Chapter 6 Aqueous and Vitreous Humors
Anterior Chamber
The anterior chamber is bounded anteriorly by the corneal endothelium; peripherally by the trabecular meshwork, a portion of the ciliary body, and the iris root; and posteriorly by the anterior iris surface and the pupillary area of the anterior lens (Figure 6-1). The center of the anterior chamber is deeper than the periphery. The anterior chamber angle is formed at the periphery of the chamber, where the corneoscleral and uveal coats meet. The aqueous humor exits the anterior chamber through the structures located in this angle.
Anterior Chamber Angle Structures
Scleral Spur
The scleral spur lies at the posterior edge of the internal scleral sulcus (see Chapter 2). The posterior portion of the scleral spur is the attachment site for the tendon of the longitudinal ciliary muscle fibers, whereas many of the trabecular meshwork sheets attach to the spur’s anterior aspect, such that the collagen of the spur is continuous with that of the trabeculae1 (Figure 6-2).
Trabecular Meshwork
The trabecular meshwork encircles the circumference of the anterior chamber, occupying most of the inner aspect of the internal scleral sulcus. In cross section it has a triangular shape, with its apex at the termination of Descemet’s membrane (Schwalbe’s line) and its base at the scleral spur (Figure 6-3). The inner face borders the anterior chamber, and the outer side lies against corneal stroma, sclera, and Schlemm’s canal. The meshwork is composed of flattened perforated sheets, with three to five sheets at the apex. These sheets branch into 15 to 20 sheets as they extend posteriorly from Schwalbe’s line to the scleral spur.2 The trabecular meshwork is an open latticework, the branches of which interlace. The intertrabecular spaces between the sheets are connected through pores, or openings within the sheets (historically called the “spaces of Fontana”).3 The openings are of varying sizes and become smaller near Schlemm’s canal. No apertures directly join the meshwork with the canal.2 A small aspect of the meshwork at the most anterior location is adjacent to connective tissue of the limbus and differs in structure from the filtering portion. Some believe that this is a niche where cells reside that have properties similar to stem cells. These cells may be capable of replacing the endothelial cells of the trabecular meshwork after injury.4
The meshwork can be separated into two anatomic divisions. The corneoscleral meshwork is the outer region; its sheets attach to the scleral spur. The inner sheets, which lie inner to the spur and attach to the ciliary stroma and longitudinal muscle fibers, make up the uveal meshwork; some of these sheets may attach to the iris root.3,5 The two portions differ slightly in structure; the corneoscleral meshwork is sheetlike, and the uveal meshwork is cordlike2 (Figure 6-4). The pores in the uveal meshwork are the largest, and pore size diminishes in the sheets closer to the canal. Projections from the surface layer of the iris, known as iris processes, connect to the trabeculae, usually projecting no farther forward than the midpoint of the meshwork.2
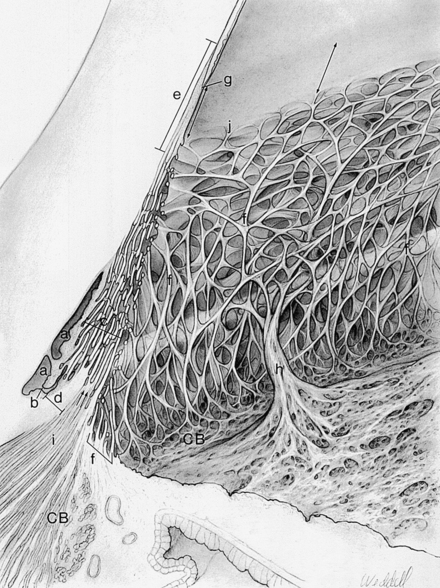
FIGURE 6-4 Drawing of aqueous outflow apparatus and adjacent tissues.
(From Hogan MJ, Alvarado JA, Weddell JE: Histology of the human eye, Philadelphia, 1971, Saunders.)
The meshwork trabeculae consist of an inner core of collagen and elastic fibers6 embedded in ground substance and covered by basement membrane and endothelium.7 The endothelial cells are a continuation of the corneal endothelium.5,8 The endothelial cells contain the cellular organelles for protein synthesis and apparently are capable of replacing the connective tissue components. These cells also contain lysosomes, which give them the capacity for phagocytosis.5 Gap junctions and short areas of tight junctions join the endothelial cells; no zonula occludens are found.9 Cytoplasmic projections connect cells of neighboring sheets.5,9,10
At the scleral spur, the trabecular sheets lose their endothelial covering, but the collagenous and elastic fibers continue into the connective tissue of the spur and ciliary body.2,5 Some connective tissue fibers of the ciliary muscle pass forward and merge with the inner sheets of the meshwork.11
Canal of Schlemm
The canal of Schlemm is a circular vessel and is considered to be a venous channel, although it normally contains aqueous humor rather than blood. It is outer to the trabecular meshwork and anterior to the scleral spur. The external wall of the canal lies against the limbal sclera, and the internal wall lies against the juxtacanalicular connective tissue and the scleral spur (see Figure 6-2). Thin tissue septa may bridge the lumen, dividing it into several channels.2,3
The lumen is lined with endothelial cells, many of which are joined by zonula occludens.2,9,12 The endothelial cells have an incomplete basement membrane.2,3,7 The continuous endothelial lining with cells joined by tight junctions make the canal similar to blood vessels, whereas the discontinuous basement membrane make it similar to lymph channels.13 The tight junctions of the inner wall restrict flow into the canal between the lateral walls of the cells. Pores and pinocytic vesicles in the cell membrane may be an avenue for passage of aqueous humor.2,3,14–16
Juxtacanalicular Connective Tissue
The region separating the endothelial cell lining of the canal from the trabecular meshwork is called the juxtacanalicular tissue5,11 or the cribriform layer.5,15,17 It consists of endothelial cells and fibroblasts embedded in a matrix of collagen, elastic-like fibers, and ground substance.15,18–20 The cells of this region have processes occasionally joined by adhering and gap junctions. The cells also form similar connections with the endothelium of the inner wall of Schlemm’s canal.21 There are micron-sized spaces within the juxtacanalicular tissue that appear to lack extracellular matrix (although these may be presumed spaces whose material is yet to be observed)22 and may provide a tortuous pathway for fluid to move toward the inner wall of the canal. The endothelium of Schlemm’s canal is anchored to the juxtacanalicular tissue by a network of elastic-containing fibrils that is also connected to the scleral spur and the tendon of the ciliary muscle.19 This connective network might help in modulating aqueous outflow.21
Clinical Comment: Gonioscopy
If the angle does not appear to be wide enough or if there is concern that aqueous exit is inadequate, a view of the chamber angle structures might be necessary. A direct view of the angle cannot be achieved because the limbus is opaque, and light directed obliquely through the cornea into the angle does not exit because of total internal reflection. Therefore a clinical procedure, gonioscopy, is performed that uses a special lens, a goniolens (Figure 6-5). A goniolens can overcome total internal reflection and contains mirrors in which the examiner views the angle. The image the examiner sees is as if he or she is facing the angle and sighting along the anterior surface of the iris. If all structures can be seen, they appear in the following order, beginning at the inferior aspect: iris root, ciliary body, scleral spur, trabecular meshwork, and Schwalbe’s line (Figure 6-6). (The iris root may or may not be visible.) Schlemm’s canal lies behind the trabecular meshwork in this view and appears as a thin red line within the meshwork if blood is backed up in the canal. Such pooling of blood occurs if the examiner exerts pressure on the goniolens, thereby compressing the episcleral veins and causing the episcleral venous pressure to exceed intraocular pressure.23
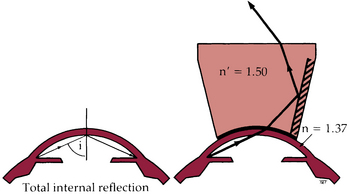
FIGURE 6-5 Optical principles of gonioscopy: n and n’, refractive index.
