Chapter 49 Antimycobacterial Agents
Abbreviations | |
---|---|
AIDS | Acquired immunodeficiency syndrome |
CNS | Central nervous system |
CSF | Cerebral spinal fluid |
DNA | Deoxyribonucleic acid |
DOT | Directly observed therapy |
GI | Gastrointestinal |
HIV | Human immunodeficiency virus |
IM | Intramuscular |
INH | Isoniazid (isonicotinic acid hydrazide) |
IV | Intravenous |
LTBI | Latent TB infection |
MAC | Mycobacterium avium complex |
MDR | Multi-drug resistance |
PAS | p-Aminosalicylic acid |
POA | Pyrazinoic acid |
PZA | Pyrazinamide |
PZase | Nicotinamidase/pyrazinamidase |
RNA | Ribonucleic acid |
TB | Tuberculosis |
WHO | World Health Organization |
XDR-TB | Extensive drug-resistant TB |
Therapeutic Overview
countries. Overall, it is estimated that one third of the people in the world are currently infected with the TB bacillus. In the United States there was a resurgence of TB between 1985 and 1992, largely because of underfunding and decline of the public health infrastructure. With increased attention and funding, since 1992 there has been a decline in the number of TB cases in the United States to 14,097 TB cases in 2005 (4.8 per 100,000 population). The global epidemic of TB has impacted the United States, where most cases now occur among foreign-born persons. There are great racial/ethnic disparities among case rates. For individuals born in the United States, in 2005, the rate among African-Americans was almost eight times that of Caucasians.
Treatment of TB is much different than treatment of other diseases because of its public health implications. The provider has the responsibility for prescribing an appropriate regimen and ensuring that treatment is completed. Directly observed therapy (DOT) is recommended for all patients with active TB disease and can help ensure higher completion rates (Fig. 49-1), decrease risk for emergence of resistance, and enhance TB control. DOT is generally provided by public health agencies. Active TB should never be treated with a single drug because of the risk of emergence of resistance; therefore multidrug therapy is required. The minimum length of therapy is 6 to 9 months. Patients with drug resistance, especially multidrug resistance (MDR) (i.e., resistance to at least isoniazid and rifampin) require longer therapy. MDR-TB is associated with much higher morbidity and mortality. Drug resistance is an important factor in determining the appropriate therapeutic regimen. Patients who are infected with M. tuberculosis (latent TB infection, or LTBI) are at risk for progressing to active disease. This can be greatly reduced by treating persons with LTBI who are at high or increased risk for progression to active disease (e.g., HIV infection, other illnesses that increase risk of progression, recent infection, or recent immigration from high TB endemic area).
Therapeutic Overview |
---|
Tuberculosis |
Tuberculosis is a huge global health problem; the second leading cause of death due to an infectious disease worldwide. |
More than 8 million new cases and 1.5 million deaths occur annually. |
M. tuberculosis is an aerobic organism. |
M. tuberculosis can cause latent infection and active pulmonary or extrapulmonary disease. |
Combinations of drugs are used for active disease; a single drug can be used for latent infection. |
Long-term treatment is needed (at least 6-9 months). |
Bacterial resistance is of growing importance. |
Multidrug-resistant tuberculosis is associated with increased morbidity and mortality. |
Directly observed therapy is an important component in treatment. |
Leprosy (Hansen’s disease) |
Leprosy is caused by M. leprae, an aerobic acid-fast bacillus organism. |
M. leprae grows extremely slowly with a long incubation period (average 2-4 years). |
It is a chronic disease; clinical manifestations depend upon immune responses. |
Long-term treatment is needed, usually with multidrug therapy. |
Leprosy “reactions” can result from immunologically mediated acute inflammatory responses. |
Mycobacterium avium complex (MAC) disease |
Disseminated MAC most commonly occurs in patients with advanced HIV/AIDS. |
Pulmonary infections are also seen in HIV-seronegative individuals, particularly with underlying or chronic pulmonary disease. |
Treatment requires a multidrug regimen for prolonged periods. |
Prophylaxis is indicated in patients with advanced HIV infection. |
Mechanisms of Action
Inhibition of Protein Synthesis
The rifamycins (rifampin, rifabutin, rifapentine) are bactericidal and inhibit deoxyribonucleic acid (DNA)-dependent ribonucleic acid (RNA) polymerase of mycobacteria (but not mammals). This enzyme is composed of four subunits; rifamycins bind to the β-subunit, which results in blocking the growing RNA chain (Fig. 49-2). Resistance is conferred by single mutations that tend to occur (>95%) in an 81-base pair region of the rpoB gene that codes for the β-subunit. The aminoglycosides streptomycin, kanamycin, and amikacin act by inhibiting protein synthesis and are described in Chapter 47. Capreomycin, a macrocyclic polypeptide antibiotic, has similar activity and toxicities as aminoglycosides.
