Chapter 26 Benign Brain Tumors
Meningiomas and Vestibular Schwannomas
Michael Chan and C. Leland Rogers
Harvey Cushing first used the term meningioma to describe tumors originating predominately from the meningeal coverings of the brain and spinal cord. Although the term does not disclose a cell of origin, it does aptly describe a range of clinically comparable histologic patterns and has endured. Though meningiomas are often approached as benign tumors, studies with long-term follow-up reveal that they are prone to infiltrate locally and to recur.1 This chapter will consider the available data on intracranial meningiomas in a succinct fashion. For a more detailed review and lengthier bibliography, readers can consult the online version of this chapter.
Etiology and Epidemiology
Based upon surgical series, approximately 8000 meningiomas are diagnosed each year in the United States. Radiographic and autopsy studies suggest an even greater number of patients with clinically occult tumors.2 A recent epidemiologic study revealed that meningiomas are the most frequently reported primary intracranial neoplasm, constituting approximately 30% of all brain primary tumors.3
The likelihood of developing a meningioma is proportional to age. Pediatric meningiomas are rare but are more likely to exhibit an aggressive clinical course.4 Meningiomas are most often diagnosed during the sixth to seventh decades of life. However, the age-specific incidence continues to rise thereafter, even beyond 85 years of age.3
Although there are known associations with certain genetic, environmental, and hormonal risk factors, most meningiomas arise without discernible causation. Genetic factors will be discussed in an ensuing section on Biologic Characteristics and Molecular Biology. Radiation exposure—stemming largely from studies of atomic bomb fallout but also from studies of cranial and scalp irradiation for tinea capitis—is a well-described environmental etiologic factor for meningiomas. Indeed, radiation-induced meningiomas are the most commonly reported secondary neoplasm.2
A role for sex hormones in meningioma induction is supported by several findings. Meningiomas occur more frequently in females, at a ratio of 2 or 3:1.2 The incidence appears to increase with hormone replacement therapy or long-acting oral contraceptive use and in obese individuals. Moreover, tumor size or symptoms may worsen during the luteal phase of menses or pregnancy. In spite of these observations, the precise role of hormones is unclear. In both sexes, more than 70% of meningiomas express progesterone receptors, and up to 40% estrogen receptors, but nearly 40% express androgen receptors as well.2,5
Biologic Characteristics and Molecular Biology
Meningiomas occur more frequently in certain rare genetic conditions, such as type 2 neurofibromatosis (NF2).4 Mutation in the NF2 gene on chromosome 22q12 is the most common cytogenetic alteration. Nearly all NF2 meningiomas have mutations of the NF2 gene, and most susceptible families have alterations of the NF2 locus.6 Genetic losses of chromosomes 1p, 10, and 14q have been linked with malignant progression or recurrence but have not yet been validated as independent prognostic markers.2,6 Markers of tumor aggressiveness have been studied. MIB-1 expression has been correlated with time to recurrence in some series7 but not in others.8
Pathology and Pathways of Spread
The World Health Organization (WHO) recently published updated grading criteria.5 This system describes 3 grades and 13 histologic subtypes of meningioma (Table 26-1). Strong associations between grade, relapse-free survival (RFS), and overall survival (OS) have been confirmed,5 but even with improved criteria there remain disparities, particularly regarding the proportion of patients with grade II (atypical) histology. A recent large analysis reported that 5% of meningiomas were atypical.3 However, Perry and associates5 found that over 20% of meningiomas were WHO grade II tumors.3 Willis and colleagues9 re-graded patients using modern WHO guidelines and reported that 20.4% were atypical. Another recent analysis found that, whereas 4.4% of meningiomas were categorized as atypical from 1994 to 1999, this percentage has steadily increased to 32.7% to 35.5% since 2004.10
TABLE 26-1 The 2007 World Health Organization (WHO) Criteria for Meningioma Grading
Grade I (Benign Tumor) | Grade II (Atypical Tumor) | Grade III (Anaplastic or Malignant Tumor) |
---|---|---|
hpf, high-power field.