(From Kanski JJ: Clinical ophthalmology: a systematic approach, ed 5, Oxford, UK, 2003, Butterworth-Heinemann.)
Function of the Filtration Apparatus
The primary function of Schlemm’s canal and the trabecular meshwork is to provide an exit for the aqueous humor. In addition, with the movement of aqueous through these structures, nutrients can diffuse into surrounding tissue, thereby supplying nutrients to the nearby deep limbal and scleral tissue.8
Posterior Chamber
The posterior chamber is an annular area located behind the iris and bounded by the posterior iris surface, the equatorial zone of the lens, the anterior face of the vitreous, and the ciliary body. The ciliary processes that secrete the aqueous humor project into the posterior chamber. The zonule fibers arise from the internal limiting membrane of the nonpigmented epithelium of the ciliary body, pass through the posterior chamber, and insert into the lens capsule.1 The posterior chamber contains two regions: The area occupied by the zonules is the canal of Hannover, and the retrozonular space, the area from the most posterior zonules to the vitreal face, is the canal of Petit2 (Figure 6-7). The canal of Petit might be better described as a potential space.
Aqueous Dynamics
The aqueous humor provides necessary metabolites, primarily oxygen and glucose, to the avascular cornea and lens. It is produced in the pars plicata of the ciliary body and is secreted into the posterior chamber through the epithelium covering the ciliary processes. It passes between the iris and lens, entering the anterior chamber through the pupil (Figure 6-8). In the anterior chamber, the aqueous circulates in convection currents, moving down along the cooler cornea and up along the warmer iris and exiting through the periphery of the chamber.
There are two avenues by which aqueous exits the anterior chamber. It can pass through the spaces within the uveal meshwork; this pathway is called the unconventional outflow and accounts for a relatively small amount (variously reported in the literature as 5% to 35% of the total outflow24–26). The fluid then passes into the connective tissue spaces surrounding the ciliary muscle bundles,27 moves into the suprachoroidal space, and is absorbed into and through the sclera.28 Alternately, the fluid is absorbed into the anterior ciliary veins and vortex veins. Additional veins recently identified may represent suprachoroidal collector channels designed to accommodate this uveoscleral flow.24 There is some evidence that lymphatic channels exist in the ciliary stroma, which might provide an additional avenue for aqueous exit.29
The remainder of the aqueous follows the conventional outflow pathway and moves through the meshwork and into the narrower pores of the corneoscleral meshwork and through the juxtacanalicular tissue and the endothelial lining into Schlemm’s canal.18 In histologic sections, many of the endothelial cells lining the inner wall of the canal have been found to contain giant vacuoles,7,12,16,30–32 some of which exhibit openings into the lumen.10,33,34 The vacuoles apparently open and close intermittently, creating transient, transcellular, unidirectional channels that provide a means for transporting large molecules, such as proteins, across the endothelium. Flow occurs only into the canal.14,15,35 An indentation forms in the basal surface of the endothelial cell, gradually enlarges, and eventually opens onto the apical surface (Figure 6-9). Then the cytoplasm in the basal aspect of the cell moves to occlude the opening.35 The number of pores throughout the endothelium is uncertain because some may be artifacts caused by tissue preparation.36 Smaller pinocytic vesicles also provide a transport system for substances. However, the greatest volume of aqueous humor diffuses passively into Schlemm’s canal. Recent speculation suggests that the tight intercellular junctions may respond to changing physiologic conditions (i.e., effects of pharmacologic agents) by modifying their permeability and increasing the ease by which aqueous flows into the canal.9,12 The endothelial cells of the trabecular meshwork may actually release cellular factors that can increase the permeability of the inner wall of Schlemm’s canal.37
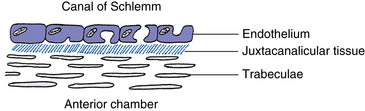
FIGURE 6-9 Formation of giant vacuoles in endothelial cells lining Schlemm’s canal.
(Modified from Bartlett JD, Jaanus SD: Clinical ocular pharmacology, ed 2, Boston, 1989, Butterworth-Heinemann.)
The internal wall of Schlemm’s canal contains a number of evaginations, or blind pouches, that extend into the juxtacanalicular tissue toward the trabecular meshwork. These internal collector channels (of Sondermann)38 can be fairly long and branching, and serve to increase the surface area of the canal2 (Figure 6-10). Their endothelium is always separated from the trabecular space by a sheet of connective tissue.
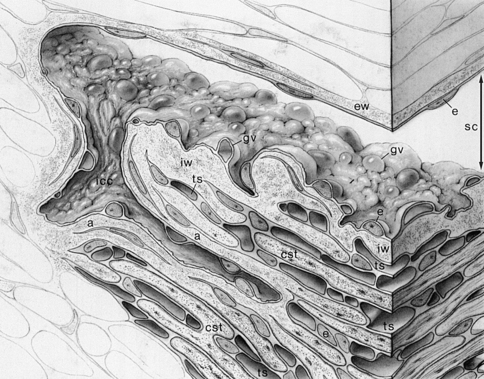
FIGURE 6-10 Drawing of Schlemm’s canal, an internal collector channel, and adjacent tissues.
(From Hogan MJ, Alvarado JA, Weddell JE: Histology of the human eye, Philadelphia, 1971, Saunders.)
The endothelial cells lining the external wall of Schlemm’s canal are joined by zonula occludens and contain no vacuoles. Approximately 25 to 35 external collector channels are distributed around the outer wall of Schlemm’s canal and branch from it to empty into either the deep scleral plexus or the intrascleral plexus of veins,5,39,40 which in turn drain into the episcleral and conjunctival veins.2 Aqueous veins (of Ascher), that pass from the outer wall of the canal directly to the episcleral veins, provide another route from the canal.41
Factors Affecting Intraocular Pressure
The outflow can be impeded at various sites along the pathway. Aqueous that exits through the ciliary body (the unconventional outflow) passes into the ciliary body from the anterior chamber either through the uveoscleral meshwork or directly into the ciliary body. There is no continuous layer of epithelium covering the ciliary body as it borders the anterior chamber and thus the tissue offers little resistance to aqueous passage. The uveoscleral outflow is believed to be a fairly constant amount not affected by IOP.26,42 Figure 6-11 shows the affect that IOP has on flow through the two outflow pathways.
There is greater variability in the amount exiting via the conventional pathway. Resistance in this outflow pathway is a major factor in the rate of aqueous exit and influences intraocular pressure. There is normally little resistance to aqueous passage through the sheets of the trabecular meshwork unless pigment or debris has accumulated within the pores. When Schlemm’s canal is wide open it also provides little to no resistance to outflow; likewise the external collector channels and aqueous veins normally provide negligible resistance.22 The location of the highest resistance to aqueous movement seems to be in the region of the juxtacanalicular tissue (JCT) and (according to some) the endothelium of the inner wall of Schlemm’s canal.13,21,22,43 Plaquelike material that accumulates in the extracellular matrix of the juxtacanalicular area either directly or indirectly increases resistance in the outflow pathway.13 In the normal eye, the cells of the trabecular meshwork and the cells within the JCT are speculated to have some self-regulating ability that can influence changes in resistance and thus IOP.21,44,45 Sustained resistance to outflow usually results in elevated IOP.
Because of the elastic-like network of fibers joining the scleral spur, the ciliary muscle, and the meshwork, ciliary muscle contraction can alter the geometry of the trabeculum by widening the spaces between the sheets, resulting in a decrease in outflow resistance.11,43 Elastic fibers of the spur are continuous with elastic fibers in the JCT, and fibers from the JCT connect with the endothelium of Schlemm’s canal. Interaction among these fibers during accommodation can increase the lumen diameter.21
It is important that the amount of aqueous formed (flow in) is equal to the amount that exits the eye (flow out) and can be represented as Fin = Fout. This represents a balance between the factors affecting production and those involved in exit. As discussed in Chapter 3, aqueous production (Fin) is dependent on molecules moving out of the ciliary body capillaries and through the ciliary stroma and epithelium. Movement out of the blood vessels occurs because the pressure within the capillaries is greater than the pressure within the eye (IOP), and can be represented as (PCB caps − IOP).42 The ease with which the molecules pass through tissue is called facility and will be represented as (Cin). (Facility is the reciprocal of resistance, as resistance increases, facility decreases.) The final factor in aqueous production is the rate at which energy-utilizing pumps actively move material toward secretion into the posterior chamber and is designated as (S). Thus Fin = (PCB caps − IOP) Cin + S.42 S is generally considered to be a constant; the other factors might fluctuate.
Flow out (Fout) includes both conventional and unconventional outflow. Flow from Schlemm’s canal and into the episcleral veins is represented as (IOP − Pev)42 and the ease with which the aqueous moves through the trabecular meshwork and into Schlemm’s canal is the facility, represented by (Cout). The small amount that exits through the uveoscleral meshwork is represented by (U) and is generally fairly constant.
Although this equation is an over-simplification, it does give an indication of the factors to be considered and their interdependence. It represents the steady state of homeostasis, and in the normal eye, only small fluctuations occur throughout the day.46 The amount of aqueous produced usually does not change appreciably and so when the steady state is disrupted, it is usually the flow out that is compromised and an elevation of IOP can follow. If aqueous exit is compromised, it is usually because of an in increase in resistance to aqueous exit (thus a decrease in facility); the major location of the increased resistance is likely to be the juxtacanalicular tissue between the last trabecular sheet and the inner wall of Schlemm’s canal.
Small fluctuations in IOP might have some small effect on the egress of aqueous from the ciliary processes, but this is usually negligible.
Clinical Comment: Glaucoma
Deposits of pigment or debris on the trabecular sheets and cords can restrict aqueous flow through the trabecular spaces. Proliferation of the juxtacanalicular tissue increases with age and has been found to cause a decrease in outflow.5 Some histologic preparations of ocular tissue from glaucomatous eyes give evidence for a decrease in outflow. A reduction in the cross-sectional diameter of Schlemm’s canal and fewer pores in the endothelial lining of the canal were found in glaucomatous eyes when compared with normal eyes.47,48 Other studies show an increase in the fibrillar component of the matrix in the juxtacanalicular tissue in glaucomatous eyes.43
Drugs that Effect IOP
Glaucoma treatment consists of attempts to reduce pressure using drugs that either decrease aqueous production or increase aqueous outflow. One of the earliest treatment plans prescribed a cholinergic agonist that caused the iris sphincter and ciliary muscle to contract, thus changing the configuration of the trabecular sheets to facilitate outflow, perhaps by allowing more separation between the sheets.11,49,50 Pilocarpine was commonly used; however, compliance was often poor because of the uncomfortable side effects—miosis and ciliary spasm.
Drugs that inhibit production act on the ciliary epithelia, either by interfering with neural pathways or by inhibiting intracellular enzymes that maintain the ionic transport mechanisms important in the formation of aqueous.51 Although the role of sympathetic innervation in aqueous production is unclear, beta-blockers and alpha-adrenergic agonists do decrease aqueous production, perhaps by interfering with ciliary epithelial function. There is some speculation that drugs that have vasoconstrictive action can decrease aqueous production by decreasing blood flow in the ciliary vessels, causing a reduction in oxygen availability to the tissue.51 During the 1970s and 1980s, beta-blockers were the preferred method of treatment.52
Carbonic anhydrase inhibitors are also common in glaucoma treatment but are not well tolerated in many patients. They inhibit key enzymes necessary for ionic transport across the epithelial layers. Currently the most effective drugs used in glaucoma treatment are prostaglandins; they are well tolerated and compliance is good because instillation may only be necessary once a day. Prostaglandins enhance outflow through the uveoscleral pathway, although the exact mechanism has not been fully defined. They are found to relax ciliary muscle tone and to remodel the extracellular matrix within the connective tissue between muscle bundles, increasing the spacing between bundles and increasing tissue permeability for increased aqueous flow.25,26,45,53–55 Certain prostaglandin analogues may also increase trabecular meshwork outflow.56
Aging Changes in the Anterior Chamber
With age, the anterior chamber angle width narrows and the anterior chamber volume decreases, probably secondary to lens growth. This narrowing is more significant in women and may be related to the higher incidence of angle-closure glaucoma in elderly women.57
Other age-related changes include a decrease in aqueous production, a reduction in uveoscleral outflow reduction, a decrease in the density and size of giant vacuoles, an accumulation of extracellular matrix plaques in the juxtacanalicular tissue, and an increase in the outflow resistance in the vicinity of the juxtacanalicular tissue and the inner wall of Schlemm’s canal.22,58,59 An increase in the amount of connective tissue in the ciliary muscle may be the cause of the reduction in uveoscleral outflow.26 However, no age-related increase in IOP is seen in healthy individuals with pressures below 22 mm Hg.58
Vitreous Chamber
Vitreal Attachments
The vitreous base, the most extensive adhesion, extends 1.5 to 2 mm anterior to the ora serrata, 1 to 3 mm posterior to it, and several millimeters into the vitreous2,60 (Figure 6-12). The vitreal fibers that form the base are embedded firmly in the basement membrane of the nonpigmented epithelium of the ciliary body and the internal limiting membrane of the peripheral retina.39,61
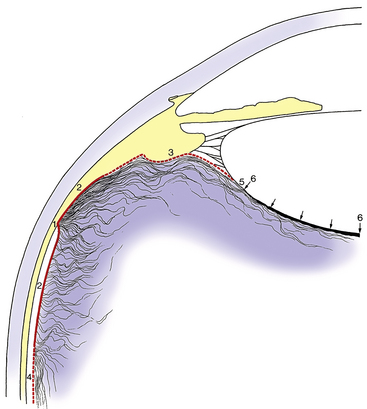
FIGURE 6-12 Vitreous relationships in the anterior eye.
(From Hogan MJ, Alvarado JA, Weddell JE: Histology of the human eye, Philadelphia, 1971, Saunders.)
The hyaloideocapsular ligament (of Weiger), or retrolental ligament, forms an annular attachment 1 to 2 mm wide and 8 to 9 mm in diameter between the posterior surface of the lens and the anterior face of the vitreous.60 This is a firm attachment site in young persons, but the strength of the bond diminishes after age 35.62 Within the ring formed by this ligament is a potential space, the retrolental space (of Berger), which is present because the lens and vitreous are juxtaposed but not joined.2
The peripapillary adhesion around the edge of the optic disc also diminishes with age. The annular ring of attachment at the macula is 3 to 4 mm in diameter.2
The attachment of the vitreous to retinal blood vessels consists of fine strands that extend through the internal limiting membrane to branch and surround the larger retinal vessels.61,63 These strands may account for hemorrhages that occur when there is vitreal traction on the retina. The nature of the attachment between the vitreous and the retinal internal limiting membrane throughout the rest of the retina remains uncertain. It is unlikely that fibrils from the posterior vitreous insert into the internal limiting membrane.64–66 But rather the vitreoretinal interface contains a “molecular glue” linking the outer part of the cortex and the inner part of the limiting membrane.2,67,68 This area contains extracellular matrix—molecules, including laminin and fibronectin, that have been identified as having adhesive properties.62
Vitreous Zones
The vitreous can be divided into zones that differ in relative density. The outermost zone is the vitreous cortex, the center zone is occupied by Cloquet’s canal, and the intermediate zone is inner to the cortex and surrounds the center canal.
Vitreous Cortex
The vitreous cortex, also called the hyaloid surface, is the outer zone.69 It is 100 μm wide,2 and it is composed of tightly packed collagen fibrils, some of which run parallel and some perpendicular to the retinal surface.70,71 The anterior cortex lies anterior to the base and is adjacent to the ciliary body, posterior chamber, and lens. The posterior cortex extends posterior to the base and is in contact with the retina. It contains transvitreal channels that appear as holes—the prepapillary hole, the premacular hole, and prevascular fissures. The prepapillary hole can sometimes be seen clinically when the posterior vitreous detaches from the retina.60 The premacular hole, a weak area, may be a region of decreased density rather than an actual hole.60,71 The prevascular fissures provide the avenue by which fine fibers enter the retina and encircle retinal vessels.61
Intermediate Zone
The intermediate zone contains fine fibers that are continuous and unbranched and that run anteroposteriorly.60,70,72 These fibers arise at the region of the vitreous base and insert into the posterior cortex.73 The peripheral fibers parallel the cortex, whereas the more central fibers parallel Cloquet’s canal. Membranelike condensations, called vitreous tracts, may be differentiated as areas that have differing fiber densities (Figure 6-13).71
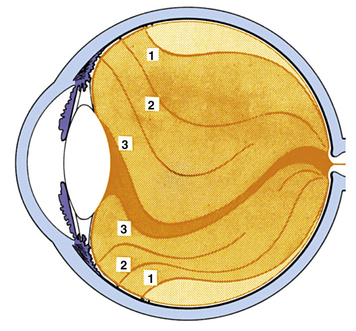
FIGURE 6-13 Eisner’s interpretation71 of vitreous structures (according to slit-lamp examinations of eyes obtained at autopsy). Vitreous body is divided into three zones: Externally, as far as retina extends, there is a relatively thick vitreous cortex (light orange). It has holes at characteristic locations: in front of papilla, in region of fovea centralis, in front of vessels, and in front of anomalies of ora serrata region (enclosed ora bays, meridional folds, zonular traction tufts). Intermediate zone (medium orange) contains vitreal tracts, membranelles that form funnels packed into one another and that diverge from region of papilla anteriorly. Central channel (dark orange) is space delimited by hyaloid tract. It is closed off anteriorly by a retrolental section of anterior vitreous membrane. It contains no typical tracts but only irregularly arranged vitreous fibers, part of which are residua of Cloquet’s canal. Outermost vitreous tract, the preretinal tract (1), separates intermediary substance from vitreous cortex. Innermost tract, the hyaloid tract (3), inserts at the edge of the lens. Between these tracts extends the median tract (2) to median ligament of pars plana and the coronary tract to coronary ligament.
(From Sebag J: The vitreous. In Hart WM Jr, editor: Adler’s physiology of the eye, ed 9, St Louis, 1992, Mosby.)
Cloquet’s Canal
Cloquet’s canal, also called the hyaloid channel or the retrolental tract, is located in the center of the vitreous body.2 It has an S shape, rotated 90 degrees with the center dip downward, and is the former site of the hyaloid artery system, which was formed during embryologic development (see Chapter 7). Cloquet’s canal arises at the retrolental space. Its anterior face is approximately 4 to 5 mm in diameter.2 It terminates at the area of Martegiani, a funnel-shaped space at the optic nerve head that extends forward into the vitreous to become continuous with the canal.2,60
Composition of Vitreous
The highly transparent vitreous is a dilute solution of salts, soluble proteins, and hyaluronic acid contained within a meshwork of the insoluble protein, collagen. Vitreous is 98.5% to 99.7% water and has been described as having connective tissue status and being an extracellular matrix.71,74 Because of its high water content, study of the vitreous is difficult. Attempts at tissue fixation often have dehydrating effects that introduce artifacts. Recent investigations suggest that the epithelium of the pars plana has a significant role in the production and secretion of several connective tissue macromolecules of the vitreous body.74
Collagen
The collagen content of the vitreous is highest in the vitreous base, next highest in the posterior cortex, next in the anterior cortex, and lowest in the center.60 A fine meshwork of uniform collagen fibrils, each 8 to 16 nm in diameter, is evident on electron microscopy and fills the vitreous body.69,75–77 The individual fibrils cannot be seen with the slit lamp, but the pattern of variations in their density and regularity can be seen. The density of this collagen fibril network differs throughout the vitreous.44
Hyaluronic Acid (hyaluronan)
The second major vitreal component, hyaluronic acid (HA), a glycosaminoglycan, is a long unbranched molecule coiled into a twisted network.72 This hydrophilic macromolecule is located in specific sites within the collagen fibril network and is believed to maintain the wide spacing between fibrils.60 The concentration of HA is highest in the posterior cortex and decreases centrally and anteriorly.68,78 The gel structure is a result of the interaction of collagen and HA. HA stabilizes the network formed by the collagen strands.
Hyalocytes
Vitreous cells, or hyalocytes, are located in a single, widely spaced layer in the cortex near the vitreal surface and parallel to it.60,72 Various functions have been attributed to these cells. Some investigators have determined that these cells synthesize HA.79–81 Others have found evidence that hyalocytes synthesize glycoproteins for the collagen fibrils.60,82 Still others indicate that hyalocytes have phagocytic properties.72,81,83 Apparently, hyalocytes can have different appearances depending on their activity at a given time.60 Cells located in the vitreous base are fibroblast-like when anterior to the ora serrata and macrophage-like when posterior to it.64
Fibroblasts present in the vitreous are located in the vitreous base near the ciliary body and near the optic disc. Although composing less than 10% of the cell population, fibroblasts may have been mistaken for hyalocytes in the past. It is believed that fibroblasts synthesize the collagen fibrils that run anteroposteriorly and are active in pathologic conditions.60
Other cells that have been identified as macrophages likely originate in the nearby retinal blood vessels.2,71
Vitreal Function
The vitreous body provides physical support holding the retina in place next to the choroid, the blood supply for the outer retina. (Neural retina and choroid are only connected to each other at the disc and the ora serrata.) The vitreous is a storage area for metabolites for the retina and lens and provides an avenue for the movement of these substances within the eye.41 The vitreous, because of its viscoelastic properties, acts as a “shock absorber,” protecting the fragile retinal tissue during rapid eye movements and strenuous physical activity.2,71 The vitreous transmits and refracts light, aiding in focusing the rays on the retina. Minimal light scattering occurs in the vitreous because of its extremely low concentration of particles and the interfibrillar spacing ensured by the HA-collagen complex.
Age-Related Vitreal Changes
In the infant the vitreous is a very homogeneous, gel-like body. With maturation, changes occur in which the gel volume decreases and the liquid volume increases; this is called vitreous liquefaction or vitreous synersis.60 By age 40 years, the vitreous is 80% gel and 20% liquid, and by 70 or 80 years it is 50% liquid,70 with most of the liquefaction occurring in the central vitreous.71 Both HA and collagen may be detrimentally affected by free radicals that cause conformational changes in the HA molecule and breakdown in collagen cross-links. Subsequent displacement of collagen from the HA-collagen network influences the change from gel to liquid.46,84,85 As the dissolution of the HA-collagen complex occurs, the macromolecule moves out of the collagen network, causing the fibrils to coalesce into fibers and then into bands.85,86 The redistribution of collagen leaves spaces adjacent to these bundles, allowing pooling of liquid vitreous; these pockets are called lacunae. 68,86,87
Clinical Comment: Peripheral Retinal Traction
With aging, the vitreous base adhesion extends further posteriorly, and the border approaches the equator.88,89 These changes can increase traction on peripheral retina and might contribute to the development of retinal tears and detachment.
Clinical Comment: Posterior Vitreal Detachment
As the HA is displaced from the collagen network and as the fibrils coalesce into bundles, the bundles can contract and apply traction to the vitreous and thus to the posterior retina. One of the most common abnormalities that occurs at the posterior retinal-vitreous interface is a posterior vitreal detachment caused by this traction. The vitreous usually detaches from the retinal internal limiting membrane at the peripapillary ring, forming a retrocortical space. If glial tissue is torn away with the vitreous, a circular condensation, Weiss’ ring (senile annular ring), may be visible within the vitreous.90 If liquid vitreous seeps into the retrocortical space through the prepapillary and premacular areas, a syneresis, or collapse, of the vitreous can follow because of the volume displacement.86,91
Physiology of the Vitreous
The vitreous was thought to merely passively interact and support surrounding tissues but a new understanding of the dynamic vitreous is developing. The cells in the cortex reamin largely quiescent because factors present in the vitreous prevent cell migration and proliferation. The interaction between HA and collagen fibrils contributes to the viscoelastic properties of the vitreous92,93 and influences the physical properties, i.e., the balance between gel and liquid, of the vitreous state.60 Most of the water in the vitreous is bound in the widely-spaced network of collagen and HA. A disruption in the HA-collagen complex can cause the collagen fibrils to aggregate into bundles, which may become large enough to be visible clinically, and reported by a patient as floaters.
