18 Anesthesia for Myocardial Revascularization
The role of the anesthesiologist in the perioperative care of patients presenting for myocardial revascularization continues to evolve. The anesthesiologist has to be well versed not only in a safe anesthesia technique, but in all areas of perioperative management in patients with coronary artery disease (CAD). This includes advances in pharmacologic risk reduction, new surgical techniques, and anesthetic management including monitoring techniques aiming to improve patient outcome. The overall number of patients presenting for coronary artery bypass graft (CABG) surgery has declined, mainly because of the growth of percutaneous coronary interventions (PCIs). Ischemic heart disease still accounts for approximately 1 of every 6 deaths in the United States. For 2010, it is estimated that 785,000 Americans will have a new heart attack, and every minute someone will die of it.1 Those patients who are not eligible for PCIs typically have an increased risk for perioperative morbidity and mortality and depend more than ever on optimal anesthetic management. This chapter provides a sequential approach to the major management issues faced by the practitioner who provides anesthesia for patients presenting for CABG surgery with cardiopulmonary bypass (CPB) or for off-pump coronary artery bypass (OPCAB) procedures.
Epidemiology
In 2010, it is estimated that 1 in 3 Americans in the United States have one or more types of cardiovascular disease, with CAD estimated to occur in 17,600,000 individuals in the United States. The total direct and indirect cost of all cardiovascular disease and stroke in the United States for 2010 is estimated to be $503.2 billion. Ischemic heart disease constituted 13.8% of the conditions of all Medicare beneficiaries in 2004; this number increased to 39.1% when only patients in the top 5% for all expenditures were considered.1 According to the same source, the number of cardiac catheterizations decreased slightly from 1996 to 2006 (Figure 18-1). There were 1,115,000 cardiac catheterizations performed in 2006, with 1,313,000 PCIs performed the same year.
Although precise data on the annual number of CABG and PCI procedures performed are not available, estimates based on sampling of large administrative and clinical databases, together with publications from discrete health care systems, have been used to derive strong estimates.2–4 The most widely cited data for nonfederal institutions in the United States are obtained from the U.S. Department of Health and Human Services National Hospital Discharge Survey.5 The latest data available estimated that, in 2006, 253,000 patients underwent a total of 448,000 CABG procedures.
Pathophysiology of coronary artery disease

Anatomy
The anesthesiologist should be familiar with coronary anatomy if only to interpret the significance of angiographic findings. An extensive review of cardiac anatomy can be found in reference cardiology or cardiac surgery texts.6,7 The following is an abbreviated description of the epicardial coronary anatomy. The coronary circulation and common sites for placement of distal anastomoses during CABG are shown in Figures 18-2 to 18-4.
The right coronary artery (RCA) arises from the right sinus of Valsalva and is best seen in the left anterior oblique view on coronary cineangiography (see Figure 18-2). It passes anteriorly for the first few millimeters, then follows the right atrioventricular groove, and curves posteriorly within the groove to reach the crux of the heart, the area where the interventricular septum (IVS) meets the atrioventricular groove. In 84% of cases, it terminates as the posterior descending artery (PDA), which is its most important branch, being the sole supply to the posterior-superior IVS. Other important branches are those to the sinus node in 60% of patients and the atrioventricular node in approximately 85% of patients. Anatomists consider the RCA to be dominant when it crosses the crux of the heart and continues in the atrioventricular groove regardless of the origin of the PDA. Angiographers, however, ascribe dominance to the artery, right coronary or left coronary (circumflex [Cx]), that creates the PDA.
The vertical and superior orientation of the RCA ostium allows easy passage of air bubbles during aortic cannulation, CPB, or open valve surgery. In sufficient concentration (e.g., coronary air embolus), myocardial ischemia involving the inferior LV wall segments and the right ventricle (RV) may occur (Figure 18-5). In contrast, the near-perpendicular orientation of the left main coronary ostium makes air embolization much less common.
The left coronary artery arises from the left sinus of Valsalva as the left main coronary artery. This is best seen in a shallow right anterior oblique projection (see Figure 18-3). The left main coronary artery courses anteriorly and to the left, where it divides in a space between the aorta and pulmonary artery. Its branches are the left anterior descending (LAD) and Cx arteries. The LAD passes along the anterior intraventricular groove. It may reach only two thirds of the distance to the apex or extend around the apex to the diaphragmatic portion of the left ventricle. Major branches of the LAD are the diagonal branches, which supply the free wall of the left ventricle, and septal branches, which course posteriorly to supply the major portion of the IVS. Although there may be many diagonal and septal branches, the first diagonal and first septal branches serve as important landmarks in the descriptions of lesions of the LAD (see Figure 18-4).
The Cx arises at a sharp angle from the left main coronary artery and courses toward the crux of the heart in the atrioventricular groove. When the Cx gives rise to the PDA, the circulation is left dominant, and the left coronary circulation supplies the entire IVS and the atrioventricular node. In approximately 40% of patients, the Cx supplies the branch to the SA node. Up to four obtuse marginal arteries arise from the Cx and supply the lateral wall of the left ventricle (see Figure 18-4). All of the previously described epicardial branches create small vessels that supply the outer third of the myocardium and penetrating vessels that anastomose with the subendocardial plexus. This capillary plexus is unique in that it functions as an end-arterial system. Each epicardial arteriole supplies a capillary plexus that forms an end loop rather than anastomosing with an adjacent capillary from another epicardial artery.8 Significant collateral circulation does not exist at the microcirculatory level. This capillary anatomy explains the distinct areas of myocardial ischemia or infarction that can be related to disease in a discrete epicardial artery (see Chapters 3 and 6).