Modified from Perry A, Louis DN, Scheithauer BW, et al: Meningeal tumours. In Louis DN, Ohgaki H, Wiestler OD, Cavenee WK (eds): WHO Classification of Tumours of the Central Nervous System. Lyon, IARC 2007. Modified with the kind assistance of Arie Perry.
The WHO grade is a dominant prognostic factor. Compared with a grade I meningioma, a grade II tumor carries a sevenfold to eightfold increased recurrence risk at 3 to 5 years. Grade III meningiomas are even more aggressive, with a 5-year OS of 32% to 64%.11,12,13,14
Clinical Manifestations, Patient Evaluation, and Staging
Most data regarding the diagnosis and treatment of meningiomas are based on surgical series. As such, there is an inherent bias toward symptomatic tumors. Symptoms depend largely on the location of the lesion but can be influenced considerably by the presence of edema. Skull base meningiomas can present with cranial nerve palsies or neuropathies. Sphenoid wing meningiomas may present with seizures.15
With the increasing use of contrast-enhanced CT and MRI scanning for head trauma, headaches, and so on, the number of incidentally discovered meningiomas has risen. Incidentally discovered meningiomas are often smaller and may show little growth over time.16Although the clinical behavior of incidental meningiomas is not uniformly predictable, younger age and larger size at detection portend increased progression risk.16
Contrast-enhanced MRI is the imaging modality of choice for meningiomas. Tumors at the skull base may also be imaged with CT to evaluate bony invasion, involvement of skull base foramina, or hyperostosis. Additionally, CT may identify calcifications, a finding predictive of more indolent growth.2 MRI is generally superior for visualization of the contrast-enhanced lesion, and MRI T2 signal changes may presage more aggressive behavior.2
Primary Therapy
Tumors with Benign Histologic Findings (WHO Grade I Tumors)
Surgery
Surgery remains the standard of care, and the extent of surgical resection has been correlated with rates of tumor recurrence. In 1957 Simpson reported a series of 265 patients treated surgically, giving rise to the standard system used to describe the extent of resection.1 The Simpson grade of resection and the associated crude recurrence rates are depicted in Table 26-2. Modern series employing current surgical and imaging techniques have validated this association.17
TABLE 26-2 Simpson’s Definitions of “Five Distinct Grades of Operation,” with Respective Recurrence Risk*
Grade | Definition of Resection Extent | Recurrence |
---|---|---|
I | Macroscopically complete removal of tumor, with excision of its dural attachments and any abnormal bone | 9% |
II | Macroscopically complete removal of tumor, with coagulation of its dural attachments | 19% |
III | Macroscopically complete removal of tumor, without resection or coagulation of dural attachments or of extradural extensions (e.g., invaded sinus or hyperostotic bone) | 29% |
IV | Partial removal, leaving tumor in situ | 44% |
V | Simple decompression or biopsy | N/A |
N/A, not applicable.
* Recurrences were identified “in a purely clinical sense to imply the reappearance of symptoms.” Dr. Simpson calculated recurrence risk in a crude fashion, often excluding patients who had had surgery within the prior 5 years.
Modified from Simpson D: J Neurol Neurosurg Psychiatry 20:22-39, 1957.
Postoperative Radiotherapy
Adjuvant radiotherapy is not recommended following gross total resection of a newly diagnosed grade I meningioma. Radiotherapy has often been used after subtotal resection, although considering the absence of randomized trials, differences in clinical practice are to be expected. Many patients are observed after subtotal resection. However, a multitude of retrospective series have reported that radiotherapy improves local control after subtotal resection. Figure 26-1 illustrates progression-free survival (PFS) outcomes from 27 series with long-term results following gross total resection alone, subtotal resection alone, and subtotal resection with external beam irradiation (EBRT).2,18 Some of the series, including many of the recent reports, include patients treated with primary radiotherapy.