Inhibition of Cell Wall Synthesis: Isoniazid (INH)
INH is a bactericidal agent that is thought to inhibit mycolic acid synthesis. Mycolic acids are a major constituent of mycobacterial cell walls (along with arabinogalactan and peptidoglycan). The mode of action of INH is complex, and a current model is shown in Figure 49-3. INH is a prodrug that has to be activated by the M. tuberculosis catalase-peroxidase enzyme encoded by the katG gene. Deletions or mutations in this gene may account for 40% to 50% of clinical INH-resistant isolates. Other mechanisms of resistance may include mutations in three other genes. inhA and kasA code for mycolic acid biosynthetic enzymes, and mutations are found in some INH-resistant isolates. ahpC has also been associated with some INH resistance strains, but its role is unclear.
Pyrazinamide (PZA) is active only against M. tuberculosis and M. africanum; it is inactive against M. bovis and nontuberculous mycobacteria. PZA is active at a low pH (e.g., pH 5), is bactericidal, and has excellent sterilizing activity against semidormant bacteria. PZA is thought to enter M. tuberculosis by passive diffusion and is then converted to the active metabolite pyrazinoic acid (POA) by nicotinamidase/pyrazinamidase (PZase). The target of POA may involve fatty acid synthase I and disruption of mycobacterial membranes by acid (Fig. 49-4). Selected mutations in the PZase gene (pncA) are associated with PZA resistance in M. tuberculosis.
Drugs for treatment of leprosy include rifampin, dapsone, clofazimine, ofloxacin, and minocycline. Rifampin is highly bactericidal against M. leprae and is discussed in the previous text. Its mechanism of action is presumed to be inhibition of M. leprae DNA-dependent RNA polymerase. Similar to sulfonamides, dapsone acts as an inhibitor of dihydropteroate synthetase in folate synthesis to produce a bacteriostatic effect. Clofazimine is active against M. leprae (weakly bactericidal), but its mechanism of action is unknown. Ofloxacin, a fluoroquinolone, is discussed in Chapter 48, and the tetracycline minocycline is discussed in Chapter 47.
Anti-M. avium Complex (MAC) Drugs
Clarithromycin and azithromycin have excellent activity against MAC, and these macrolides are the cornerstone of therapy. They can be used in both prevention and treatment of disease (see Chapter 47). Ethambutol is usually combined with clarithromycin (or azithromycin) for treatment of MAC infections, especially among HIV-infected patients with disseminated MAC. Rifabutin can also be used to prevent MAC in HIV-infected patients who are unable to take macrolide drugs, and it can also be used in combination with other agents. Amikacin and the fluoroquinolones also have activity against MAC and are discussed in Chapters 47 and 48, respectively.
Pharmacokinetics
Key pharmacokinetic parameters are summarized in Table 49-1 and in Chapters 47 and 48.
INH is well absorbed orally and is widely distributed, with peak concentrations achieved in pleural, peritoneal, and synovial fluids. Cerebrospinal fluid (CSF) concentrations are approximately 20% of plasma levels but can increase to 100% with meningeal inflammation. INH is metabolized by a liver N-acetyltransferase, and the rate of acetylation determines its concentration in plasma and its half-life. As discussed in Chapter 2, slow acetylation is inherited as an autosomal recessive trait. The average plasma concentration of drug in rapid acetylators is half of that in slow acetylators. However, there is no evidence that these differences are therapeutically important if INH is administered once daily, because plasma levels are well above inhibitory concentrations.