CAD most commonly affects the epicardial muscular arteries with rare intramyocardial lesions (with the exception of the transplanted heart). However, severe disorders of the microcirculation and primary impairment of coronary vascular reserve in normal coronary arteries have been described, especially in diabetics, female patients, and those with variant angina.9–11 Atherosclerosis in all organs is most common at the outer edges of vessel bifurcations because in these regions blood flow is slower and changes direction during the cardiac cycle, resulting in less net shear stress (i.e., frictional force per unit area) than in other regions with more steady blood flow and higher shear stress.12 Low shear stress has been shown to stimulate an atherogenic phenotype in the endothelium. Epicardial lesions can be single but are more often multiple. A combined lesion of the RCA and both branches of the left coronary artery is referred to as triple-vessel disease. The left coronary artery supplies the thickest portions of the LV, at least the exterior two thirds of the IVS, and the greater part of the atria. Most bypass grafts are done on the left coronary system.
Venous drainage of the myocardium is primarily to the coronary sinus, which drains 96% of the LV free wall and septum, and the remainder of the venous return goes directly into the right atrium.7 A small fraction may enter other cardiac chambers directly through the anterior-sinusoidal, anterior-luminal, and thebesian veins.13

Myocardial Ischemia and Infarction
In patients with CAD, myocardial ischemia usually results from increases in myocardial oxygen demand that exceed the capacity of the stenosed coronary arteries to increase their oxygen supply (Figure 18-6). However, the determinants of myocardial oxygen balance are complex, and alterations may have several effects. For example, an increase in blood pressure (i.e., increased afterload) increases wall tension and oxygen demand while also increasing coronary blood flow (CBF). Myocardial ischemia may occur without changes in systemic hemodynamics and in awake patients may occur in the absence of chest pain (i.e., silent ischemia), particularly in patients with diabetes.
In atherosclerotic heart disease, the fundamental lesion is an intimal lipid plaque that causes chronic stenosis and episodic thrombosis, occurring most often in an epicardial coronary artery (Figure 18-7), thereby reducing myocardial blood supply. Characteristics of the vulnerable plaque include high lipid content, a thin fibrous cap, a reduced number of smooth muscle cells, and increased macrophage activity.14 The lipid core is the most thrombogenic component of the plaque.
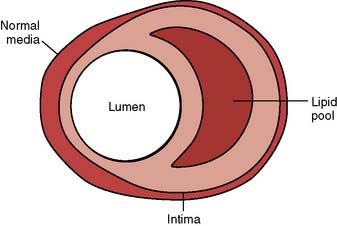
Figure 18-7 Lipoid plaque lesion of a coronary artery.
(From Davies MJ: A macro and micro view of coronary vascular insult in ischemic heart disease. Circulation 82(Suppl II):II-38, 1990, by permission of American Heart Association.)
Fuster et al15 described five phases in the progression of CAD by plaque morphology. Phase 1 is a small plaque present in many people younger than 30 years and usually progresses very slowly, depending on the presence of risk factors associated with CAD (i.e., increased low-density lipoprotein cholesterol). Phase 2 is a plaque with a high lipid content that has the potential to rupture. If it ruptures, it will lead to thrombosis and increased stenosis (phase 5), possibly producing unstable angina or an acute coronary syndrome. The phase 2 plaque usually does not rupture; it instead progresses into phases 3 and 4, with enlargement and fibrous tissue organization, which ultimately may produce an occlusive plaque at phase 5.
Acute coronary syndrome is produced by a sudden decrease in CBF. In unstable angina, a relatively small fissure in a plaque may produce a temporary thrombotic occlusion of a vessel.16 Release of vasoactive substances from platelets and white blood cells, as well as dysfunction of the endothelium, may lead to vasoconstriction and reduction of CBF.17,18 Reduced vasoconstriction, spontaneous thrombolysis, or the opening of collateral channels may limit the duration of myocardial ischemia. A larger plaque disruption and prolonged thrombosis will produce a Q-wave infarction with transmural myocardial necrosis. The potential lesions seen with plaque fissure are shown in Figure 18-8. Several studies have found that the coronary artery responsible for an acute infarction is often only moderately obstructed.19,20 It is the extent of plaque rupture and thrombosis that determines the size and extent of an infarction, rather than the degree of stenosis. Patients with severely obstructed coronary arteries often have extensive collateral circulations that protect them from infarction. Similar findings have been reported in patients developing postoperative myocardial infarction after noncardiac surgery.21–23
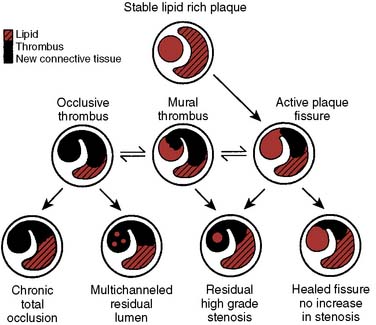
Figure 18-8 Possible outcomes of intimal plaque rupture.
(From Davies MJ: A macro and micro view of coronary vascular insult in ischemic heart disease. Circulation 82(Suppl II):II-38, 1990, by permission of American Heart Association.)
The physiology of the coronary circulation is reviewed in Chapter 6. CBF in the normal individual is independent of perfusion pressure, but it is related to tissue oxygen demand.7 This phenomenon is referred to as autoregulation. Autoregulation affects all layers of the ventricular wall, maintaining essentially equal flows from the epicardium to the endocardium.24,25 The difference between autoregulated flow and maximal flow constitutes the coronary vascular reserve. As an obstruction of a coronary artery increases in size, dilation of the capillary bed occurs, with maintenance of adequate blood supply as the result. Coronary flow reserve, however, is diminished and eventually exhausted, and autoregulation begins to fail. Autoregulatory failure is loss of metabolic control of CBF and the sole dependence on pressure gradients to determine flow. Autoregulation fails first in the subendocardium, where blood flow fails to match demand, resulting in subendocardial ischemia and dysfunction.11,26
Autoregulation is pressure dependent, in that as perfusion pressure declines below a critical value, autoregulation begins to fail. In conscious animals, the pressure at which autoregulation begins to fail is very low (mean arterial pressure [MAP] of 38 to 40 mm Hg).27 Because CBF reserve is diminished in CAD, the pressure at which subendocardial autoregulation fails is increased. Heart rate (HR) also appears to have an effect on autoregulation. In an unanesthetized animal model, when the HR was doubled, the perfusion pressure that produced failure of subendocardial autoregulation was increased from a mean of 38 mm Hg at the normal HR to a mean of 61 mm Hg at the doubled rate.28 This pressure is not that much less than normal perfusion pressures. This effect of HR on subendocardial autoregulation is related to the increased myocardial oxygen consumption and flow caused by tachycardia and to the reduction in diastolic time and, therefore, perfusion. The net effect is a reduction in coronary vascular reserve and earlier failure of autoregulation, with resultant subendocardial ischemia. Tachycardia, because of its effects on demand and supply (reduction in diastolic perfusion time), is especially deleterious in the presence of CAD, in which perfusion pressures beyond an epicardial arterial obstruction are unknown.29 Avoidance of hypotension and tachycardia has been the basic tenet of anesthetic practice in patients with CAD for many years.