Goldsmith and colleagues11 reported a series of 140 patients who received radiotherapy following subtotal resection and identified a dose response. Benign subtotally resected tumors had significantly improved rates of PFS (93% vs. 65% at 10 years) when a dose greater than 52 Gy was delivered. Moreover, the researchers found significantly improved outcome with CT- or MRI-based treatment planning11 (Fig. 26-2). Other studies evaluating radiotherapy after subtotal resection have exhibited superior cause-specific and possibly even OS.19,20
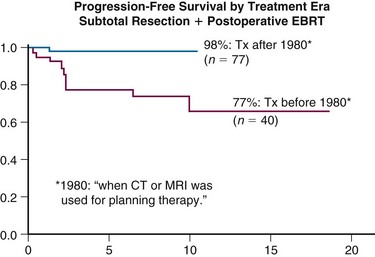
Figure 26-2 Progression-free survival rates of meningioma patients treated with external beam radiotherapy based on treatment era. Treatment planning techniques changed after 1980 with the advent of CT and MRI. Local control has likely improved secondary to better targeting of the tumor.5
Recurrent meningiomas, whether benign or atypical, display a considerably higher rate of recurrence after surgery alone than newly diagnosed tumors.19,20,21 In this setting, postoperative radiotherapy decreases the rate of tumor progression.20,21 In a series by Taylor and associates,20 the local control benefit of postoperative radiotherapy at the time of first recurrence (88% vs. 30% at 5 years) translated into an OS benefit (90% vs. 45% at 5 years).20
Definitive External Beam Radiation Therapy
Early reports of primary radiotherapy revealed inferior results, with local control rates on the order of 47%.22 However, these reports included patients treated in the 1960s and 1970s, before the advent of current imaging and treatment planning paradigms. Many recent series using conformal techniques have corroborated excellent results from definitive radiotherapy with local control in excess of 90% at 5 to 10 years.2,18,23,24 Total doses have ranged from 45 Gy18 to 57.6 Gy,25 typically in 1.8- to 2-Gy fractions. The higher cumulative doses were predominately for higher-grade, large, or recurrent meningiomas. For most patients, modern imaging-based planning and treatment with doses in the range of 50 to 54 Gy with standard fractionation have produced excellent results. Figure 26-3 depicts two examples of tumors appropriate for definitive EBRT.
Optic Nerve Sheath Meningioma
Optic nerve sheath meningiomas represent only 1% to 2% of meningiomas2 but pose considerable clinical challenges owing to their intimate association with the optic nerve and its vasculature. Historically, treatments included resection or observation, both leaving patients with poor functional outcomes. On this account, fractionated radiation therapy is being increasingly used.
Turbin and colleagues26 reported 64 patients managed with surgery alone, surgery plus radiotherapy, radiotherapy alone, or observation. They found that radiotherapy alone resulted in excellent tumor control and was the only modality not leading to worsening of visual acuity. Other series have confirmed excellent outcomes, with stabilization or improvement in visual acuity in up to 90% of patients, with local control exceeding 90%.2,21 Doses of 40 to 54 Gy with fractions of 1.6 to 1.8 Gy have been standard and have produced results more favorable than observation, surgery alone, or surgery plus irradiation.2,26,27
Stereotactic Radiosurgery
Over the past two decades, radiosurgery has become an acceptable and frequently used modality and is generally considered suitable for meningiomas of less than 3 to 4 cm in maximum diameter, with distinct margins, with little or no surrounding edema, and situated at a sufficient distance from critical normal tissues to permit appropriate dose restrictions.17,28 Long-term local control has exceeded 85% in the majority of studies.2
Table 26-3 compares the outcomes for radiosurgery with those of definitive EBRT; this is not a direct comparison but is a compilation of retrospective series reported in the literature and is therefore inherently biased in terms of patient selection. The University of Pittsburgh’s initial publication of long-term outcomes used a median marginal dose of 16 Gy.28 The rate of new neurologic toxicity was 5%. Since this report, it has become evident that lower single doses may be sufficient. In the University of Pittsburgh’s recent update29 of 972 patients, the median marginal dose was 14 Gy. Other reports have shown good local control with doses of 12 Gy. Figure 26-4 illustrates the potential dosimetric advantages of lower marginal doses.