Rifampin is well absorbed orally and widely distributed, achieving therapeutic concentrations in lung, liver, bile, bone, and urine and entering pleural, peritoneal, and synovial fluids and CSF, tears, and saliva. Its high lipid solubility enhances its entrance into phagocytic cells, where it kills intracellular bacteria. Rifampin is metabolized in the liver to a desacetyl derivative that is biologically active. Unmetabolized drug is excreted in bile and reabsorbed from the gastrointestinal (GI) tract into the enterohepatic circulation; the deacetylated metabolite is poorly reabsorbed with eventual elimination in urine and from the GI tract. Rifampin induces its own metabolism by inducing the expression of cytochrome P450s, resulting in increased biliary excretion with continued therapy. Induction of metabolism results in reduction of the plasma life by 20% to 40% after 7 to 10 days of therapy. Patients with severe liver disease may require dose reduction, but dose adjustment is not necessary in renal failure. Oral bioavailability of rifabutin is less than that of rifampin, and the plasma half-life is approximately 10 times greater. Rifabutin is more lipid soluble than rifampin and is extensively distributed. Rifabutin also induces its own metabolism but has less of an effect on cytochrome P450s. Rifapentine is used once weekly in the continuation phase of highly selected patients with TB. It is metabolized in a similar manner but does not significantly induce its own metabolism.
Relationship of Mechanisms of Action to Clinical Response
It is believed that there are three separate subpopulations of M. tuberculosis in the host with TB disease. The first and largest consists of rapidly growing extracellular organisms that mainly reside in well-oxygenated cavities (abscesses) containing 107 to 108 organisms. The second subpopulation consists of poorly oxygenated, closed, solid caseous lesions (e.g., noncaseating granulomas) containing 104 to 105 organisms. These organisms are considered semidormant and undergo only intermittent bursts of metabolic activity. The third subpopulation consists of a small number of organisms (less than 104 to 105) believed to be semidormant within acidic environments—both intracellular (e.g. in macrophages) or extracellular within areas of active inflammation and recent necrosis. INH is most potent in killing rapidly multiplying M. tuberculosis (the first subpopulation) during the initial part of therapy (early bactericidal activity). Rifampin and ethambutol have less early bactericidal activity than INH but considerably more than PZA, which has weak early bactericidal activity during the first 2 weeks of treatment. Drugs with potent early bactericidal activity reduce the chance of resistance emerging. Multidrug therapy is required to prevent development of resistance as a consequence of the selection pressure from administration of a single agent.
Several different regimens are available for treatment of drug-susceptible disease. In addition to the total duration of therapy, the number of completed doses should be counted and tracked to ensure the proper amount of therapy is given. Nonadherence is the most common cause of treatment failure, relapse, and emergence of resistance. DOT has been proven to improve completion rates and outcomes and is recommended for all patients with TB. Administration of anti-TB therapy on an intermittent basis is possible (especially in the continuation phase) for patients with drug susceptible disease and facilitates supervision of therapy. Intermittent therapy (e.g., twice or thrice weekly) should only be given by DOT to patients with drug-susceptible disease. Specific regimens for treatment of active TB disease have been developed (Fig. 49-5) but are beyond the scope of this text.
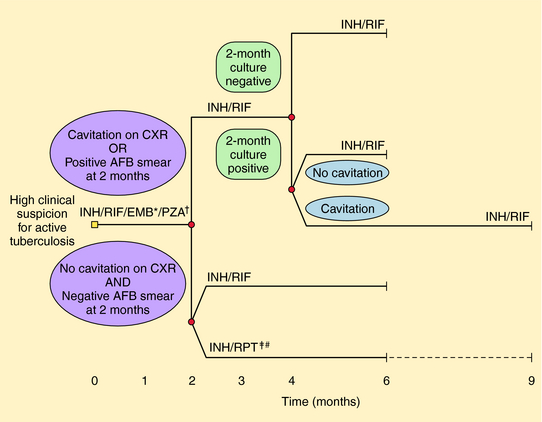
FIGURE 49–5 Treatment algorithm for tuberculosis. Patients in whom tuberculosis is proven or strongly suspected should have treatment with isoniazid (INH), rifampin (RIF), pyrazinamide (PZA), and ethambutol (EMB) for an initial 2 months. A repeat smear and culture should be performed at that time. If cavities were seen on chest radiograph (CXR) or the acid-fast bacillus (AFB) smear is positive after 2 months, the continuation phase should consist of INH and RIF daily or twice-weekly for 4 months to complete a total of 6 months of treatment. If cavitation was present on the initial CXR and the culture at the time of completion of 2 months of therapy is positive, the continuation phase should be lengthened to 7 months (total 9 months). If no cavitation was seen on CXR and there are negative AFB smears at completion of 2 months of treatment, the continuation phase may consist of either once-weekly INH and rifapentine (RPT) or daily or twice-weekly INH and RIF, to complete a total of 6 months (bottom). Patients receiving INH and RPT, and whose 2-month cultures are positive, should have treatment extended by an additional 3 months. Modified from Blumberg H, Burman WJ, Chaisson RE, et al. Am J Respir Crit Care Med 2003; 167:603-662.) * EMB may be discontinued when results of drug susceptibility testing indicate no resistance. † PZA may be discontinued after 2 months. ‡ RPT should not be used in HIV-infected patients with TB or in patients with extrapulmonary TB. # Therapy should be extended to 9 months if 2-month culture is positive.
