Autoimmunity
Learning Objectives
• Examine the relationship between thyroglobulin and iodine
• Identify the differences between T3 and T4
• Understand the hypothalamus–pituitary axis and its role in thyroid function
• Discuss the role of the thyrotropin-releasing hormone (TRH)
• Explain the function of the thyroid-stimulating hormone (TSH)
• Identify the target of the autoantibodies found in Graves’ disease
• Discuss the role(s) of cytokines and fibroblasts in Graves’ ophthalmopathy
• Define the term thyroid storm
• Design a therapy regimen to treat Graves’ disease
• Compare and contrast the symptoms of Graves’ disease and Hashimoto’s thyroiditis
• Discuss the immunologic mechanisms that contribute to Hashimoto’s thyroiditis
• Discuss the role of the intrinsic factor in vitamin B12 transport
• Identify the target of autoantibodies found in pernicious anemia
• Explain the role of vitamin B12 in red blood cell synthesis
• Design a therapy regimen to treat pernicious anemia
• Recognize the target of autoantibodies found in myasthenia gravis
• Identify the two mechanisms by which acetylcholine receptors are reduced in myasthenia gravis
• Compare and contrast ptosis and diplopia
• Design a therapy regimen to treat mild and severe myasthenia gravis
• Identify the clinical symptoms of systemic lupus erythematosus (SLE)
• List the viruses that may play a role in SLE
• Describe the two immunologic defects associated with SLE
• Design a therapy regimen to treat SLE
• Compare and contrast SLE and the “lupus-like” syndrome
• Recognize the autoantibody targets in Goodpasture syndrome
• Describe the genetic and immunologic factors that increase the risk of Goodpasture syndrome
• Design a therapy regimen to treat Goodpasture syndrome
• Differentiate between the function of pancreatic alpha, beta, and delta cells
• Relate the role(s) of insulin in the body
• List the targets of autoantibodies found in type 1a diabetes
• Identify the human leukocyte antigen (HLA) alleles that increase the risk of diabetes
• List the viruses that are implicated in the onset of diabetes
• Design a drug regimen to treat diabetes
• Define ankylosing spondylitis (AS)
• List the possible microbial triggers for AS
• Explain the role of HLA-B27 in the pathophysiology of AS
• Design a treatment regimen for AS
• Compare and contrast rheumatoid arthritis and reactive arthritis
• List the microbial agents that play a role in reactive arthritis
• Define rheumatoid arthritis (RA)
• Explain the relationship between HLA alleles and RA
• Identify the six viruses that may act as triggers for RA
• Compare and contrast the roles of macrophages and fibroblasts in the pathophysiology of RA
• Define rheumatoid factor (RF)
• Understand the relationship between RF and agalactosyl antibodies
• Design a treatment regimen to treat RA
• Discuss the role of the immune system in rheumatic heart disease
• Recognize the definition of autoimmune hemolytic anemia
• Contrast the mechanisms by which α-methyldopa, penicillin, and cephalosporin cause anemia
Key Terms
Acetylcholine
Acetylcholinesterase
Ankylosing spondylitis
Arthritis
Diabetes
Goiter
Goodpasture syndrome
Graves’ disease
Hashimoto’s thyroiditis
Intrinsic factor
Molecular mimicry
Myasthenia gravis
Pernicious anemia
RANKL (receptor activator of nuclear factor kappa-β ligand)
Reactive arthritis
Rheumatic fever
Rheumatoid factor
Systemic lupus erythematosus
Thyrotropin-releasing hormone
Thyroid-stimulating hormone
Introduction
Autoimmune diseases arise when an individual’s immune system attacks his or her own tissue and organs. The immune response may be mediated by antibodies or activated lymphocytes and macrophages. These diseases are found in 5% to 7% of the population, and the disease incidence is heavily skewed toward females. In women over 65 years of age, autoimmune diseases are one of the 10 leading causes of death.
The etiology of autoimmune disease in women is multifactorial. The factors include genetic, environmental, and hormonal triggers. Individuals expressing certain human leukocyte antigen (HLA) alleles are at high risk of developing autoimmune disease. For example, individuals expressing HLA B8 and DR3 have an increased risk of developing Graves’ disease. Because the onset of autoimmune diseases occurs shortly after puberty and just before the onset of menopause, estrogen particularly is thought to be one of the triggers for autoimmune disease. A wide range of different autoimmune diseases may affect a single organ (e.g., thyroid) or multiple organs (e.g., in systemic lupus erythematosus).
Autoimmune Diseases of the Thyroid
The function of the thyroid is to produce the hormones T3 and T4, which control physiologic functions in the cardiac, pulmonary, hematopoietic, gastrointestinal, skeletal, and endocrine systems. To produce the hormones, thyroglobulin (Tg) is synthesized by thyroid cells (thyrocytes) and stored in the follicular lumen. Iodine covalently binds to thyroglobulin tyrosine residues by using thyroperoxidase. Intracellular proteases digest thyroglobulin to produce functional triiodothyronine (T3) and thyroxin (T4), which differ only in the number of bound iodine molecules.
Synthesis of T3 and T4 is controlled by the hypothalamus–pituitary–thyroid axis. When the T3 and T4 levels fall below acceptable levels, the hypothalamus produces thyrotropin-releasing hormone (TRH) which travels through blood to the anterior pituitary gland. The anterior pituitary gland releases another hormone called thyroid-stimulating hormone (TSH). This hormone interacts with thyrotropin receptors and stimulates iodine uptake and the production of T3 and T4 (Figure 16-1).