TABLE 26-3 Comparative Analysis of Results with SRS and EBRT by Grade of Meningioma in Multiple Retrospective Series*
Tumor volume has also been shown to be a predictor for success with radiosurgery. DiBiase and colleagues30 reported 5-year disease-free intervals of 91.9% for patients with tumors of 10 cc or smaller versus 68% for larger tumors. Kondziolka and associates28 similarly cited a decreased control rate with larger tumors.
Tumors in Higher-Risk Patients (WHO Grades II and III Tumors)
Tumors with Atypical Histology (WHO Grade II Tumors)
Considerable controversy exists regarding the proper treatment paradigms for grade II meningiomas. These tumors carry considerably higher recurrence risk.19 Although grade II tumors are often treated with combined surgery and postoperative irradiation, this has not been universally adopted, particularly following gross total resection.13
After an incomplete resection, postoperative radiotherapy is typically recommended. This can be either fractionated EBRT or radiosurgery. A relatively higher dose, as compared with the dose for grade I tumors, may be advisable. One study demonstrated improved local control with doses exceeding 53 Gy.11 A recent analysis of combined photon and proton therapy for atypical and anaplastic meningiomas found improved cause-specific (CSS) and (OS) with a total dose exceeding 60 CGE (cobalt Gy equivalent).31
Radiosurgery may also be considered for atypical tumors.32,33 Hakim and colleagues,33 using a median marginal dose of 15 Gy, achieved a 4-year local control rate of 83%. However, caution is to be recommended. Although control of disease within the radiosurgical volume has been acceptable, marginal failures remain problematic.32 Radiosurgery may best be reserved for less aggressive meningiomas or for salvage.
Analyzing 108 atypical meningioma patients after Simpson grade I resection, Aghi and colleagues34 reported a 5-year recurrence rate of 41% following gross total resection alone. Recurrences led to numerous reoperations and to worsened survival rates. Eight of these patients received postoperative fractionated radiotherapy, to a mean dose of 60.2 Gy to the resection bed with a 1-cm margin. Among this small cohort of patients there were no recurrences.34
Tumors with Malignant Histology (WHO Grade III Tumors)
Five-year recurrence rates in two large surgical series ranged from 72% to 78%.14,35 Overall survival rates at 5 years ranged from 32% to 64%. Failure of local tumor control is often a cause of death in patients with these tumors.12,13
Patients with WHO grade III tumors are frequently treated with postoperative radiotherapy; however, given the relative rarity of grade III tumors, this has not been explicitly tested in a clinical trial. A recent series from the Cleveland Clinic reported 13 patients over 23 years, 3 of whom received postoperative irradiation. The report suggested that postoperative radiotherapy improved median survival (5.4 vs. 3.4 years, p = .13) over surgery alone.36 In a publication by Dziuk and associates,37 19 of 38 patients received initial postoperative radiation therapy and experienced significant improvement in 5-year disease-free survival (DFS) rates (80% vs. 15% for surgery alone; p = .002).37 As with grade II tumors, there appears to be a dose response with grade III meningiomas.12,31,38 Boskos and associates31 reported improved OS with doses exceeding 60 Gy and a trend toward further improvement beyond 65 Gy. Nevertheless, caution must be used with aggressive dose escalation. One report of accelerated hyperfractionation revealed a 55% rate of grade 3 to 5 toxicities.
Systemic Therapy
Systemic agents have not had a significant impact on the general management of meningioma to date. Several classes of agents have been evaluated, including hormonal therapy agents, chemotherapy agents, immune modulators, and targeted agents. Hydroxyurea has been used for several years, but radiographic responses are uncommon, and rates of progression for atypical and malignant histologic types have remained high.39
Because a majority of meningiomas express progesterone receptors, a randomized trial of the antiprogestin mifepristone versus placebo was conducted. Mifepristone resulted in increased toxicity but no improvement in response.40 Several recent trials employing novel growth factor agents have been disappointing as well.41 There is a critical need for new approaches to patients who have anaplastic tumors, have failed multiple local therapies, or have diffuse and progressive meningiomatosis. This remains a challenge for future investigation.