A coronary arterial stenosis may be rigid or, more commonly (70%), compliant in nature.30 When the pressure in the coronary circulation distal to a fixed stenosis is decreased, flow across the stenotic area decreases. Conversely, when the pressure distal to a compliant obstruction is increased, flow across the lesion is increased.31 The clinical implication is that decreased blood pressure to an area of myocardium supplied by a vessel with a variable stenosis will decrease blood supply to the myocardium by two mechanisms: loss of collateral flow and decreased flow across the compliant stenosis. Collateral vessels exist in normal hearts, but in the presence of CAD, they are increased in size and number.32 When a coronary vessel has a high-grade stenosis, the microvasculature distal to it may be maximally dilated even at rest and subject to ischemia. Collaterals may develop between this ischemic zone and an adjacent nonischemic area supplied by a different vessel. When vasodilation of the microcirculation is induced by exercise or drugs, perfusion of the ischemic bed by the collateral circulation may be decreased or cut off, particularly when the collateral vessels are poorly developed, because these present a greater degree of resistance than collaterals that are well developed. When vasodilation occurs in the nonischemic bed, pressure within it is decreased, and flow across collateral vessels with high resistance is reduced. An increase in myocardial blood flow in one region that reduces flow in another is referred to as a coronary steal.33 In experimental models, a partial lesion of the epicardial artery supplying the collateral vessel is required to produce a coronary steal.34 This anatomic configuration, called steal-prone anatomy, occurred in 23% of patients with symptomatic CAD in one large registry of coronary angiograms.35
The hallmark symptom of myocardial ischemia is pain. It has been found, however, that significant ischemia may occur without pain (“silent ischemia”).36,37 Early research using ambulatory electrocardiographic (ECG) monitoring reported that these silent episodes were frequent and occurred at lower HRs and activity levels when compared with exercise stress test data for the same individuals.38 Silent ischemic episodes are not considered to be an electrical anomaly but are due to myocardial perfusion impairment resulting in reduced regional function.39 A study of patients presenting for elective CABG confirmed these findings in the perioperative period: 42% of 50 patients studied had ischemic episodes before surgery, 87% of these episodes were clinically silent, and few were precipitated by adverse hemodynamics.40 In summary, there is no doubt that primary reductions in myocardial oxygen supply because of hypotension, anemia, or coronary vasoconstriction are important mechanisms in the development of ischemia, particularly in the perioperative period.
Risk assessment in patients scheduled for coronary artery bypass grafting surgery
Operative mortality (usually defined as death within 30 days of surgery), as expected, varies widely with patient risk, acuity, and previous cardiac surgery. On average, however, it appears that mortality has declined progressively despite increases in patient risk (Figure 18-9).41 The rapid growth of PCI has led to the perception of a shunting of healthier patients away from CABG, leaving a greater percentage of older and sicker patients not studied in the original CABG efficacy trials (e.g., the seminal coronary artery surgery study [CASS] trial excluded patients with ejection fractions [EFs] less than 35%). Publications since 1980 addressing whether patients presenting for CABG are sicker or at greater risk than they were previously have uniformly answered this affirmatively, noting older patients, worse ventricular function, and more emergent cases.41 Despite these findings, however, operative mortality continues to decline. Davierwala et al42 observed that LV dysfunction and reoperative status have decreased in significance as predictors of mortality in a large cohort of Canadian patients, whereas emergency surgery has increased in importance in predicting adverse outcome.
Preoperative risk assessment for patients undergoing CABG has evolved dramatically since 1990, driven by a variety of diverse factors. Institution of a federally mandated accounting of surgical outcomes for cardiac surgery in the Department of Veterans Affairs in the 1970s led to the establishment of what is considered the first large-scale, multicenter surgical outcomes database applying rigorous statistical methodology for comparing outcomes between centers.43,44 This group and others have pioneered methodology for adjusting for different severities of illness between patients (i.e., risk adjustment) using multiple preoperative and perioperative variables thought to be of intrinsic value (usually by expert consensus) that easily could be captured and have high consistency of definition. Entering these variables into logistic regression models of mortality (considering all of the patients operated on in the particular time frame in which the variables were collected) allows determination of an “expected” mortality based on coefficients of the regression equation most correlated with outcome. By simple comparison of the “observed” to “expected” (i.e., the value calculated by entering a unique patient’s coefficients into the general population mortality model) mortality ratio (O/E ratio), hospitals in a particular system can be ranked from best (low O/E ratio) to worst (high O/E ratio).45 However, as observed by a variety of experts, caution is advised in use of such adjectives given inherent assumptions and controversies in the statistical approaches for determining thresholds for “quality” and problems in “gaming” of the system by exploiting variables with imprecise definitions.46
Nonetheless, this methodology and its variants have been adopted widely by many organizations as a measure of quality of care.47 The Society of Thoracic Surgeons (STS) instituted a voluntary clinical database system with this approach in the early 1990s, which has continued to grow rapidly as cardiac surgical groups are increasingly interested in benchmarking their practices against others.48,49 Many states have established and maintain risk-adjusted mandatory reporting systems for hospital and individual surgeon performance.50 A natural offshoot of this approach has been the exploration of surgeon and hospital volumes as predictors of outcome, a topic that has generated substantial controversy in the literature given conflicting findings (i.e., low-volume centers in which “high-volume” surgeons operate appear to do as well as high-volume centers, and low-risk patients do better relative to high-risk patients at high-volume centers).51,52 This is a hotly contested topic given the high stakes involved in federal and private sector initiatives to regionalize cardiac surgery care into “centers of excellence.”53 The EuroSCORE scoring system is based on outcomes in 128 centers in 8 European countries and has received increasing attention. It appears to compare favorably with the STS model in North American patients.54,55 It is freely accessible by means of an interactive Web-based calculator (www.euroscore.org) and is decidedly simpler and faster to use than the STS’s scoring system, which is now also freely accessible to the public (http://www.sts.org/sections/stsnationaldatabase/riskcalculator/index.html).