HIV serologic testing should be offered to all patients with TB. Treatment in patients with HIV is similar to that in other patients, with two major exceptions. The first is that HIV coinfected patients should not be treated with a once-weekly INH-rifapentine regimen in the continuation phase (which is reserved for highly selected HIV seronegative patients without cavitary disease), and HIV-infected patients with CD4+ lymphocyte counts of <100/μL should not receive twice-weekly intermittent regimens (e.g., INH-rifampin or INH-rifabutin) because of increased risk of relapse resulting in rifamycin resistance. As discussed in the following text, there are many drug interactions between rifamycins and other drugs, including antiretroviral agents. Paradoxical or immune reconstitution reactions are more common among HIV-infected patients with TB who are started on antiretroviral therapy early in the course of TB treatment. Therefore some have recommended a delay of initiation of antiretroviral therapy in HIV-infected patients, if possible, until after 1 to 2 months of TB disease therapy. However, data are lacking, and recommendations on the use of antiretroviral therapies in HIV-infected patients with TB continue to evolve. They are available from the Centers for Disease Control and Prevention at www.cdc.gov/nchstp/tb/.
Therapy for LTBI can markedly reduce the risk of progression to active disease and is recommended in those infected with M. tuberculosis who are at increased risk. The tuberculin skin test is the most common diagnostic test, but there is hope that improved tests will become available. The risk of progression from infection to active disease can range from a 5% to 10% lifetime risk in immunocompetent persons to 10% per year in HIV-infected persons with LTBI. HIV/acquired immunodeficiency syndrome (AIDS) is clearly the greatest risk factor. Others include recent infection and LTBI among injection drug users and those with silicosis, diabetes mellitus, renal failure, certain malignancies, gastrectomy or jejunoileal bypass, solid organ transplantation, or use of immunosuppressive drugs. Others at increased risk include immigrants who have arrived in the United States within 5 years from areas with a high incidence of TB, racial/ethnic minorities, children 4 years of age or younger with LTBI, and children and adolescents exposed to high-risk adults. All persons with suspected LTBI should have a chest radiograph performed to exclude active disease. Those with LTBI and risk factors for progression should be encouraged to take LTBI therapy, which generally involves a 9-month course of INH. A course of 6 months of INH is an alternative in HIV-seronegative adults. Rifampin for 4 months is an alternative therapy for adults or those suspected of being infected with an INH-resistant strain of M. tuberculosis. A 2-month short course of rifampin plus PZA for treatment of LTBI is not recommended because of a high rate of hepatotoxicity (although these drugs remain important in multidrug regimens for active TB).
In AIDS patients with disseminated MAC disease, a regimen combining clarithromycin (or azithromycin) (see Chapter 47) with ethambutol is recommended. Rifabutin may be added as a third drug, although data on whether this improves outcome are conflicting. The addition of clofazimine to a multidrug anti-MAC regimen is contraindicated, because it was found to be associated with a worse outcome and higher mortality in HIV-seropositive patients. In treatment of MAC infections in HIV-seronegative immunocompetent patients (e.g., pulmonary MAC), treatment regimens generally include clarithromycin, ethambutol, and rifabutin (or rifampin). Rifabutin is preferred by some because it decreases the serum levels of clarithromycin less than rifampin and may be more active in vitro against MAC. Treatment of MAC disease requires long-term therapy (at least 12 months). Adult and adolescent HIV-infected patients with disseminated MAC should receive lifelong therapy unless immune reconstitution occurs as a consequence of highly active antiretroviral therapy. Among immunocompetent patients with pulmonary MAC, treatment is often recommended for 12 months after sputum conversion.
Pharmacovigilance: Side Effects, Clinical Problems, and Toxicity
Rifampin interacts with many other drugs, usually resulting in increased metabolism and enhanced clearance. It is a potent inducer of cytochrome P450 enzymes, resulting in a number of clinically significant drug-drug interactions (Box 49-1). The concomitant use of anti-TB drugs, including rifampin, and antiretroviral drugs is complex. Rifampin cannot be used with protease inhibitors, although it can be used with nucleoside and some non-nucleoside reverse transcriptase inhibitors. Rifabutin has less of an effect on cytochrome P450s than rifampin and can be used with several protease inhibitors. It is substituted for rifampin when treating HIV-infected patients taking protease inhibitors. The Centers for Disease Control and Prevention has established a website that provides updated information on TB/HIV drug interactions at http://www.cdc.gov/nchstp/tb/tb_hiv_drugs/toc.htm.
Women of child-bearing age should be advised to use alternative contraceptive methods while on rifampin, because oral contraceptives will not be effective.
Adverse effects of rifapentine are similar to those of rifampin. Monitoring is also similar.
Adverse effects of rifampin are discussed earlier in the chapter, and fluoroquinolones are discussed in Chapter 48.
Chemotherapy-Associated Reactions in Leprosy
Patients with leprosy can experience episodic immunologically mediated acute inflammatory responses termed “reactions,” which can cause nerve damage (see Clinical Problems Box). These reactions can be characterized by swelling and edema in preexisting skin lesions, or peripheral neuropathy/neuritis, which can cause pain, tenderness, and loss of function. They occur in up to one third of patients with leprosy and, if not recognized and treated aggressively, can lead to irreversible nerve damage and limb deformity. There are two common types: type I, or reversal, reactions characterized by cellular hypersensitivity; and type 2, or erythema nodosum leprosum, characterized by a systemic inflammatory response to immune complex deposition. In-depth characterization and treatment of these two types of reactions are beyond the scope of this chapter. However, the reversal reactions typically occur after initiation of treatment (especially with dapsone and rifampin) but can occur spontaneously before therapy or after multidrug therapy. A decline in type 2 reactions has been observed since introduction of multidrug therapy and is thought to be due in part to the antiinflammatory effects of daily clofazimine treatment. Recurrences of both types of reactions are common and can result in prolonged use of steroids for suppression.
The main problems posed by most anti-MAC drugs (macrolides, ethambutol) are noted earlier in this chapter and Chapter 47. Rifabutin is generally well tolerated at the lower doses used for prophylaxis against MAC infections, although serious side effects such as uveitis have been reported.
Isoniazid | Hepatotoxicity, peripheral neuropathy, CNS effects |
Rifampin | Orange discoloration of secretions, GI upset, hepatotoxicity, hypersensitivity reactions, many drug interactions, rash |
Pyrazinamide | GI upset, hepatitis, hyperuricemia, arthralgias |
Ethambutol | Optic neuritis |
Capreomycin | Ototoxicity, renal toxicity |
Ethionamide | GI upset, hepatotoxicity |
Cycloserine | Psychosis, seizures, headache, depression, other CNS effects |
PAS | GI upset, hypersensitivity, hepatotoxicity, drug interactions |
Blumberg HM, Burman WJ, Chaisson RE, et al. American Thoracic Society/Centers for Disease Control and Prevention/Infectious Diseases Society of America: Treatment of tuberculosis. Am J Respir Crit Care Med. 2003;167:603-662. (Also published as: Centers for Disease Control and Prevention. Treatment of Tuberculosis, American Thoracic Society, CDC, and Infectious Diseases Society of America. MMWR 2003; 52(No. RR-11):1-77)
Benson CA, Williams PL, Currier JS, et al. AIDS Clinical Trials Group 223 Protocol Team. A prospective, randomized trial examining the efficacy and safety of clarithromycin in combination with ethambutol, rifabutin, or both for the treatment of disseminated Mycobacterium avium complex disease in persons with acquired immunodeficiency syndrome. Clin Infect Dis. 2003;37:1234-1243.
Boggild AK, Keystone JS, Kain KC. Leprosy: A primer for Canadian physicians. CMAJ. 2004;170:71-78.
National Center for HIV/AIDS, Viral Hepatitis, STD and TB Prevention: Division of Tuberculosis Elimination. TB Guidelines. http://www.cdc.gov/tb/pubs/mmwr/maj_guide.htm. 4/18/2007 (Accessed on September 2, 2007).
Neurmberger E, Grassert J. Pharmacokinetics and pharmacodynamic issues in the treatment of mycobacterial infections. Eur J Clin Microbiol Infect Dis. 2004;23:243-255.