Irradiation Techniques and Tolerance
Several methods of target delineation have been described, and there is controversy concerning the optimal method to account for microscopic spread along dura and bone and into the brain. With EBRT, margins as large as 2 cm beyond the gross tumor volume (GTV) have been described using modern imaging.19 However, margins as small as 1 to 2 mm in fractionated stereotactic radiotherapy series have produced good long-term outcome (although the follow-up in such series is shorter, and the patients selected may be inherently different).23,24 Such tight margins may not be safe for meningiomas beyond grade I. Recent reports of radiosurgery for atypical meningiomas have found that increased conformality predicted for a higher rate of local failure.31
Targeting of the dural tail within the clinical target volume (CTV) is also controversial. Surgical series have found that the dural tail is composed almost entirely of hypervascular dura, and is typically an imaging finding, not routinely visualized at surgery.2 The dural tail is not included in the Simpson resection grading scale, and the results of surgery alone have largely been obtained without specific attention to it. Furthermore, radiosurgical series have generally not targeted the dural tail.2,28,42
Inclusion of hyperostotic bone within the CTV is also worthy of discussion. A Simpson grade 1 resection includes gross total resection of tumor, its dural attachments, and any abnormal bone.1 Biopsy series have demonstrated a significant rate of bone involvement by meningioma in cases of hyperostosis,43 and this is associated with a higher rate of recurrence.44 This question needs to be addressed more thoroughly, but it is clearly justifiable to target hyperostotic bone within the treatment volume when this can be accomplished safely.
Toxicities of EBRT
The toxicities associated with EBRT are commonly related to the location. Many patients experience fatigue and some develop alopecia. Late toxicities include cataract formation, cranial nerve palsy or neuropathy, and panhypopituitarism. Rarely, patients experience symptomatic radionecrosis and may require long-term steroid therapy or surgical intervention.11 Debus and colleagues23 reported a series of 189 patients who received radiotherapy with modern fractionation schedules and treatment planning and delivery techniques. The rate of grade 3 toxicities was 2.2%; it was even lower in patients with no preexisting deficit.
Cognitive decline remains a possible late effect. Like most other sequelae, it has site-specific dose-volume relationships. Temporal lobe dose predicts for cognitive decline,45 and large treatment volumes are more likely to lead to symptomatic decline.46 The likelihood of radiation-induced cognitive loss rises over time after brain radiotherapy. Cavernous sinus meningiomas, located just medial to the hippocampus, and sphenoid wing meningiomas, lateral to the hippocampus, may carry a higher risk of cognitive decline.
Toxicities of SRS
The nature of the toxicities of SRS is also dependent on tumor size and location, and most toxicities are related to damage to nerves or to radiation-induced edema. Sensory nerves, such as the optic nerve, are more susceptible to injury than motor nerves. Fraction sizes of 10 Gy or less to the anterior visual pathway carry a 1% to 2% rate of optic neuropathy, but rates rise quickly at doses higher than 10 Gy.42
Edema is a more common complication with single-fraction radiosurgery than with standard fractionation.2,47 Several risk factors have been identified for radiosurgery-induced edema, including higher marginal dose, tumor size greater than 4 cm, presence of pretreatment edema, and periventricular or parasagittal location.2,28,48 A recent series found that parasagittal meningiomas had a greater than fourfold increased risk of peritumoral edema after radiosurgery.49
Treatment Algorithm, Controversies, and Clinical Trials
A treatment algorithm is presented in Figure 26-5. Corresponding dose and treatment volume guidelines are contained in Table 26-4. These depict a literature-based approach defined by risk strata. However, many controversies persist. There is substantial debate concerning which patients to observe, which to treat with primary fractionated radiotherapy or radiosurgery rather than surgery, and which to select for postoperative radiotherapy. Gross total resection of a benign (WHO grade I) meningioma is considered definitive therapy, but there is less agreement about this following subtotal resection of a WHO grade I tumor or after gross total resection of a grade II meningioma. Subtotally resected grade II meningiomas are frequently managed with irradiation, and all grade III meningiomas demand aggressive treatment, irrespective of resection extent. Despite aggressive management, however, current approaches are insufficient for many high-risk patients. New systemic and biologic treatments are needed.