Given the mass of literature on this topic and the large number of risk models available, some of which are easily calculated (e.g., a simple additive score), whereas others require manipulation of a logistic regression equation on an advanced calculator or microcomputer, considerable debate exists about how they are best applied. The American College of Cardiology/American Heart Association CABG guidelines group accords a Class IIa recommendation (level of evidence C) to their use for predicting hospital mortality.56 However, they caution that risk scores should be individually calibrated for regional mortality rates (a difficult task) and updated periodically to maintain accuracy (many have not been, with the exception of the Department of Veterans Affairs program and the STS).
Table 18-1 presents an evaluation of the importance of risk factors in several major risk models and large-scale outcome analyses grouped by the classification that Jones et al57–63 advocated.
The major database models only predict perioperative mortality (usually defined as death within 30 days of surgery, although some use any death directly related to a perioperative complication regardless of the time interval, a factor further complicating comparison of models). As such, these models are often of less practical importance to the cardiac anesthesiologist. Predicting which patients are most likely to be difficult to wean from CPB because of development of a low cardiac output syndrome (LCOS) in the operating room or early postoperative period, or who may acquire postoperative complications, is of greater interest to the anesthesiologist. Despite publication of several studies, difficulty in standardization of what constitutes difficult weaning or LCOS has limited generalization of findings. However, given that most CABG mortality is related to difficulty in revascularization and/or myocardial protection, the risk factors are quite similar to those reported for overall mortality.64,65 An analysis of 1009 patients undergoing CABG at Duke University, all of whom were monitored with transesophageal echocardiography (TEE), found six independent predictors of inotrope support, which was required in 39% of the cohort during weaning from CPB (Table 18-2 and Figure 18-1066; see Box 18-1).
The introduction and rapid growth of OPCAB have complicated matters because large-scale, well-calibrated outcome models are not yet available for this procedure. Initial publications have addressed this topic.67,68
Considerable data have been reported on the potential impact of various anesthetic techniques on outcomes, particularly effects on pre-CPB myocardial ischemia and postoperative myocardial infarction. However, much of these data suffer from inadequate statistical power, use of single centers, and difficulties in standardization of definitions or reporting of surrogate (otherwise called soft) outcomes. Earlier reports supported a lack of effect of the anesthetic technique, suggesting that hemodynamic control was more important (e.g., “It’s not what you use, but how you use it.”), particularly for prevention of ischemia.69,70 However, newer data support various degrees of efficacy with different approaches for preserving ventricular function with volatile anesthetics on weaning from CPB,71,72 or high sympathetic blockade relative to intravenous techniques.73 Some of these have been linked to improved recovery or shorter lengths of stay. However, the advantages appear to be modest, and demonstration of overt reductions in serious morbidity or mortality in large-scale, multicenter cohorts has not yet been reported. Similar data suggest that although use of a specific anesthetic agent (e.g., opioid or neuromuscular agent) may facilitate earlier extubation, the small magnitude of effect in most instances does not appear to reduce overall length of hospital stay, have significant effects on recovery, or substantially reduce cost.74,75 Nonetheless, nearly all anesthesiologists continue to strive for the “perfect technique.”
Several publications have attempted to more precisely delineate the association of perioperative hemodynamics with outcomes in larger cohorts of patients than have been previously reported in the older randomized trials of anesthetic techniques. Reich et al76 merged computerized anesthesia record data from 2149 CABG patients (with CPB) at two New York hospitals from 1993 to 1995 with outcome data from the state’s mandatory reporting database. Four independent predictors of mortality were identified: high mean pulmonary arterial pressure (PAP) before CPB (>30 mm Hg; odds ratio [OR] = 2.1), low MAP during CPB (40 to 49 mm Hg; OR =1.3), tachycardia (HR > 120 beats/min; OR = 3.1), and high diastolic PAP (>20 mmHg; OR =1.2) after CPB. The investigators observed that three of the four are markers of severe LV dysfunction.
Morbidity and mortality also are influenced by events in the operating room, including time on CPB and aortic cross-clamping, adequacy of revascularization, and complications such as cardiovascular decompensation or bleeding. Reevaluation of the patient’s risk can be performed on arrival in the intensive care unit (ICU) at the start of the postoperative period. The APACHE (Acute Physiology and Chronic Health Evaluation) III system has been modified for CABG patients, and Becker et al77 found that predictors of outcome included the risk score, age, reoperative status, and number of grafts. Higgins et al78 devised the Cleveland Clinic ICU admission score, which includes preoperative and physiologic factors present on admission to the ICU. Morbidity rate was 3% with scores of 5 or less, and the rate increased to 83% with scores greater than 20.
The SYNTAX (Synergy between PCI with TAXUS drug-eluting stent and cardiac surgery) trial, a prospective, randomized, multicenter trial, originally was designed to evaluate current practice patterns and to evaluate optimal revascularization strategies in patients with three-vessel and left main CAD in Europe and the United States.79 For the purpose of risk stratification in this trial, the SYNTAX score was developed, characterizing the complexity of coronary pathology. The SYNTAX score is based on already existing classifications and takes number, location, complexity, and functional impact of the coronary lesions into consideration.80 Patients with more complex disease and potentially worse prognosis have greater SYNTAX scores. The initial analysis showed that there was no difference in outcome (major adverse cardiovascular and cerebrovascular events) among patients randomized to surgery between those who had low, intermediate, and high scores (major adverse, cardiovascular, and cerebrovascular events 14.4%, 11.7%, 10.7%, respectively).81 In the patients randomized to PCI, however, the SYNTAX score was able to predict adverse outcome between the earlier mentioned risk groups at 12 months (13.5%, 16.6%, 23.3%, respectively). Based on these findings, it was concluded that patients with a low-risk SYNTAX score constellation of three-vessel and/or left main disease can be treated with surgery, PCI, or both, whereas intermediate-and high-risk groups should be referred for surgery. Further analysis of the SYNTAX trial and associated risk score will be performed at medium and long-term time points.
