overload, hypothermia, and metabolic and electrolyte derangements are other complications that may occur in high-volume transfusion.54
Transfusion and Morbidity Outcomes
Although transfusion of RBCs is necessary for some patients, its use has been associated with a dose-dependent greater prevalence of morbidity after cardiac surgery.55 Greater rates of postoperative infectious complications, prolonged postoperative ventilatory support, renal injury, and reductions in short- and long-term survival are more common in patients transfused with RBCs.43,55–57 Greater rates of bacteremia, septicemia, and deep and superficial sternal wound infections are thought to be secondary to a downregulation of the immune system.56,58 Transfusion has been related to an increased development of postoperative atrial fibrillation thought to be secondary to the influence of RBC transfusion on inflammation. RBC transfusion results in a direct infusion of inflammatory mediators, as well as augmenting the inflammatory response to cardiopulmonary bypass (CPB) and cardiac surgery.59,60
Storage duration of the RBC product also may be a contributing factor for observed adverse outcomes. The storage lesion consists of a series of structural and functional changes occurring with increasing RBC storage. Some of these changes are reversible, others are not, and together may result in decreased microvascular tissue flow.61–63 A recent investigation reported transfusion of RBCs stored longer than 14 days was associated with a greater risk for death and complications after cardiac surgery.64 A recent laboratory investigation from Sweeney et al65 reported increased thrombin generation for RBC units of increasing storage duration, suggesting a cause for increased complications observed with transfusion of RBCs of increased storage duration.
Genetic causes of hemorrhage
In general, the coagulation system can be thought of as being highly optimized toward rapid cessation of hemorrhage. There likely has been a strong evolutionary pressure toward rapid coagulation and wound healing. Of course, there must be an equally highly developed system that prevents overwhelming coagulation of the entire blood volume in response to a trivial intravascular insult. In contrast, there was likely little or no pressure to avoid a deep venous thrombosis in older age because most individuals did not live beyond 40 years until a few centuries ago. Similarly, there has been no evolutionary pressure to successfully undergo blood exposure to foreign surfaces such as the CPB circuit. Because severe bleeding abnormalities are strongly selected against, in evolutionary terms, they are rare. A sentinel example is the hemophilias, in which a rare variant causes production of a nonfunctional protein with severe consequences. In contrast, trivial abnormalities have not undergone marked evolutionary pressures and are likely to be more common. A good example of this is the wide, but seemingly unimportant, heritable variation seen in the circulating level of many coagulation proteins.66–69
Genetic causes of impaired coagulation can be somewhat simplistically thought of as either a qualitative defect arising from abnormal protein structure because of a coding genetic variant or a quantitative defect arising from abnormal, usually reduced, production of a normal protein because of a noncoding (promoter) genetic variant. This approach is rudimentary but gives the best basic understanding of the genetic mechanisms of coagulation disorders. Like all genetic disorders, the overall effect of a genetic variant on the whole human or surgical population is a product of the frequency of the genetic variant and the biologic effect of the variant.70 If the variant is rare, such as the hemophilias, although the disease is problematic for a single individual, the overall effect on day-to-day practice is low because of its rarity. By contrast, a more common variant may have greater effect. Similarly, for two variants of equal frequency, the one with low biologic effect will have less overall influence than one with a higher biologic effect. To date, no frequent variants with high biologic effect on coagulation have been identified.69

Variation in Coagulation Protein Levels
Several studies have demonstrated the strong heritability of levels of plasma proteins and platelet levels in normal populations.68,71–74 This type of research is undertaken by examining the plasma level of coagulation proteins in multigenerational families and estimating the within-family variation compared with the whole-population variation.75 In accordance with the believed strong evolutionary importance of coagulation function, there is similar strong inheritance within families, with up to 70% of the variation in plasma protein levels determined by genetic heritability. Similar heritability of platelet count and platelet volume has been observed.76,77
Genetic variation associated with the circulating level of a coagulation protein is usually in or around the gene for that coagulation protein. For example, the circulating level of plasminogen activator inhibitor 1 (PAI-1) principally is determined by a common promoter variant that alters the binding of the transcription factor inhibitor.66,67 Similarly, the circulating level of thrombin-activated fibrinolysis inhibitor is determined by two variants,78 and circulating prothrombin levels are determined by a single variant.79 However, some coagulation protein levels are regulated by genetic variation that is not in or around the protein’s gene. Similarly, platelet count and platelet volume are regulated by genes that would not be intuitive choices.76,77