While there is little metabolic activity within the vitreous and a slow degradation occurs with age, an intact vitreous gel may be quite important to ocular health; age-related degeneration of the vitreous gel and liquefaction accompanies several age-related ocular diseases, such as nuclear sclerotic cataract and neovascular diabetic retinopathy.94 Studies suggest a correlation between vitreous degeneration and the development of nuclear sclerotic cataract (NSC), inferring that an intact vitreous provides some protection against lens nuclear changes. A much higher incidence of NSC after vitrectomy was found in patients over 50 than in those younger.95,96 It may be that the younger lens is more resistant to cataractous changes or that the presence of anterior vitreous that is still adherent to posterior lens (it was not removed in the vitrectomy because of the stronger adhesion) provides some protection against NSC.
Nuclear sclerotic cataract is the result of oxidative changes within the lens nucleus. The vitreous has a high concentration of ascorbate (up to 40 times higher than blood plasma) and might have a role in the regulation of intraocular molecular oxygen.94 As oxygen diffuses into the vitreous from the retinal vessels, it is likely to be consumed by ascorbate before it reaches the lens and anterior segment, providing some protection from oxidative stress. The lens might therefore be exposed to a greater concentration of oxygen after vitrectomy. Vitreous gel has a higher concentration of ascorbate and consumes oxygen at a faster rate than liquid vitreous.97 Vitreous loss due to liquefaction or vitrectomy can be linked to disease processes in which excessive oxygen causes oxidative stress and tissue damage.
Another hypothesis suggests that some might benefit from vitreous liquefaction or surgical removal of the vitreous. Vitreous loss that results in increased intraocular molecular oxygen may benefit ischemic retinal disease by lowering vascular endothelial growth factor (VEGF) and thus reduce neovascularization.94 The importance of the vitreous and a better understanding of its relationship with neighboring tissues will become more evident as studies continue.
1. Fine B.S., Yanoff M. The vitreous body. In: Fine B.S., Yanoff M., editors. Ocular histology. ed 2. Hagerstown, Md: Harper & Row; 1979:131.
2. Hogan M.J., Alvarado J.A., Weddell J.E. Histology of the human eye. Philadelphia: Saunders; 1971.
3. Warwick R. Eugene Wolff’s anatomy of the eye and orbit, ed 7. Philadelphia: Saunders; 1976.
4. Kelley M.J., Rose A.Y., Keller K.E., et al. Stem cells in the trabecular meshwork: present and future promises. Exp Eye Res. 2009;88:747-751.
5. Lütjen-Drecoll E., Rohen J.W. Functional morphology of the trabecular meshwork. Tasman W., Jaeger E.A., editors. Duane’s foundations of clinical ophthalmology, vol 1. Philadelphia: Lippincott; 1994:1.
6. Alexander R.A., Garner A. Elastic and precursor fibres in the normal human eye. Exp Eye Res. 1983;36:305.
7 Gong H., Ruberti J., Overby D., et al. A new view of the human trabecular meshwork using quick-freeze, deep-etch electron microscopy. Exp Eye Res. 2002;75:347.
8. Fine B.S. Structure of the trabecular meshwork and the canal of Schlemm. Trans Am Acad Ophthalmol Otolaryngol. 1966;70:777.
9. Bhatt K., Gong F., Freddo T.F. Freeze-fracture studies of interendothelial junctions in the angle of the human eye. Invest Ophthalmol Vis Sci. 1995;36(7):1379.
10. Raviola G., Raviola E. Paracellular route of aqueous in the trabecular meshwork and canal of Schlemm. A freeze-fracture study of the endothelial junctions in the sclerocorneal angle of the macaque monkey eye. Invest Ophthalmol Vis Sci. 1981;21:52.
11. Kaufman P.L. Enhancing trabecular outflow by disrupting the actin cytoskeleton, increasing uveoscleral outflow with prostaglandins, and understanding the pathophysiology of presbyopia interrogating Mother Nature: asking why, asking how, recognizing the signs, following the trail. Exp Eye Res. 2008;86:3-17.
12. Ye W., Gong H., Sit A., et al. Interendothelial junctions in normal human Schlemm’s canal respond to changes in pressure. Invest Ophthalmol Vis Sci. 1997;38(12):2460.
13. Johnson M. What controls aqueous humour outflow resistance? Exp Eye Res. 2006;82:545-557.
14. Bill A. Scanning electron microscopic studies of the canal of Schlemm. Exp Eye Res. 1970;10:214.
15. Epstein D.L., Rohen J.W. Morphology of the trabecular meshwork and inner-wall endothelium after cationized ferritin perfusion in the monkey eye. Invest Ophthalmol Vis Sci. 1991;32:160.
16. Ethier C.R., Coloma F.M., Sit A.J., et al. Two pore types in the inner-wall endothelium of Schlemm’s canal. Invest Ophthalmol Vis Sci. 1998;39(11):2041.
17. Lütjen-Drecoll E., Futa R., Rohen J.W. Ultrahistochemical studies on tangential sections of the trabecular meshwork in normal and glaucomatous eyes. Invest Ophthalmol Vis Sci. 1981;21:563.
18. Inomata H., Bill A., Smelser G.K. Aqueous humor pathways through the trabecular meshwork and into Schlemm’s canal in the cynomolgus monkey (Macaca irus). Am J Ophthalmol. 1972;73:760.
19. Rohen J.W., Futa R., Lütjen-Drecoll E. The fine structure of the cribriform network in normal and glaucomatous eyes as seen in tangential sections. Invest Ophthalmol Vis Sci. 1981;21:574.
20. Umihira J., Nagata S., Nohara M., et al. Localization of elastin in the normal and glaucomatous human trabecular meshwork. Invest Ophthalmol Vis Sci. 1994;35(2):486.
21. Acott T.S., Kelley M.J. Extracellular matrix in the trabecular meshwork. Exp Eye Res. 2008;86:543-561.
22. Overby D.R., Stamer W.D., Johnson M. The changing paradigm of outflow resistance generation: towards synergistic models of the JCT and inner wall endothelium. Exp Eye Res. 2009;88:656-670.
23. Kanski J.J. Clinical ophthalmology, ed 3. Oxford, UK: Butterworth-Heinemann; 1994.
24. Krohn J., Bertelsen T. Corrosion casts of the suprachoroidal space and uveoscleral drainage routes in the human eye. Acta Ophthalmol Scand. 1997;75:32.
25. Nilsson S.F. The uveoscleral outflow routes. Eye. 1997;11:149.
26. Alm A., Nilsson S.F. Uveoscleral outflow—a review. Exp Eye Res. 2009;88:760-768.
27. Bill A. Some aspects of aqueous humour drainage. Eye. 1993;7:14.
28. Krohn J., Bertelsen T. Light microscopy of uveoscleral drainage routes after gelatine injections into the suprachoroidal space. Acta Ophthalmol Scand. 1998;76(5):521. (abstract)
29. Gupta N., Patel M., Ly T., et al. Evidence of a new uveolymphatic outflow pathway in human and sheep; implications for aqueous drainage and glaucoma. Invest Ophthalmol Vis Sci. 49, 2008.
30. Holmberg A.S. The fine structure of the inner wall of Schlemm’s canal. Arch Ophthalmol. 1959;62:956.
31. Speakman J.S. Drainage channels in the trabecular wall of Schlemm’s canal. Br J Ophthalmol. 1960;44:513.
32. Parc C.E., Johnson D.H., Brilakis H.S. Giant vacuoles are found preferentially near collector channels. Invest Ophthalmol Vis Sci. 2000;41:2984.
33. Feeney .L. Outflow studies using an electron dense tracer. Trans Am Acad Ophthalmol Otolaryngol. 1966;70:791.
34. Anderson D.R. Scanning electron microscopy of primate trabecular meshwork. Am J Ophthalmol. 1971;71:90.
35. Tripathi E.R.C. Mechanism of the aqueous outflow across the trabecular wall of Schlemm’s canal. Exp Eye Res. 1971;11:116.
36. Sit A.J., Coloma F.M., Ethier C.R., et al. Factors affecting the pores of the inner wall endothelium of Schlemm’s canal. Invest Ophthalmol Vis Sci. 1997;38(8):1517.
37. Alvarado J.A., Alvarado R.G., Yeh R.F., et al. A new insight into the cellular regulation of aqueous outflow: how trabecular meshwork endothelial cells drive a mechanism that regulates the permeability of Schlemm’s canal endothelial cells. Br J Ophthalmol. 2005;89:1500-1505.
38. Sondermann R. The formation, morphology and function of Schlemm’s canal. Acta Ophthalmol. 1933;11:280.
39. Dvorak-Theobold G. Schlemm’s canal: its anastomosis and anatomic relations. Trans Am Ophthalmol Soc. 1934;32:574.
40. Ashton N. Anatomical study of Schlemm’s canal and aqueous veins by means of neoprene casts. Part I. Aqueous veins. Br J Ophthalmol. 1951;35:291.
41. Ascher K.W. Aqueous veins: preliminary notes. Am J Ophthalmol. 1942;25:31.
42. Gabfelt B.T. Kaufman PL: Aqueous humor hydrodynamics. In Kaufman P.L., Alm A., editors: Adler’s physiology of the eye, ed 10, St Louis: Mosby, 2003. p 293
43. Tamm E.R. The trabecular meshwork outflow pathways: structural and functional aspects. Exp Eye Res. 2009;88:648-655.
44. Selbach J.M., Gottanka J., Wittmann M., et al. Efferent and afferent innervation of primate trabecular meshwork and scleral spur. Invest Ophthalmol Vis Sci. 2000;41(8):2184.
45. Faralli J.A., Schwinn M.K., Gonzalez J.M.Jr., et al. Functional properties of fibronectin in the trabecular meshwork. Exp Eye Res. 2009;88:689-693.
46. Lund-Andersen H., Sander B. The vitreous. In: Kaufman P.L., Alm A., editors. Adler’s physiology of the eye. ed 10. St Louis: Mosby; 2003:293.
47. Johnson S., Chan D., Read A.T., et al. The pore density in the inner wall endothelium of Schlemm’s canal of glaucomatous eyes. Invest Ophthalmol Vis Sci. 2002;43(9):2950.
48. Allingham R.R., de Kater A.W., Ethier C.R. Schlemm’s canal and primary open angle glaucoma: correlation between Schlemm’s canal dimensions and outflow facility. Exp Eye Res. 1996;62(1):101. (abstract)
49. Rohen J., Unger H.H. Studies on the morphology and pathology of the trabecular meshwork in the human eye. Am J Ophthalmol. 1958;46:802.
50. Grierson I., Lee W.R., Abraham S. Effects of pilocarpine on the morphology of human outflow apparatus. Br J Ophthalmol. 1978;62:302.
51. Keil J.W., Reithamer H.A. Relationship between ciliary blood flow and aqueous production: does it play a role in glaucoma therapy? J Glaucoma. 2006;15:172-181.
52. Zimmerman T.J. Topical ophthalmic beta blockers: a comparative review. J Ocul Pharmacol. 1993;9:373-384.
53. Alm A. Uveoscleral outflow. Eye. 2000;14:488.
54. Lütjen-Drecoll E., Gabelt A.T., Tian B., et al. Outflow of aqueous humor. J Glaucoma. 2001;10(Suppl 1):42.
55. Cracknell K.P.B., Grierson I. Prostaglandin analogues in the anterior eye: their pressure lowering action and side effects. Exp Eye Res. 2009;88:786-791.
56. Toris C.B., Gabelt B.T., Kaufman P.L. Update on the mechanism of action of topical prostaglandins for intraocular pressure reduction. Surv Ophthalmol. 2008;53(Suppl1):S107-S120.
57. Chen H.B., Kashiwagi K., Yamabayashi S., et al. Anterior chamber angle biometry: quadrant variation, age change and sex difference. Curr Eye Res. 1998;17(2):120. (abstract)
58. Toris C.B., Yablonski M.E., Wang Y.L., et al. Aqueous humor dynamics in the aging human eye. Am J Ophthalmol. 1999;127:407.