Anesthesia for coronary artery bypass grafting

Role of Central Neuraxial Blockade
A wide variety of techniques have been used for anesthetic induction and maintenance for CABG. A balanced general anesthetic, however, is still the most commonly used anesthetic technique in patients undergoing CABG surgery. Nevertheless, the interest in the use of thoracic epidural anesthesia (TEA) for cardiac surgery has increased steadily since 1990. It has been long appreciated that thoracic sympathectomy has favorable effects on the heart and coronary circulation.82 Its coronary vasodilating effects have been well documented, and it has been used to treat unstable angina for many years, albeit infrequently because of logistics and the contemporary standard of use of potent antiplatelet agents. There has been increased interest in TEA including reports of using chronically implanted, patient-controlled catheters83–87 and its use as a supplement to general anesthesia for cardiac surgery, particularly in Europe and Asia (Figure 18-11).88–90
TEA in conscious patients appears to be increasingly used for OPCAB or minimally invasive direct coronary artery bypass approaches with recent reports from diverse settings (e.g., Canada, Germany, Turkey, India), and has been designated as conscious OPCAB.91–96 Although most reports were of relatively small patient cohorts (15 to 30), Karagoz et al97 described 137 patients, of whom 97% were successfully managed. In the reported series, most grafts were single-vessel left internal mammary artery (LIMA)-to-LAD grafts, although two-vessel and even a small group of three-vessel procedures were performed successfully. In most series, 2% to 3% of catheters were unable to be placed in potential candidates, and 2% to 3% of patients were converted to general anesthesia because of a large pneumothorax or incomplete analgesia. Patients were fast-tracked, an ICU stay was not used, and some were discharged from the hospital the day of surgery. Patient acceptance appeared to be quite high. No complications related to TEA were observed. This is clearly an area of growing interest and one that has potential advantages, particularly for countries with different health care systems, resource constraints, and sociocultural differences.
Given the fact that most conscious OPCABs are performed mostly for single-vessel LAD lesions, the sophistication and aggressiveness of the interventional cardiologists at a particular institution are major variables. Noiseux et al98 recently published a series of 15 patients undergoing conscious OPCAB surgery combining a high TEA with a femoral nerve block for venous graft harvesting. Three patients needed conversion to general anesthesia. The authors concluded that even though this technique is feasible, technical limitations still exist that need to be overcome.
When neuraxial techniques are compared with general anesthesia alone, or as a combined technique, no significant difference in measured major outcome parameters such as perioperative mortality and major morbidity were found in most studies.99–101 Differences in minor outcome findings such as quality of analgesia and time to extubation were reported in others. In a prospective study, Scott et al102 randomized 420 patients undergoing CABG surgery under general anesthesia to either TEA or intravenous narcotic analgesia. No neurologic complications were associated with TEA. Many of the examined outcome parameters such as time to extubation, pulmonary function, atrial fibrillation, and renal function were significantly better in the TEA group. However, this study was widely criticized for design flaws that may have impacted the reported findings.
Priestley et al103 consequently conducted another prospective, randomized study and did not find improved pulmonary or cardiac function with TEA or decreased length of hospital stay, despite improved analgesia and earlier extubation. In 2006, Hansdottir et al104 randomized patients who all had a general anesthesia for cardiac surgery to either TEA (inserted before surgery) or intravenous morphine for postoperative analgesia. Again, even though time to extubation was shorter in the TEA group, none of the other examined outcome parameters was significantly different between the two groups. The authors concluded that TEA combined with general anesthesia offers no major advantage when compared with general anesthesia alone. Bracco et al105 found fewer postoperative complications such as delirium, pneumonia, acute renal failure, and myocardial function in patients undergoing cardiac surgery who had TEA in addition to general anesthesia, compared with general anesthesia alone. Based on shorter ICU and mechanical ventilator times in patients with TEA, they calculated $8800 cost savings per person if TEA was used. A recently published randomized trial in obese patients (body mass index > 30 kg/m2) undergoing OPCAB surgery showed better analgesia, improved lung function tests, and shorter time to extubation and length of ICU stay in patients who had TEA in addition to general anesthesia.106 Liu et al107 reported a meta-analysis of 15 randomized trials of TEA in 1178 patients. In contrast with an earlier mixed meta-analysis (i.e., cardiac and noncardiac surgery, observational and randomized),108 there were no effects on postoperative myocardial infarction or mortality. However, significant favorable effects were observed for arrhythmias, pulmonary complications, time to extubation, and reduction in visual analog pain scales.
In a propensity-matched, retrospective study, Salvi et al109 compared high TEA combined with general anesthesia with a total intravenous anesthesia technique (without TEA) in 1473 patients undergoing CABG surgery. There were no major differences in measured early outcome parameters (postoperative mortality, myocardial infarction, stroke, acute renal failure, ICU stay) between the two techniques. Patients with high TEA had shorter time to extubation.