Thymic hormone levels are controlled by negative feedback loops. High levels of TSH act on the hypothalamus to downregulate the production of TRH. T3 and T4 also act on both the pituitary and the hypothalamus to downregulate hormone production. Autoantibodies directed at the thyroid results in either hypothyroidism or hyperthyroidism.
Graves’ Disease
Graves’ disease was first reported by Dr. Robert Graves in the 1830s and is the most common cause of hyperthyroidism in children and young women. The disease is caused by IgG1 antibodies reacting with thyrotropin or TSH receptors. What causes the immune system to recognize thyroid antigens is unclear. It is conceivable that a viral infection may expose a hidden epitope in thyroid receptors.
Constant stimulation of TSH receptors causes glandular hyperplasia (goiter) and accelerated synthesis of T3 and T4. Patients often exhibit a rapid heart rate, exophthalmos, tremors, sweating, and attention deficit disorders. TSH receptors are also found in orbital cells and muscles. Reaction between the autoantibody and the TSH orbital receptor causes an inflammatory reaction called Graves’ ophthalmopathy (Figure 16-2).
Graves’ Ophthalmopathy
In the pathogenesis of Graves’ ophthalmopathy, lymphocytes infiltrate the orbit of the eye and release cytokines such as tumor necrosis factor (TNF) and interleukin 1 (IL-1). These cytokines stimulate fibroblasts to secrete a glycosaminoglycan (GAG) mucopolysaccharide. GAG increases osmotic pressure in the eye, causing edema in the extraocular and retro-orbital muscles and in adipose tissue. These changes force the eyeball forward, creating bulging of the eye, or exophthalmos.
Thyroid Storm
In patients with undiagnosed Graves’ disease, thyroid hormones often increase dramatically, which precipitates a life-threatening clinical crisis. A thyroid storm is precipitated by excessive stress, surgery, or sepsis. High levels of thymic hormones cause extreme tachycardia and atrial fibrillation. The mortality rate is 20%, and death occurs within 48 hours.
Treatment for Graves’ Disease
Thiourea was the drug initially used to treat Graves’ disease; however, it had considerable toxicity. Second-generation drugs and derivatives such as propylthiouracil and methylthiouracil, methimazole, and carbimazole have less toxicity. With the exception of methimazole, which prevents the storage of thyroid hormones, these drugs inhibit thyroperoxidase, thereby reducing the synthesis of T4 and T3.
Oral administration of radiolabeled iodine-131 is now the most common therapy for Graves’ disease. The high-intensity radiation destroys thyroid cells and brings the levels of thyroid hormones into the normal range. Subtotal thyroidectomy was the treatment of choice before the advent of radioactive iodine. Methimazole therapy is indicated for those patients in whom radioactivity is contraindicated.
Hashimoto’s Thyroiditis
Hashimoto’s thyroiditis is the most common form of hypothyroidism, with a prevalence rate of 2% of the population of the United States. Individuals mount both an antibody response and a cellular response to thyroid tissue. The disease is caused by a break in peripheral tolerance to thyroid tissue, and the failure of regulatory cells to control auto-reactive T and B cells. About 85% to 90% of patients with Hashimoto’s thyroiditis develop anti-thyroid peroxidase and anti-thyroglobulin antibodies. The inactivation of thyroperoxidase prevents the incorporation of free iodine into thyroglobulin. Elevated intracellular iodine also stimulates the maturation of dendritic cells and macrophages, which present antigens to auto-reactive CD4 and CD8 cells that have infiltrated the thyroid (Figure 16-3).
CD4Th1 cells produce IL-12, tumor necrosis factor alpha (TNF-α) and interferon gamma (INF-γ), which induce apoptosis of thyrocytes. Individuals with Hashimoto’s thyroiditis also express a molecule called Fas on thyroid cells. The expression of the Fas molecules marks the cell for destruction by T lymphocytes.
Patients with Hashimoto’s thyroiditis present with nonspecific symptoms such as fatigue, dry skin, and weight gain. Other symptoms include decreased sweating, deafness, peripheral neuropathy, depression, and memory loss.
Treatment for Hashimoto’s Thyroiditis
Oral sodium levothyroxine, which is a synthetic thyroid hormone (T4), usually has to be taken for life by patients with Hashimoto’s thyroiditis. However, the dose must be carefully titrated on the basis of the patient’s needs. Some patients cannot tolerate the synthetic hormone. Natural porcine gland preparations of T3 and T4 can be used in these patients.
Pernicious Anemia
In pernicious anemia (PA), antibodies are directed at a glycoprotein known as intrinsic factor. These autoantibodies also neutralize any soluble intrinsic factor in the intestinal lumen.
Neutralization of the soluble intrinsic factor prevents the transport of vitamin B12 across the intestinal mucosa to portal blood (Figure 16-4). Without this essential vitamin, red blood cell precursors cannot divide. This results in a megaloblastic anemia characterized by the presence of immature, dysfunctional red blood cells (megaloblasts) in the blood. If untreated, PA is usually fatal after 1 to 3 years (Figure 16-5).
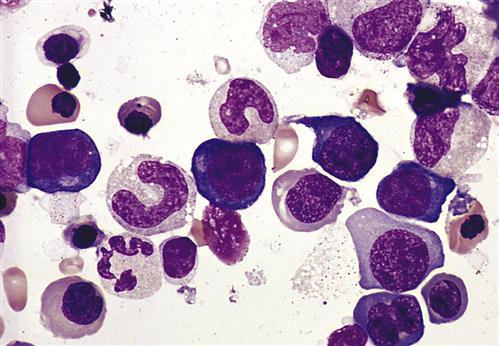