59. Boldea R.C., Roy S., Mermoud A. Ageing of Schlemm’s canal in nonglaucomatous subjects. Int Ophthalmol. 2001;24:67.
60. Sebag J. The vitreous. In: Hart W.M.Jr., editor. Adler’s physiology of the eye. ed 9. St Louis: Mosby; 1992:268.
61. Mutlu F., Leopold I.H. Structure of the human retinal vascular system. Arch Ophthalmol. 1964;71:93.
62. Lund-Andersen H., Sebag J., Sander B., et al. The vitreous. In: Fischbarg J., editor. The biology of the eye. Amsterdam: Elsevier; 2006:181-194.
63. Wolter J.R. Pores in the internal limiting membrane of the human retina. Arch Ophthalmol. 1964;42:971.
64. Gaertner J. Vitreous electron microscopic studies on the fine structure of the normal and pathologically changed vitreoretinal limiting membrane. Surv Ophthalmol. 1964;9:219.
65. Matsumato B., Blanks J.C., Ryan S.J. Topographic variations in rabbit and primate internal limiting membrane. Invest Ophthalmol Vis Sci. 1984;25:71.
66. Malecaze F., Caratero C., Caratero A., et al. Some ultrastructural aspects of the vitreoretinal juncture. Ophthalmologica. 1985;191:22.
67. Russell S.R., Shepherd J.D., Hageman G.S. Distribution of glycoconjugates in the human retinal internal limiting membrane. Invest Ophthalmol Vis Sci. 1991;32(7):1986.
68. La Goff M.M., Bishop P.N. Adult vitreous structure and postnatal changes, Eye. 2008;22:1214-1222.
69. Schwarz W. Electron microscopic observations on the human vitreous body. In: Smelser G.K., editor. Structure of the eye. New York: Academic Press; 1961:283.
70. Balazs E.A. Functional anatomy of the vitreous. Tasman W., Jaeger E.A., editors. Duane’s foundations of clinical ophthalmology, vol 1. Philadelphia: Lippincott, 1994.
71. Eisner G. Clinical anatomy of the vitreous. Tasman W., Jaeger E.A., editors. Duane’s foundations of clinical ophthalmology, vol 1. Philadelphia: Lippincott, 1994.
72. Balazs E.A., Toth L.Z., Eckl E.A., et al. Studies on the structure of the vitreous body. XII. Cytological and histochemical studies on the cortical tissue layer. Exp Eye Res. 1964;3:57.
73. Sebag J., Balazs E.A. Morphology and ultrastructure of human vitreous fibers. Invest Ophthalmol Vis Sci. 1989;30:1867.
74. Bishop P.N., Takanosu M., Le Goff M., et al. The role of the posterior ciliary body in the biosynthesis of vitreous humour. Eye. 2002;16:454.
75. Pirie A., Schmidt G., Waters J.W. Ox vitreous humor. I. The residual protein. Br J Ophthalmol. 1948;32:321.
76. Matoltsy A.G. A study on the structural protein of the vitreous body (vitrosin). J Gen Physiol. 1952;36:29.
77. Gross J., Matoltsy A.G., Cohen C. Vitrosin: a member of the collagen class. J Biophys Biochem Cytol. 1955;1:215.
78. Bembridge B.A., Crawford C.N., Pirie A. Phase-contrast microscopy of the animal vitreous body. Br J Ophthalmol. 1952;36:131.
79. Osterlin S.E. The synthesis of hyaluronic acid in the vitreous. IV. Regeneration in the owl monkey. Exp Eye Res. 1968;7:524.
80. Hultsch E., Balazs E.A. In vitro synthesis of glycosaminoglycans and glycoproteins by cells of the vitreous. Invest Ophthalmol Vis Sci. 1973;14(Suppl):43.
81. Freeman M.I., Jacobson B., Balazs E.A. The chemical composition of vitreous hyalocyte granules. Exp Eye Res. 1979;29:479.
82. Ayad S., Weiss J.B. A new look at vitreous-humor collagen. Biochem J. 1984;218:835.
83. Szirmai J.A., Balazs E.A. Studies on the structure of the vitreous body. Cells in the cortical layer. Arch Ophthalmol. 1958;59:34.
84. Armand G., Chakrabarti B. Conformational differences between hyaluronates of gel and liquid human vitreous: fractionation and circular dichroism studies. Curr Eye Res. 1987;6:445.
85. Ponsioen T.L., Deemter M., Bank R.A., et al. Mature enzymatic collagen cross-links, hydroxylysylpyridinoline and lysylpyridinoline, in the aging human vitreous. Invest Ophthalmol Vis Sci. 2009;50:1041-1046.
86. Sebag J. Age-related differences in the human vitreoretinal interface. Arch Ophthalmol. 1991;109(7):966.
87. Kishi S., Shimizu K. Posterior precortical vitreous pocket. Arch Ophthalmol. 1990;108:979.
88. Teng C.C., Che H.H. Vitreous changes and the mechanism of retinal detachment. Am J Ophthalmol. 1957;44:335.
89. Wang J., McLeod D., Henson D.B., et al. Age-dependent changes in the basal retinovitreous adhesion. Invest Ophthalmol Vis Sci. 2003;44(5):1793.
90. Spalton D.J., Hitchings R.A., Hunter P.A. Atlas of clinical ophthalmology. Philadelphia: Lippincott; 1984. p 124
91. Lindner B. Acute posterior vitreous detachment and its retinal complications. Acta Ophthalmol Suppl. 1966;87:1.
92. Weber H., Landwehr G. A new method for the determination of the mechanical properties of the vitreous. Ophthalmic Res. 1982;14:326.
93. Weber H., Landwehr G., Kilp H., et al. The mechanical properties of the vitreous of pig and human donor eyes. Ophthalmic Res. 1982;14:335.
94. Holekamp N.M. The vitreous gel: more than meets the eye. Am J Ophthalmol. 2010;149:32-36.
95. Harocopos G.J., Shui Y., McKinnon M. Importance of vitreous liquefaction in age-related cataract. Invest Ophthalmol Vis Sci. 2004;45:77-85.
96. Melberg N.S., Thomas M.A. Nuclear sclerotic cataract after vitrectomy in patients younger than 50 years of age. Ophthalmology. 1995;102:1466-1471.
97. Shui B, Holekamp NM, Kramer BC, et al: The gel state of the vitreous and ascorbate-dependent oxygen consumption: relationship to the etiology of nuclear cataracts, Arch Ophthtalmol 127:475–482, 2009.