The potential advantages of TEA on cardiac function were investigated specifically in the following studies. Berendes et al110 reported improved regional LV function (by wall motion score index) with lower troponin I and atrial and brain natriuretic peptide levels. However, the control for this study was a total intravenous anesthetic technique, and it is unclear whether a volatile anesthetic would have similar effects, as demonstrated by several investigators. In a prospective, controlled study, Barrington et al111 randomized 120 patients to general anesthesia with or without high TEA. Even though postoperative analgesia was improved in the TEA group and led to earlier extubation, there was no significant difference seen with troponin levels between the two groups. Crescenzi et al112 evaluated the effect of TEA on N-terminal-protein-B-natriuretic peptide levels in elderly patients undergoing CABG surgery. TEA, in addition to general anesthesia, significantly attenuated N-terminal-protein-B-natriuretic peptide release. Lee et al113 addressed a slightly different question, using total spinal sympathectomy (bupivacaine, 37.5 mg) before induction of general anesthesia. Patients randomized to bupivacaine had less β-receptor dysfunction in response to CPB of more than 1 hour, with lower catecholamine levels, a greater cardiac index, and a lower pulmonary vascular resistance index in the post-CPB period.
Safety concerns are a major consideration in use of neuraxial techniques in patients undergoing cardiac surgery given chronic use of antiplatelet agents, use of systemic anticoagulation and platelet inhibition for acute therapy of unstable angina, and high-dose systemic anticoagulation and potential coagulopathy induced by CPB. The true incidence of serious complications (particularly epidural hematoma) is unknown. The most recent and widely quoted estimation of the risk for epidural hematoma with TEA in patients undergoing cardiac surgery is 1 in 12,000, with 95% confidence intervals of 1:2,100 to 1:68,000, and 1 in 1000 with 99% confidence.114 Intrathecal risks from a different older source of risk assessment are quoted as 1 in 3610 and 1 in 2400, respectively.115 Even when under-reporting of such complications is assumed,116 there is increasing evidence that neuraxial anesthesia can be performed safely even in patients undergoing cardiac surgery with full heparinization.
Chakravarthy et al117 presented an audit of 2113 cardiac surgery TEA cases over a 13-year period with no permanent neurologic deficits, a 0.9% dural puncture rate, and 0.2% transient neurologic deficits. Jack et al published their experience of thoracic epidural catheter placement in 2837 patients undergoing cardiac surgery.118 No epidural hematoma was seen in this series. Similar results were reported by Royse et al,119 who reviewed 874 cardiac surgery cases involving epidural anesthesia over a 7-year period with no complications attributable to epidural catheter use. Pastor et al120 reported 714 uneventful cases over a 7-year period, emphasizing their use of safety guidelines in which antiplatelet drugs were discontinued 7 days before surgery, and routine coagulation tests and neurologic examinations were performed after surgery.
Careful attention to the most recent guidelines on neuraxial anesthesia in the setting of anticoagulant and antiplatelet agents is of paramount importance. The American Society of Regional Anesthesia and Pain Medicine published Consensus Statements on Neuraxial Anesthesia and Anticoagulation.121 These include recommendations for appropriate withdrawal of anticoagulant and antiplatelet therapy before neuraxial anesthesia (these recommendations can be found online at: www.asra.com). Chaney122 also has published an extensive review on the use of intrathecal and epidural anesthesia and analgesia for patients undergoing cardiac surgery.

Premedication
Anxiolysis, Amnesia, and Analgesia
The sedative and anesthetic-sparing actions of α2-adrenergic agonists (e.g., clonidine, dexmedetomidine) have been evaluated for their efficacy in several studies of CABG patients alone or in combination with a benzodiazepine.123–125 The use of α2-adrenergic agonists reduced stress response and anesthetic requirements compared with conventional regimens. A meta-analysis reported reductions in mortality and postoperative myocardial infarction with their use in cardiac surgery (started before surgery and often continuing until CPB).126 Nevertheless, some of the hemodynamic side effects of α2-agonists warrant caution in certain patient populations. Decreased HR, MAP, cardiac output (CO), contractility, and transiently increased systemic vascular resistance (SVR) seen with the less-selective α2-blocker clonidine, as well as an increased risk for hypotension during anesthesia induction, have been reported in general and cardiac surgery patients who were premedicated with α2-agonists.127,128 Consequently, α2-agonists should be used with caution in patients with preexisting severe bradycardia, conduction problems such as second- or third-degree heart block, hypovolemia, or hypotension.
Management of Antianginal and Antihypertensive Medications
The use of β-blocking agents in patients with poor ventricular function has been evaluated. Contrary to earlier reports,129 Kaplan et al130,131 reported in the mid-1970s and long before the ongoing discussion of perioperative β-blockade in noncardiac surgical patients that it was safe to continue β-blockade in patients presenting for cardiac and noncardiac surgery. Slogoff et al132 performed a randomized trial evaluating the safety of administration of propranolol within 12 hours of surgery. Based on a significantly greater increase in the incidence of pre-CPB ischemia in patients withdrawn from propranolol (within 24 to 72 hours), they also recommended continuation of therapy up until the time of surgery. Further work by these and other investigators in the 1980s documented the efficacy of β-blocker continuation through CABG surgery with regard to reducing pre-CPB ischemia and their superior efficacy over the increasingly popular calcium channel blockers133,134 (Box 18-2).
BOX 18-2 Preoperative Medication Management
The earlier mentioned studies were instrumental in laying the groundwork for the subsequent noncardiac surgery studies in the late 1980s.135–137 They led to the contemporary randomized trials of Poldermans et al138 and Mangano et al,139 which rallied support for routine perioperative β-blocker use; and the most recent conclusions drawn from the POISE trial140 that questioned parts of this practice. These trials resulted in the latest recommendations of β-blockade in patients undergoing noncardiac surgery.141 There is little doubt that β-blockers are beneficial for most CABG patients, particularly if HR is increased. When acutely administered in adequate dose, they significantly reduce myocardial oxygen demand and the incidence of atrial and ventricular arrhythmias. Several observational studies have documented associations of β-blocker therapy with reduction in perioperative mortality in CABG patients.142,143 The largest of these by Ferguson et al144 considered 629,877 patients in the STS database (1996 to 1999) in which a modest but statistically significant reduction in 30-day risk-adjusted mortality was reported. This treatment effect was observed in many high-risk subgroups, although a trend toward increased mortality was seen in patients with EF less than 30%. In a meta-analysis, Wiesbauer et al145 found that perioperative β-blockers reduced perioperative arrhythmias after cardiac surgery, but they had no effect on myocardial infarction or mortality. Considerable efforts are being expended by major organizations (STS, American College of Cardiology) in increasing compliance with existing guidelines for use of β-blockers at the time of hospital discharge (together with use of aspirin, statins, and angiotensin-converting enzyme [ACE] inhibitors).146,147
An increasing number of patients are presenting for CABG surgery while being treated with platelet inhibitors. Aspirin is a well-recognized component of primary and secondary prevention strategies for all patients with ischemic heart disease.148,149 Treatment with clopidogrel is required for coronary artery stent placement, has been shown to improve outcome, and is now recommended in combination with aspirin after acute coronary syndrome.150,151 Antiplatelet therapy is also used after CABG to reduce ischemic complications. Aspirin (and other platelet inhibitors such as dipyridamole) have long been recognized to have strong efficacy in the prevention of early graft thrombosis after CABG.152 Mangano et al,153 in a large observational analysis, reported substantial reduction in overall mortality rate (1.3% vs. 4.0%) and ischemic complications of the heart, brain, kidneys, and gastrointestinal tract in 5065 patients at 70 hospitals when aspirin was administered within 48 hours after surgery.
The combination of aspirin and clopidogrel after CABG may be even more effective.154 However, great controversy exists in regard to preoperative antiplatelet therapy. The risk for hemorrhagic complications needs to be weighed against the potential benefits of antiplatelet therapy. In a recent meta-analysis, patients receiving aspirin immediately before surgery had more mediastinal bleeding and received more blood products.155 Several retrospective studies have reported that CABG surgery in patients receiving clopidogrel is associated with increased bleeding and transfusion requirements.156 Filsoufi et al157 also reported longer ICU and hospital length of stay (LOS) and increased all-cause morbidity and mortality in clopidogrel-treated patients. In a multicenter, retrospective study, Berger et al158 reported that clopidogrel-treated patients were at increased risk for reoperation, major bleeding, and increased LOS.
Recent guidelines from the American College of Chest Physicians on antithrombotic and thrombolytic therapies recommend institution of aspirin within 6 hours after CABG surgery over continuation of preoperative therapy (level of evidence IIa).159 In those with ongoing bleeding, it should be administered as soon as possible thereafter. In those allergic to aspirin, clopidogrel should be used instead. Low-dose aspirin should be continued indefinitely. They further recommend the use of clopidogrel in addition to aspirin for 9 to 12 months after CABG after a non–ST-elevation acute coronary syndrome. The STS has released formal practice guidelines on this topic. In high-risk patients (those with unstable angina or recent myocardial infarction) requiring urgent or emergent CABG, aspirin should be continued until the time of surgery (Class IIa recommendation) unless they are in an “aspirin-sensitive high-risk subgroup” (i.e., those on other antiplatelets or anticoagulants, or those who have platelet abnormalities). For elective patients in whom active platelet aggregation is less likely to be a critical factor in precipitating ischemia, they recommend discontinuation of aspirin for 3 to 5 days before surgery to reduce transfusion requirements (Class IIa), with reinstitution in the early postoperative period (Class I).160 The American College of Chest Physicians practice guidelines, as well as the American Heart Association/American College of Cardiology guidelines, also suggest discontinuing clopidogrel 5 days before CABG surgery in patients who received clopidogrel for acute coronary syndrome if clinical circumstances allow.159,161
The efficacy of calcium channel antagonists with regard to their anti-ischemic properties in patients undergoing CABG is a controversial topic. Early observational studies suggest they were ineffective, particularly relative to β-blockers.162,163 Subsequently, there was even less enthusiasm for their use given concerns in the mid-1990s of excess mortality with shorter-acting preparations (in particular, nifedipine, which was thought to cause reflex adrenergic activation because of abrupt vasodilation with each dose).164 Two meta-analyses evaluating their efficacy in noncardiac surgery produced conflicting information.165,166 However, one meta-analysis and a large observational cohort study with propensity matching adjustment suggest they are effective in reducing mortality in CABG patients.167,168 For patients taking them chronically, it appears prudent to continue them perioperatively. Caution is advised with concurrent administration of nondihydropyridine drugs (diltiazem) and β-blockers, although the risk (purported to be possible precipitation of advanced degrees of atrioventricular block) appears to be minor, based on a lack of problems in a reported large cohort of thoracotomy patients in whom this was done frequently.169 A new issue recently has been raised as well, which will require further investigation. A recent observational study concluded that calcium channel blockers decrease clopidogrel-mediated platelet inhibition, the latter playing a crucial role in the management of CAD.170
ACE inhibitors and 3-hydroxy-3-methylglutaryl coenzyme A reductase inhibitors (statins) currently are receiving special attention as agents because of a variety of important “pleiotropic” effects (e.g., effects independent of their primary antihypertensive or lipid-lowering actions, respectively).171–173 Potent anti-inflammatory and antithrombotic effects and beneficial effects on endothelial function have been reported for both agents, as well as less clear effects on angiogenesis.174,175 Both agents commonly are administered acutely during PCI,176–178 and have direct effects on platelet aggregation and plasminogen activator inhibitors.173,179 Statins have been reported to reduce circulating levels of adhesion molecules, which have been implicated in endothelial dysfunction after CPB (Figure 18-12).180–182 Statins also have been shown to attenuate myocardial reperfusion injury after cardiac surgery.183 ACE inhibitors are widely considered to be vasculoprotective, particularly with regard to ventricular remodeling after acute myocardial infarction, and they appear to reduce damage after ischemic reperfusion (likely related to reduction in ischemia-induced vasoconstriction and reduction in leukocyte adhesion).184 Several investigators have published retrospective studies with similar reports of the efficacy of statins to reduce the short-and long-term mortality of CABG patients.185–189 In a large meta-analysis evaluating the impact of preoperative statin use on adverse clinical outcomes after cardiac surgery, Liakopoulos et al190 reported that preoperative statin use significantly reduced all-cause mortality after surgery. Statins also have been found in retrospective studies to decrease the need for postoperative renal replacement therapy,191 possibly a reflection of their anti-inflammatory properties, with conflicting results regarding a reduction in acute renal dysfunction.192,193 In addition, statins have been shown to decrease the incidence of atrial fibrillation after cardiac surgery.194 The current American College of Cardiology/American Heart Association guidelines on perioperative cardiovascular evaluation and care for noncardiac surgery patients recommend the continuation of statins in patients currently on them and the consideration of starting statins in patients with clinical risk factors.195 Most clinicians continue them routinely (albeit orally only, so there is usually a short withdrawal period after surgery). Postoperative withdrawal of statin treatment is independently associated with increased hospital mortality after CABG.196