Hemophilias
Hemophilias are the most well-known example of a rare severe genetic disorder of coagulation. Hemophilia A (factor VIII deficiency) is the most common hemophilia, occurring in about 1 in 5000 male births.80 Hemophilia B (factor IX deficiency) occurs in about 1 in 34,000 male births. Both genes lie on the X chromosome, meaning that in male individuals, only one copy of the chromosome is present and a single variant may result in hemophilia (often called a sex-linked or X-linked disease). Accordingly, female hemophiliacs must have two copies of the rare variant—an event that usually occurs only in consanguineous births in which the father has hemophilia. Although most hemophilias are maternally inherited, about 30% are spontaneous mutations not present in the mother. An obvious question is why female individuals do not have twice the factor VIII and IX levels as male individuals. The reason is that in female individuals, one of the X chromosomes is usually “turned off” and does not generate messenger RNA for translation of proteins. However, it is possible for female carriers to have mild hemophilia because of lyonization (inactivation) of the X chromosome that carries the normal gene, leaving the abnormal gene to be the most active. Adult women may experience menorrhagia because of the bleeding tendency. Hemophilia C is an autosomal genetic disorder (not X-linked) involving a lack of functional clotting factor XI. Hemophilia C is not completely recessive because heterozygous individuals also show increased bleeding.81
The gene F8 encodes factor VIII of the intrinsic pathway. Factor VIII is a cofactor for factor IXa, which converts factor X to the activated form Xa. There are almost 700 known coding variations of the F8 gene, many of which have been seen in only one individual or family. More than 170 give severe forms of hemophilia A, and more than 180 produce milder forms.82,83 Some of these mutations change one amino acid; many of these have little effect on protein function because only one amino acid is changed, often to a similar type of amino acid.84 Others cause a frameshift mutation that usually truncates the protein and markedly reduces its function. Another severe mutation is an inversion of a portion of the genome and, therefore, the sequence of a portion of the protein is “back-to-front” with marked reduction in function. Rarely, the entire gene is deleted so that both quantitative and qualitative assays show the absence of factor VIII. The diagnosis of hemophilia A is made by reduced factor VIII activity, prolonged activated partial thromboplastin time except in mild disease, and a normal PT and platelet count.84
The gene F9 encodes the vitamin K–dependent factor IX of the intrinsic pathway. Factor IX is activated by factor XI to factor IXa. Because hemophilia B is rarer than hemophilia A, fewer mutations have been described, but the mechanisms of protein dysfunction from genetic variation are similar. The diagnosis of hemophilia B is made by reduced factor IX activity, prolonged activated partial thromboplastin time except in mild disease, and a normal PT and platelet count.84
Hemophilia is not a contraindication for cardiac surgery, but it is an absolute indication for involvement of a hematologist and blood banker. Most experience has been small case series or single case reports, but all emphasize the need for prolonged factor therapy and subsequent excellent outcomes. For hemophilia A, the factor VIII activity level should be corrected to 100% of normal for cardiac surgery, although some hematologists use 50% to 70% of normal as a goal. One unit of factor VIII is the normal amount of factor VIII in 1 mL plasma, by definition. Because the volume of distribution of factor VIII is that of plasma, the amount of factor VIII in an individual is ~50 mL/kg. The factor VIII dose needed to correct the level is calculated as follows85,86:
Approximately 30% of people with severe hemophilia A develop antibodies to transfused factor VIII. These antibodies (also called inhibitors) bind to transfused factor VIII and reduce its activity so increased doses of factor VIII are required.87 The next dose should be administered 6 to 12 hours after the initial dose, together with repeated factor VIII activity monitoring with the goal of keeping the trough activity greater than 50%. During hemorrhage, cryoprecipitate and FFP can be given to restore levels of factor VIII and other coagulation factors, as well as blood volume. 1-Deamino-8-d-arginine vasopressin (DDAVP) at a dose of 0.3 μg/kg can be used in mild or moderate hemophilia A, and works by increasing circulating factor VIII and von Willebrand factor (vWF) levels. DDAVP works only when some normal factor VIII activity is present and usually with only modest success.88
For hemophilia B, the factor IX activity level should be corrected to 100% of normal for cardiac surgery, although some hematologists use 50% to 70% of normal as a goal. One unit of factor IX is the normal amount of factor IX in 1 mL plasma, by definition. Unlike factor VIII, the volume of distribution of factor IX in an individual is 100 mL/kg. The factor IX dose needed to correct the level is calculated as follows89: