Chapter 14 Autonomic Innervation of Ocular Structures
Autonomic Pathway
Ocular structures supplied by the sympathetic system are the iris dilator, ciliary muscle, smooth muscle of the lids, lacrimal gland, and choroidal and conjunctival blood vessels.1–5 Ocular structures supplied by the parasympathetic system are the iris sphincter, ciliary muscle, lacrimal gland, and blood vessels.
Sympathetic Pathway to Ocular Structures
Sympathetic fibers are controlled by the hypothalamus through a pathway that terminates in the lateral column of the cervical spinal cord. The fiber from the preganglionic neuron leaves the spinal cord in one of the first three thoracic nerves via the ventral root and enters the sympathetic ganglion chain located adjacent to the vertebrae (Figure 14-1). These preganglionic fibers then ascend in the sympathetic chain to a synapse in the superior cervical ganglion, located near the second and third vertebrae.6,7
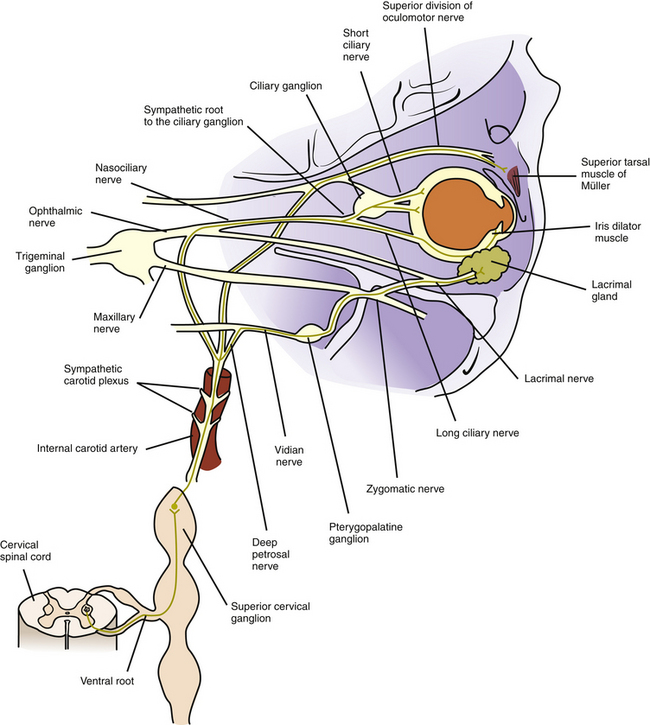
FIGURE 14-1 Sympathetic innervation to iris dilator, Müller muscle, blood vessels, and lacrimal gland.
Some of these sympathetic fibers travel with the ophthalmic division of the trigeminal nerve from the cavernous sinus into the orbit.8 Once in the orbit the sympathetic fibers follow the nasociliary nerve and then travel with the long ciliary nerves to innervate the iris dilator and the ciliary muscle8–12 (see Figure 14-1).
Other fibers from the carotid plexus follow this same route to the nasociliary nerve and then branch to the ciliary ganglion as the sympathetic root; these fibers pass through the ganglion without synapsing. They enter the globe as the short ciliary nerves to innervate the choroidal blood vessels. Alternately, the sympathetic root to the ciliary ganglion may emanate directly from the internal carotid plexus.12,13 A sympathetic nerve network accompanies the ophthalmic artery and its branches could have a role in the control of blood flow to ocular structures.14 The pathway to the conjunctival vasculature may be through either the long or the short ciliary nerves.
Other fibers from the carotid plexus join the oculomotor nerve and travel with it into the orbit to innervate the smooth muscle of the upper eyelid. These fibers follow the same path as the superior division of the oculomotor nerve as it supplies the levator muscle8 (see Figure 14-1). An alternate route to Müller’s muscle from the infratrochlear or lacrimal nerve has been suggested.14
Sympathetic stimulation activates the iris dilator, causing pupillary dilation and thereby increasing retinal illumination. It also causes vasoconstriction of the choroidal and conjunctival vessels and widening of the palpebral fissure by stimulating the smooth muscle of the eyelids. The sympathetic nerves also exhibit a small inhibitory effect on the ciliary muscle.1–3,5,15–18
Parasympathetic Pathway to Ocular Structures
The preganglionic neuron in the parasympathetic pathway to the intrinsic ocular muscles is located in the midbrain in the parasympathetic accessory third-nerve nucleus, also called the Edinger-Westphal nucleus. The preganglionic fibers leave the nucleus with the motor fibers of the oculomotor nerve and follow the inferior division of that nerve into the orbit.19 The parasympathetic fibers leave the inferior division and enter the ciliary ganglion as the parasympathetic root13,20–22 (Figure 14-2).
The ciliary ganglion is a small, somewhat flat structure, 2 mm long and 1 mm high, located within the muscle cone between the lateral rectus muscle and the optic nerve, approximately 1 cm anterior to the optic canal.9,13,23 Three roots are located at the posterior edge of the ganglion: the parasympathetic root, mentioned previously; the sensory root, which carries sensory fibers from the globe and joins with the nasociliary nerve; and the sympathetic root, which supplies the blood vessels. Only the parasympathetic fibers synapse in the ciliary ganglion; the sensory and sympathetic fibers pass through without synapsing (see Figure 14-2).
The short ciliary nerves, located at the anterior edge of the ciliary ganglion, carry sensory, sympathetic, and parasympathetic fibers. The postganglionic parasympathetic fibers, which are myelinated,20 exit the ganglion in the short ciliary nerves, enter the globe, and travel to the anterior segment of the eye to innervate the sphincter and ciliary muscles. Most of the fibers innervate the ciliary body; only approximately 3% supply the iris sphincter.20,21 The two groups of neurons likely share some characteristics and differ in others, but specifics have not been identified.24
Clinical Comment: Inhibition of Ciliary Muscle
Parasympathetic activation causes contraction of the ciliary muscle in accommodation. Many investigators, using pharmacologic,25,26 electrophysiologic,27 and anatomic20,28,29 evidence, have demonstrated the presence of both sympathetic receptors and fibers in animals and humans.30,31 The sympathetic effect on the ciliary muscle appears to be a small, slow inhibition that is a function of the level of parasympathetic activity.1–5
Autonomic Innervation to Lacrimal Gland
The efferent autonomic pathway to the lacrimal gland follows a complex route. Fibers controlling the parasympathetic innervation originate in the pons in an area within the nucleus for cranial nerve VII designated as the lacrimal nucleus. These preganglionic fibers exit the pons with the motor fibers of the facial nerve, enter the internal auditory canal, and pass through the geniculate ganglion of the facial nerve without synapsing. They leave the ganglion as the greater petrosal nerve, which exits the petrous portion of the temporal bone.32 The greater petrosal nerve is joined by the deep petrosal nerve, composed of sympathetic postganglionic fibers from the carotid plexus. The greater petrosal and the deep petrosal nerves together form the vidian nerve (nerve of the pterygoid canal) (see Figures 14-1 and 14-2).
The vidian nerve enters the pterygopalatine ganglion, where the parasympathetic fibers synapse. The pterygopalatine ganglion (also called the sphenopalatine ganglion) lies in the upper portion of the pterygopalatine fossa (see Figure 12-5). It is a parasympathetic ganglion because it contains parasympathetic cell bodies and synapses; sympathetic fibers pass through without synapsing.
The autonomic fibers (all of which are now postganglionic) leave the ganglion, join with the maxillary branch of the trigeminal nerve, pass into the zygomatic nerve, and then form a communicating branch to the lacrimal nerve (see Figures 14-1 and 14-2). An alternate pathway bypasses the zygomatic nerve and travels from the ganglion directly to the gland.33 The parasympathetic fibers that innervate the lacrimal gland are of the secretomotor type and thus cause increased secretion. The sympathetic fibers innervate the blood vessels of the gland and might indirectly cause decreased production of lacrimal gland secretion by restricting blood flow.14 Parasympathetic stimulation causes increased lacrimation. Figure 14-3 provides a flow chart of the common autonomic nerve pathways to orbital structures. Sympathetic fibers from the zygomatic nerve also branch into the lower eyelid to innervate Müller’s muscle of the lower lid.34
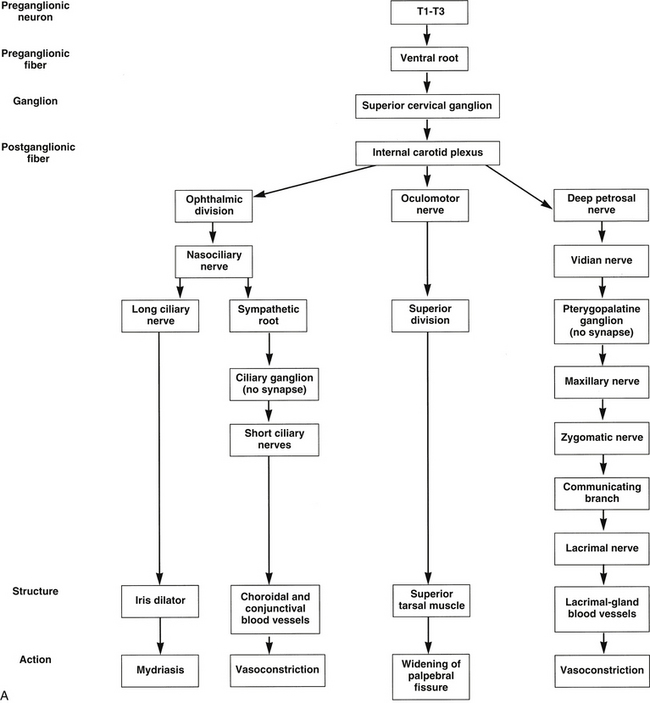
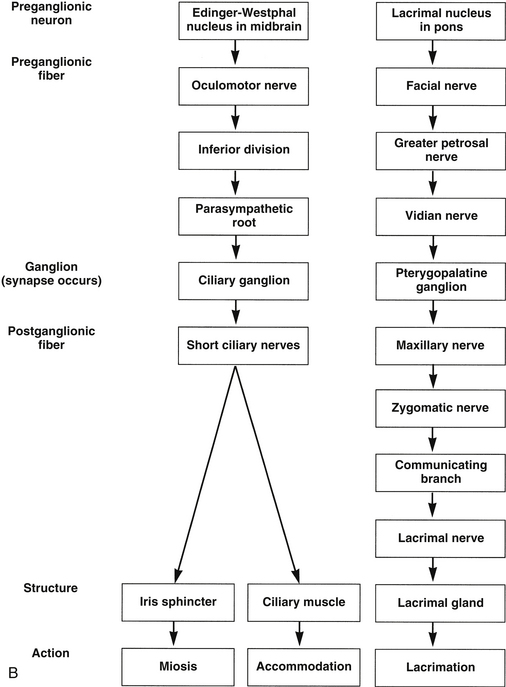
FIGURE 14-3 Flow chart of autonomic nervous system. A, Sympathetic innervation. B, Parasympathetic innervation.
Parasympathetic innervation to the choroidal blood vessels is believed to emanate directly from the sphenopalatine ganglion through a network of fine nerves, the rami oculares.35 Parasympathetic activation presumably causes vasodilation, which might raise intraocular pressure.33,36
Irritation of any branch of the trigeminal nerve activates a reflex afferent pathway, precipitating increased lacrimation.7,37
Clinical Comment: Corneal Reflex
Corneal touch initiates the three-part corneal reflex: lacrimation, miosis, and a protective blink (Figure 14-4). The pain sensation elicited by the touch travels to the trigeminal ganglion and then into the pons as the trigeminal nerve. Communication from the trigeminal nucleus to the Edinger-Westphal nucleus causes activation of the sphincter muscle. Communication to the facial nerve nucleus activates the motor pathway to the orbicularis muscle, causing the blink, and communication to the lacrimal nucleus and the parasympathetic pathway to the lacrimal gland stimulates increased lacrimation.
Pharmacologic Responses of Intrinsic Muscles
Neurotransmitters
When an action potential reaches the terminal end of an axon, a neurotransmitter is released that activates either the next fiber in the pathway or the target structure, the effector. In the sympathetic pathway the neurotransmitter released by the preganglionic fiber is acetylcholine, and the neurotransmitter released by the postganglionic fiber is norepinephrine. In the parasympathetic system both preganglionic and postganglionic fibers secrete acetylcholine (Figure 14-5). Fibers that release acetylcholine are called cholinergic, and fibers that release norepinephrine are called adrenergic.
Drugs: Agonists and Antagonists
Ophthalmic Agonist Agents
Epinephrine and phenylephrine are direct-acting adrenergic agonists that bind to sites on the dilator muscle, causing contraction38 (Figure 14-6). Hydroxyamphetamine and cocaine are indirect-acting adrenergic agonists. Hydroxyamphetamine causes the release of norepinephrine from the nerve ending, thus indirectly initiating muscle contraction. Cocaine prevents the reuptake of norepinephrine by the nerve ending; thus norepinephrine remains at the neuromuscular junction and can continue to activate the dilator.38
Pilocarpine is a direct-acting cholinergic agonist that directly stimulates the sites on the iris sphincter and ciliary muscle, causing contraction38 (Figure 14-7). Physostigmine is an indirect-acting cholinergic agonist that inhibits acetylcholinesterase.38 Therefore, acetylcholine is not broken down but remains in the junction, and the sphincter and ciliary muscle contraction continues in a spasm.
Ophthalmic Antagonist Agents
Dapiprazole is an adrenergic antagonist that blocks receptor sites, thereby preventing norepinephrine from activating the dilator muscle. Atropine, cyclopentolate, and tropicamide are cholinergic antagonists that compete with acetylcholine by blocking sphincter and ciliary muscle sites, thereby inhibiting miosis and accommodation38 (Figure 14-8).
Accommodation-Convergence Reaction (Near-Point Reaction)
The accommodation-convergence reaction is not a true reflex but rather a synkinesis or an association of three occurrences: convergence, accommodation, and miosis. As an object is brought near along the midline, the medial rectus muscles contract to move the image onto each fovea; the ciliary muscle contracts to keep the near object in focus; and the sphincter muscle constricts to decrease the size of the pupil, thereby improving depth of field.
Each of these actions can occur without the others. If plus lenses are placed in front of each eye, pupillary constriction and convergence occur without accommodation. If a base-in prism is placed in front of each eye, pupillary constriction and accommodation occur without convergence.39
The afferent pathway for this reaction follows the visual pathway to the striate cortex. From the striate cortex, information is sent to the frontal eye fields, which communicate with the oculomotor nucleus and the Edinger-Westphal nucleus through a pathway that passes through the internal capsule (Figure 14-9). The efferent pathway, via the oculomotor nerve, innervates the medial rectus muscle, and the parasympathetic pathway innervates the ciliary muscle and iris sphincter.
Pupillary Light Pathway
An understanding of the pupillary light pathway can be an important tool in diagnosing clinical problems with pupillary manifestations. Shining a bright light into an eye normally will initiate pupillary constriction. The afferent fibers that carry this information are called pupillary fibers, to distinguish them from visual fibers, which carry visual information. For some time it was unclear whether there were two separate sets of fibers or whether the pupillary fibers were branches of the visual fibers.39 It now seems that both theories are somewhat correct. One classification model groups retinal ganglion cells as W, X, and Y cells. The W cells project to the pretectum, carrying pupillary afferent information, and project to the colliculi, carrying information for reflexive eye movements. The Y cells send collateral branches to the pretectum and to the colliculi. The X cells primarily carry visual information to the lateral geniculate nucleus, with a few having collateral fibers projecting to the midbrain.24
The afferent pupillary light pathway parallels the visual pathway as far as the posterior optic tract, with the nasal fibers crossing in the chiasm. The pupillary fibers exit in the posterior third of the optic tract and travel within the brachium of the superior colliculus to an area of the midbrain known as the pretectal nucleus, located near the superior colliculus. Synapse occurs, and the fibers that leave the pretectal region travel to the two Edinger-Westphal nuclei, distributing about equally to both.39 The fibers that cross to the opposite Edinger-Westphal nucleus travel in the posterior commissure (Figure 14-10).
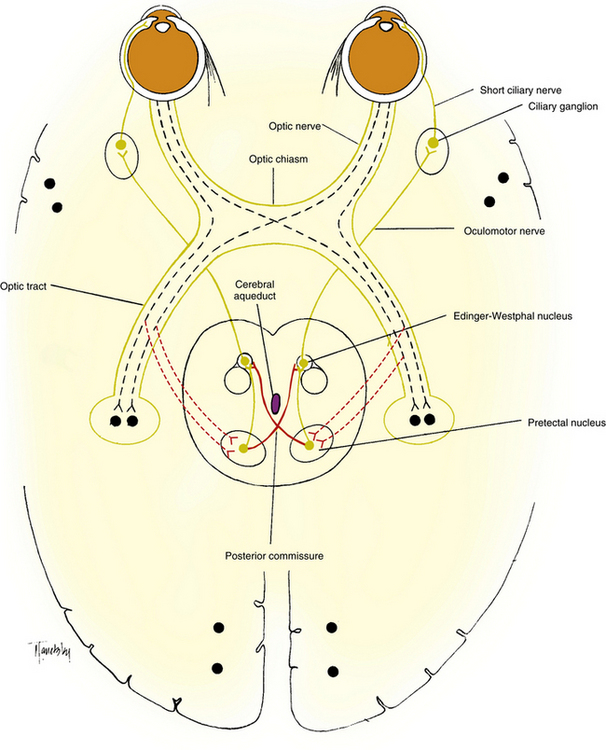
FIGURE 14-10 The pupillary light pathway. Dotted lines indicate the afferent pathway and solid lines the efferent pathway.
The efferent parasympathetic pathway from the Edinger-Westphal nucleus to the sphincter and ciliary muscles is described earlier under the Parasympathetic Pathway to Ocular Structures section. As the third nerve leaves the midbrain, the pupillomotor fibers generally lie in a superior position; but as the nerve leaves the cavernous sinus and enters the orbit, the pupillomotor fibers move into an inferior position and travel in the inferior division of the oculomotor nerve.39
While the parasympathetic system is activated, an inhibition of the dilator muscle apparently occurs. When light is removed from the eye and the Edinger-Westphal neurons stop firing, the preganglionic sympathetic fibers are no longer inhibited, their firing rate increases, and the dilator muscle increases in tone.24 The fibers that carry the inhibition message from the retina likely pass through an accessory optic system to the cervical spinal cord. There is similar inhibition of the parasympathetic innervation while the sympathetic nerves cause dilator contraction. These inhibitory fibers course through the midbrain.24
Disruption in the Afferent Pathway
Disruption can occur anywhere in the afferent pathway: retina, optic nerve, chiasm, optic tract, or superior brachium. The swinging-flashlight test can be used to determine the presence of an RAPD. Damage posterior to the crossing in the chiasm might not be evident with the swinging-flashlight test unless the damage affects a great number of fibers from one eye and significantly fewer fibers from the other eye.40 There are more crossed (contralateral) fibers in the optic tract than uncrossed (ipsilateral); therefore, with a complete optic tract lesion the pupillary constrictions may be greater with light into the ipsilateral eye than with light into the contralateral eye.
Clinical Comment: Swinging-Flashlight Test
The patient is asked to fixate on a distant object, and then the practitioner swings a light from eye to eye, several times rhythmically, taking care to illuminate each pupil for an equal length of time, about 2 or 3 seconds. If both pathways are normal, little or no change in pupil size will be noted; the eye will not recover from the consensual response before it is subjected to the direct light beam. The normal, symmetric response is characterized by equal pupillary constriction in both eyes when the light is presented to either eye. An abnormal response is characterized by larger pupils when the light is directed into the affected eye than when the light is directed into the normal eye (Figure 14-11).
As the intensity of the light increases, stronger constrictions occur when light is presented to a normal eye. There is a threshold, however, beyond which no increase occurs. A very bright light can be used for detecting subtle defects; however, the luminance level should be recorded because a future change in the measured RAPD might reflect only a different light condition.41,42
Clinical Comment: Afferent Pupillary Defect in Cataract
It would appear likely that a dense cataract would cause an RAPD because less light penetrates a cataract to stimulate the retina. In a clinical situation, however, a dense cataract has been found to cause an RAPD in the contralateral eye.43,44 Light scattered back to the retina from the lens opacity probably produces an enhanced pupillary response, which is manifested as an RAPD of the contralateral eye.
Disruption within the Central Nervous System
Injury to the dorsal tegmentum of the midbrain, that interrupts the fibers from the pretectal nucleus to the parasympathetic third-nerve nucleus, if limited to one side and affecting all fibers into the Edinger-Westphal nucleus, results in a pupil that shows a poor direct and consensual response but does constrict with the near response. This pupillary response is commonly called the Argyll Robertson pupil and is said to show a “light-near dissociation.45” Since the fibers carrying the message for the near reaction approach the Edinger-Westphal nucleus from a more ventral location, they do not pass through the affected area of the midbrain. Since the pathway from the frontal eye fields is intact and the efferent path is viable, the sphincter and ciliary muscle still will constrict to a near object.46 In the Argyll Robertson pupil, the retained near response exceeds the best direct-light response (Figure 14-12), and when the patient looks from near to distant, the pupils redilate briskly.
Because the fibers that carry inhibitory feedback to the parasympathetic nucleus also pass through the dorsal midbrain, miosis is a component of the Argyll Robertson syndrome and is evident in darkness, with the affected pupil smaller than would be seen in the normal individual.24 The Argyll Robertson syndrome is bilateral in approximately 80% to 90% of cases, but the two sides may be affected unequally.24 Diabetic neuropathy, alcoholic neuropathy, or neurosyphilis is suspected in a complete Argyll Robertson pupil that involves both sides. A near response that exceeds the light response is always a sign of a pathologic pupil.24
Disruption in the Efferent Pathway
A lesion in the efferent pathway will cause the eye to show poor direct and consensual pupillary responses and a poor near response. The pupil appears large on clinical presentation, and other ocular structures are involved. Damage in the oculomotor nucleus or nerve could involve the superior rectus, medial rectus, inferior rectus, inferior oblique, or levator muscle, and the patient should be examined for related ocular motility impairment. The parasympathetic fibers in the oculomotor nerve are often spared in ischemic lesions, as from diabetes, but are especially vulnerable to compression because the fibers are superficial as the nerve emerges from the midbrain.24 Third nerve involvement that includes a dilated pupil is highly suspicious of a compressive intracranial lesion.
Damage to the ciliary ganglion or the short ciliary nerves could be caused by local injury or disease and results in a tonic pupil, which is characterized by poor pupillary light response and loss of accommodation. Decreased corneal sensitivity often occurs because some afferent sensory fibers from the cornea pass through the short ciliary nerves and the ganglion.47 The affected muscle may exhibit cholinergic denervation supersensitivity, a physiologic phenomenon resulting from injury to the fibers directly innervating muscles.48 The near response is retained, but it is delayed and slow, and the pupil redilates sluggishly. One theory postulates that because the density of the innervation to the ciliary muscle is much greater than the density of innervation to the sphincter, some ciliary muscle nerve fibers remain intact. With near stimulation, these fibers release acetylcholine, which diffuses into the aqueous humor and then causes the supersensitive sphincter to constrict.49 In late stages of this condition, the pupil becomes tonic and the miotic near reaction difficult to demonstrate, but the accommodative facility appears to recover, perhaps as a result of regeneration of the fibers.20,50
Clinical Comment: Adie’s Tonic Pupil
If the cause of the tonic pupil is not apparent, the syndrome is called Adie’s tonic pupil. The typical patient with Adie’s pupil is a woman 20 to 40 years of age; 90% of these patients also have diminished tendon reflexes. Because of this systemic manifestation, it is believed that similar degenerative processes are occurring in the ciliary ganglion and in the dorsal column of the spinal cord,51 but the cause is unknown.52 If pupillary constriction in early Adie’s pupil is examined with the biomicroscope, segmental constriction affecting only a section of the iris may be evident.53 An Adie’s pupil that has been tonic for years eventually becomes smaller and does not dilate well in the dark; thus it is the larger pupil in light and the smaller one in darkness.50
In the differential diagnosis of Adie’s pupil, a very mild, direct-acting cholinergic agonist can be used because the sphincter muscle is supersensitive.20,54 A dilute concentration of pilocarpine (0.125%) has minimal effect on a normal sphincter but will cause significant clinical miosis in a supersensitive sphincter. Some investigators believe that a normal pupil can respond to the 0.125% pilocarpine and recommend using a 0.0625% pilocarpine solution.55 With one drop instilled in each eye, the Adie’s pupil should show a much greater constriction than the normal pupil (Figure 14-13).
There is indication that a tonic pupil might in some cases be associated with autoimmune disease.56
Disruption in the Sympathetic Pathway
An interruption in the sympathetic pathway causes miosis. The usual tone that the dilator muscle normally exerts is not present, and there is no counteracting pull against the sphincter muscle, making the pupil smaller than normal. Anisocoria (a difference in pupil size) is present under normal room light conditions but is more pronounced in dim light, with the normal eye having the larger pupil. The pupil responds briskly to light, but with slow and incomplete dilation in the dark. If the anisocoria decreases in bright lights and the pupils react normally to a light stimulus, the disruption is likely a sympathetic interruption to the dilator muscle or benign anisocoria.50
Clinical Comment: Benign Anisocoria
Approximately 20% of the population have benign anisocoria (also called simple or physiologic anisocoria), which is usually more apparent in dim light than in room light, with the difference between pupils usually less than 1 mm.50 Sometimes the anisocoria may switch sides, but both pupils are round and react well to all stimuli and dilate equally with the lights off. It represents an asymmetric balance between the sympathetic and parasympathetic innervation to the iris. Benign anisocoria may be caused by asymmetric supranuclear inhibition of the Edinger-Westphal nucleus.50
Clinical Comment: Horner’s Syndrome
Clinical Features
Damage in the sympathetic pathway to the head can cause Horner’s syndrome, which consists of ptosis, miosis, and facial anhidrosis (absence of sweat secretion). Loss of innervation to the smooth muscle of the upper eyelid causes ptosis, whereas loss of innervation to the lower eyelid causes it to rise slightly such that the palpebral fissure appears narrow, simulating enophthalmos (Figure 14-14).
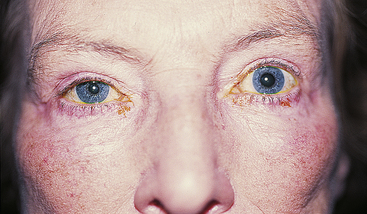
FIGURE 14-14 Ptosis and miosis in Horner’s syndrome.
(From Kanski JJ, Nischal KK: Ophthalmology: clinical signs and differential diagnosis, St Louis, 1999, Mosby.)
The preganglionic fibers leave the dorsal column of the spinal cord, pass into the chest, course over the apex of the lung, and loop around the subclavian artery en route to the superior cervical ganglion (Figure 14-15). These fibers can be damaged in thoracic injury or surgery or in metastatic disease involving the chest.6
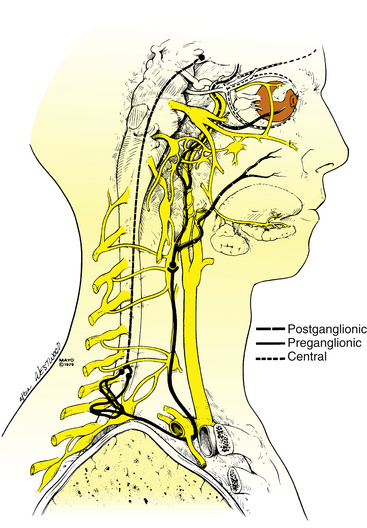
FIGURE 14-15 Sympathetic innervation of the eye.
(From Maloney WF, Younge BR, Moyer NJ: Evaluation of the causes and accuracy of pharmacologic localization in Horner’s syndrome, Am J Ophthalmol 90:394, 1980. With permission from the Mayo Foundation.)
The postganglionic fibers that enter the skull through the carotid plexus can be damaged by a fracture of the skull base or an injury to the internal carotid artery. Painful Horner’s syndrome is a classical symptom of carotid artery dissection and should be treated as an emergent situation.56 Horner’s in combination with a sixth nerve paresis indicates cavernous sinus involvement and a mass in or near the sinus must be ruled out.56 Damage along the rest of the postganglionic neuron can involve the nasociliary or long ciliary nerves.
Iris Heterochromia in Horner’s Pupil
Normal sympathetic innervation is necessary for the development and maintenance of iris melanocyte pigmentation. In congenital Horner’s syndrome, normal iris pigmentation fails to develop, and heterochromia is present (Figure 14-16). Heterochromia is rarely seen in acquired Horner syndrome but may develop after long-standing conditions.24
Differential Diagnosis
In addition to the clinical presentation of ptosis and miosis, dilation lag occurs in dim illumination, and this will differentiate Horner’s pupil from simple anisocoria. The normal pupil dilates within 5 seconds of lights being off because of the normal sympathetic activity to the dilator and the parasympathetic inactivation of the sphincter. In the Horner’s pupil there is no sympathetic activity, and the pupil thus dilates only from inactivation of the sphincter muscle and dilates more slowly, taking 10 to 20 seconds.50,56 Dilation lag does not occur with physiologic anisocoria.56
The location of the disruption of the sympathetic pathway is useful in determining appropriate care. In the differential diagnosis of Horner’s syndrome, diagnostic drugs used to determine the site of interruption include cocaine and hydroxyamphetamine, the effects of which are shown in Figures 14-17 and 14-18. If the sympathetic pathway is intact, instillation of one drop of a 5% or 10% ophthalmic cocaine solution, an indirect-acting adrenergic agonist, causes dilation in 30 to 60 minutes.50,57,58 In contrast, with a disruption anywhere in the pathway, norepinephrine is lacking in the neuromuscular junction, and therefore cocaine has little or no effect, and the pupil dilates poorly.
Hydroxyamphetamine 1% can be administered to determine whether the damage is in the preganglionic or postganglionic pathway.59–61 A topical administration of this indirect-acting adrenergic agonist acts on the postganglionic fiber, causing release of norepinephrine. If the lesion is in the preganglionic pathway, the postganglionic fiber still is viable and will contain stores of norepinephrine. Instillation of hydroxyamphetamine will cause release of the neurotransmitter, and dilation will occur. If the damage is in the ganglion or the postganglionic fiber, norepinephrine will not be stored in the nerve endings, and therefore no dilation will occur with instillation of hydroxyamphetamine. The instillation should occur 24 to 48 hours after the cocaine test, and dilation may take up to an hour.50,62
In the presence of preganglionic and central lesions, the pupil on the affected side usually dilates more than the normal eye with hydroxyamphetamine instillation, either because of enhanced receptor sensitivity or because the adrenergic nerve endings have accumulated more norepinephrine.62,63 In adults, central Horner’s syndrome is often related to stroke, preganglionic Horner’s often is associated with neoplasm, and postganglionic Horner’s syndrome may have a vascular cause.50
An alternative drug might be used in diagnosing postganglionic involvement because the dilator muscle could be supersensitive to a sympathomimetic and a solution of 1% phenylephrine could cause contraction. In the normal pupil, 1% phenylephrine will generally cause only minimal dilation but in some clinical patients with a postganglionic lesion, the Horner’s pupil was found to respond sooner and more vigorously than the unaffected pupil.64 With a preganglionic lesion, the Horner’s pupil would be expected to only dilate minimally although validation with published findings has yet to occur.
1. Olmsted J.M.D., Morgan M.W. The influence of the cervical sympathetic nerve on the lens of the eye. Am J Physiol. 1941;133:720.
2. Olmsted J.M.D. The role of the autonomic nervous system in accommodation for near and far vision. J Nerv Ment Dis. 1944;99:794.
3. Morgan M.W. The nervous control of accommodation. Am J Optom. 1944;21:87.
4. Gilmartin B. A review of the role of sympathetic innervation of the ciliary muscle in ocular accommodation. Ophthalmic Physiol Opt. 1986;6(1):23.
5. Rosenfield M., Gilmartin B. Oculomotor consequences of beta-adrenoceptor antagonism during sustained near vision. Ophthalmic Physiol Opt. 1987;7(2):127.
6. Maloney W.F., Younge B.R., Moyer N.J. Evaluation of the causes and accuracy of pharmacologic localization in Horner’s syndrome. Am J Ophthalmol. 1980;90:394.
7. Doxanas M.T., Anderson R.L. Clinical orbital anatomy. Baltimore: Williams & Wilkins; 1984. pp 93, 131
8. Pick T.P., Howden R., editors. Gray’s anatomy, ed 15. New York: Crown; 1977:799.
9. Warwick R. Eugene Wolff’s anatomy of the eye and orbit, ed 7. Philadelphia: Saunders; 1976. p 306
10. Mohney J.B., Morgan M.W., Olmsted J.M.D., et al. The pathway of sympathetic nerves to the ciliary muscles in the eye. Am J Physiol. 1942;135:759.
11. Ruskell G.L. Sympathetic innervation of the ciliary muscle in monkeys. Exp Eye Res. 1973;16:183.
12. Natori Y., Rhoton A.L. Microsurgical anatomy of the superior orbital fissure. Neurosurgery. 1995;36:762-775.
13. Izci Y., Gonul E. The microsurgical anatomy of the ciliary ganglion and its clinical importance in orbital traumas: an anatomic study. Minim Invasive Neurosurg. 2006;49:156-160.
14. Thakker M.M., Huang J., Possin D.E., et al. Human orbital sympathetic nerve pathways. Ophthal Plast Reconstr Surg. 2008;24:360-366.
15. Gilmartin B., Hogan R.E. The relationship between tonic accommodation and ciliary muscle innervation. Invest Ophthalmol Vis Sci. 1985;26:1024.
16. Gilmartin B., Bullimore M.A., Rosenfield M., et al. Pharmacological effects on accommodative adaptation. Optom Vis Sci. 1992;69(4):276.
17. Winn B., Culhane H.M., Gilmartin B., et al. Effect of ß-adrenoceptor antagonists on autonomic control of ciliary smooth muscle. Ophthalmic Physiol Opt. 2002;22(5):359.
18. Gilmartin B., Mallen E.A.H., Wolffsohn J.S. Sympathetic control of accommodation: evidence for inter-subject variation. Ophthalmic Physiol Opt. 2002;22(5):366.
19. Warwick R. The ocular parasympathetic nerve supply and its mesencephalic sources. J Anat. 1954;88:71.
20. Ruskell G.L. Accommodation and the nerve pathway to the ciliary muscle: a review. Ophthalmic Physiol Opt. 1990;10(3):239.
21. Burde R.M. Direct parasympathetic pathway to the eye: revisited. Brain Res. 1988;463:158.
22. Reiner A., Erichsen J.T., Cabot J.B., et al. Neurotransmitter organization of the nucleus of Edinger-Westphal and its projection to the avian ciliary ganglion. Vis Neurosci. 1991;6(5):451.
23. Duke-Elder W. The anatomy of the visual system, vol 2, System of ophthalmology. St Louis: Mosby; 1961. p 497
24. Loewenfeld I.E. The pupil: anatomy, physiology, and clinical applications. Boston: Butterworth-Heinemann; 1999.
25. Tornqvist G. The relative importance of the parasympathetic and sympathetic nervous systems for accommodation in monkeys. Invest Ophthalmol Vis Sci. 1967;6:612.
26. Hurwitz B.S., Dacidowitz J., Chin N.B., et al. The effects of the sympathetic nervous system on accommodation. I. Beta sympathetic nervous system. Arch Ophthalmol. 1972;87:668.
27. Tornqvist G. Effect of cervical sympathetic stimulation on accommodation in monkeys. An example of a beta-adrenergic inhibitory effect. Acta Physiol Scand. 1967;67:363.
28. Van Alphen G.W. The adrenergic receptors of the intraocular muscles of the human eye. Invest Ophthalmol Vis Sci. 1976;15:502.
29. Wax M.B., Molinoff P.B. Distribution and properties of beta-adrenergic receptors in human iris/ciliary body. Invest Ophthalmol Vis Sci. 1984;25(Suppl):305. (ARVO abstract)
30. Stephens K.G. Effect of the sympathetic nervous system on accommodation. Am J Optom Physiol Opt. 1985;62(6):402.
31. Miller R.J., Takahama M. Arousal-related changes in dark focus accommodation and dark vergence. Invest Ophthalmol Vis Sci. 1988;29(7):1168.
32. Monkhouse W.S. The anatomy of the facial nerve. Ear Nose Throat J. 1990;69:677.
33. Ruskell G.L. An ocular parasympathetic nerve pathway of facial nerve origin and its influence on intraocular pressure. Exp Eye Res. 1970;106:323.
34. Rodriguez-Vazquez J.F., Merida-Velasco J.R., Jimenez-Collado J. Orbital muscle of Müller: observations on human fetuses measuring 35-150 mm. Acta Anat (Basel). 1990;139(4):300.
35. Ruskell G.L. Facial nerve distribution to the eye. Am J Optom Physiol Opt. 1985;62(11):793.
36. Stjernschantz J., Bill A. Vasomotor effects of facial nerve stimulation: non-cholinergic vasodilation in the eye. Acta Physiol Scand. 1980;109:45.
37. Wobig J.L. The lacrimal apparatus. In: Reeh M.J., Wobig J.L., Wirtschafter J.D., editors. Ophthalmic anatomy. San Francisco: American Academy of Ophthalmology; 1981:55.
38. Jaanus S.D., Pagano V.T., Bartlett J.D. Drugs affecting the autonomic nervous system. In: Bartlett J.D., Jaanus S.D., editors. Clinical ocular pharmacology. ed 3. Boston: Butterworth-Heinemann; 1995:168.
39. Thompson H.S. The pupil. In: Hart W.M.Jr., editor. Adler’s physiology of the eye. ed 9. St Louis: Mosby; 1992:412.
40. Newman S.A., Miller N.R. The optic tract syndrome. Neuro-ophthalmologic considerations. Arch Ophthalmol. 1983;101:1241.
41. Johnson L.N. The effect of light intensity on measurement of the relative afferent pupillary defect. Am J Ophthalmol. 1990;109(4):481.
42. Lam B.L., Thompson H.S. Brightness sense and the relative afferent pupillary defect. Am J Ophthalmol. 1989;108(4):462.
43. Sadun A.A., Bassi C.J., Lessell S. Why cataracts do not produce afferent pupillary defects. Am J Ophthalmol. 1990;110(6):712.
44. Lam B.L., Thompson H.S. A unilateral cataract produces a relative afferent pupillary defect in the contralateral eye. Ophthalmology. 1990;97(3):334.
45. Loewenfeld I.E. The Argyll Robertson pupil, 1869-1969: a critical survey of the literature. Surv Ophthalmol. 1969;14:199.
46. Thompson H.S. Light-near dissociation of the pupil. Ophthalmologica. 1984;189:21.
47. Purcell J.J., Krachmer J.H., Thompson H.S. Corneal sensation in Adie’s syndrome. Am J Ophthalmol. 1977;84:496.
48. Scheie H.G. Site of disturbance in Adie’s syndrome. Arch Ophthalmol. 1940;24:225.
49. Wirtschafter J.D., Volk C.R., Sawchuk R.J. Transaqueous diffusion of acetylcholine to denervated iris sphincter muscle: a mechanism for the tonic pupil syndrome (Adie syndrome). Ann Neurol. 1978;4:1.
50. Kawasaki A., Kardon R. Disorders of the pupil. Ophthalmol Clin North Am. 2001;14(1):149.
51. Selhorst J.B., Madge G., Ghatak N. The neuropathology of the Holmes-Adie syndrome. Ann Neurol. 1984;16:138.
52. Harriman D.G., Garland H. The pathology of Adie’s syndrome. Brain. 1968;91:401.
53. Thompson H.S. Segmental palsy of the iris sphincter in Adie’s syndrome. Arch Ophthalmol. 1978;96:1615.
54. Bourgon P., Pilley F.J., Thompson H.S. Cholinergic supersensitivity of the iris sphincter in Adie’s tonic pupil. Am J Ophthalmol. 1978;85:373.
55. Leavitt J.A., Wayman L.L., Hodge D.O., et al. Pupillary response to four concentrations of pilocarpine in normal subjects: application to testing for Adie tonic pupil. Am J Ophthalmol. 2002;133:333.
56. Wilhelm H. The pupil Curr Opin Neurol. 2008;21:36-42.
57. Kardon R.H., Denison C.E., Brown C.K., et al. Critical evaluation of the cocaine test in the diagnosis of Horner’s syndrome. Arch Ophthalmol. 1990;108(3):384.
58. Thompson H.S., Mensher J.H. Horner’s syndrome. Am J Ophthalmol. 1974;72:472.
59. Cremer S.A., Thompson H.S., Digre K.B., et al. Hydroxyamphetamine mydriasis in normal subjects. Am J Ophthalmol. 1990;110(1):66.
60. Thompson H.S., Mensher J.H. Adrenergic mydriasis in Horner’s syndrome: hydroxyamphetamine test for diagnosis of postganglionic defects. Am J Ophthalmol. 1971;72:472.
61. Salvesen R., di-Souza C.D., Sjaastad O. Horner’s syndrome: sweat gland and pupillary responsiveness in two cases with a probable 3rd neurone dysfunction. Cephalalgia. 1989;9(1):63.
62. Patel S., Ilsen P.F. Acquired Horner’s syndrome: clinical review. Optometry. 2003;74(4):245.
63. Cremer S.A., Thompson H.S., Digre K.B., et al. Hydroxyamphetamine mydriasis in Horner’s syndrome. Am J Ophthalmol. 1990;110(1):71.
64. Danesh-Meyer H.V., Savino P., Sergott R. The correlation of phenylephrine 1% with hydroxyamphetamine 1% in Horner’s syndrome. Br J Ophthalmol. 2004;88:592-593.