Fewer studies have been performed on ACE inhibitors. The QUO VADIS (QUinapril on Vascular Ace and Determinants of Ischemia) study197 showed a significant reduction in ischemic events in patients on ACE inhibitors before CABG. ACE inhibitor therapy before CABG has also been associated with a reduced risk for acute kidney injury198 and may be effective in the prevention of new-onset atrial fibrillation.199 ACE inhibitors, however, also have been associated with greater degrees of hypotension during induction or even profound degrees of vasodilation (vasoplegic syndrome) during CPB and weaning because of their vasodilatory effects.200–208 In a large, retrospective, observational study on more than 10,000 patients undergoing CABG surgery, preoperative ACE inhibitor therapy was associated with an increased incidence of perioperative hypotension and ACE inhibitor therapy was found to be an independent predictor of mortality, need for inotropic support, postoperative renal dysfunction, and new-onset postoperative atrial fibrillation.209 Another concern expressed by Lazar210 was in relation to potential antagonism between ACE inhibitors and aspirin because ACE inhibitors increase prostaglandin levels, whereas aspirin inhibits them. Despite strongly promoting their perioperative use, he advised they be withheld for 24 to 48 hours before surgery if possible and restarted after surgery after reinstitution of β-blockade (assuming systolic blood pressure > 100 mm Hg). They are otherwise contraindicated with renal insufficiency, and their adverse effect of cough can be detrimental to sternal stability in the early postoperative period. At this point, further studies are needed to make recommendations about the perioperative use and/or continuation of ACE inhibitors and their effect on outcome parameters in patients undergoing CABG surgery.

Monitoring
Electrocardiogram
On arrival in the operating room, the patient undergoing CABG should have routine monitors placed, including pulse oximetry, noninvasive blood pressure (BP), and the ECG. A five-lead system is standard in patients undergoing cardiac surgery. Monitoring leads V5 and II allows for the detection of 90% of ischemic episodes, as well as monitoring the rhythm to diagnose various atrial and ventricular arrhythmias. The ECG detection of myocardial ischemia is reviewed in Chapter 15 (Box 18-3).
BOX 18-3 Intraoperative Monitoring for Myocardial Revascularization
Arterial Pressure Monitoring
The radial artery usually is cannulated for BP monitoring during CABG. Choosing the best site for radial artery cannulation depends on surgery-specific considerations, as well as institutional and practitioner preferences. Surgical technique such as radial artery harvesting or axillary CPB cannulation may influence the site chosen for invasive arterial pressure monitoring. With modern sternal retractors, blunting of the arterial pressure tracing on the ipsilateral side of internal mammary artery (IMA) dissection is not typically seen. Some practitioners use bilateral arterial cannulation or choose a more central artery such as the axillary or femoral artery to ensure accurate pressure readings after CPB. Radial arterial pressures have been shown to be inaccurate immediately after hypothermic CPB. Substantial reductions in radial arterial versus aortic pressure have been reported in several clinical investigations, often requiring 20 to 60 minutes after CPB to resolve.211–215 Alterations in forearm vascular resistance (decrease) are believed to be responsible for this common phenomenon. This problem can be overcome by temporarily transducing the arterial pressure directly from the aorta (by a needle or a cardioplegia cannula).
Central Venous Cannulation
The placement of a central venous pressure (CVP) catheter routinely is performed in cardiac anesthesia both for pressure measurement and for infusing vasoactive drugs. Some centers routinely place two catheters (a large introducer and a smaller CVP catheter) in the central circulation to facilitate volume infusion and vasoactive or inotropic drug administration. Increasingly, ultrasound guidance is used for placement, although there remains controversy in the literature regarding whether it is a standard of care216–218 (see Chapter 14).
Pulmonary Artery Catheterization
The use of PAC in medical and surgical settings has declined steadily, mostly because of the increasing amount of data from large randomized studies showing that major clinical outcomes (particularly mortality) are not changed by PAC use and that adverse effects of PAC monitoring have to be considered. This includes surgery for myocardial revascularization and in the ICU setting, suggesting that despite the substantial amount of physiologic information obtained, patient outcome is independent of PAC use. Tuman et al,219 in a prospective observational study, examined the effect of the PAC on outcome in 1094 patients undergoing CABG surgery. Although no direct data on LV function were provided, there was no difference in the incidence of LV dysfunction between the group treated with a CVP versus the group treated with a PAC. In this study, the investigators could not demonstrate that a PAC had any effect on outcome; however, 7% of the patients initially assigned to CVP monitoring subsequently required a PAC for management.
Several other reports have focused exclusively on PAC use in CABG surgery. Stewart et al220 reported a retrospective analysis of 312 patients undergoing CABG (in 1996) who were believed to be low risk and suitable for CVP monitoring alone. Of these, 32% had a PAC placed and received greater volumes of fluid, gained more weight, and had longer times to extubation. Ramsey et al221 retrospectively analyzed a commercial health care outcomes benchmarking database with 13,907 patients undergoing nonemergent CABG in 56 hospitals (Figure 18-13). Patients who had a PAC placed for perioperative monitoring (58% of the patients) were found to have a greater risk for mortality after risk adjustment (relative risk = 2.1), longer lengths of stay, and higher total costs, particularly in the hospitals with low rates of PAC use. Schwann et al222 retrospectively analyzed 2685 consecutive CABG patients at a single private center (1994 to 1998) in which PAC use was “highly selective” (i.e., used in only 9% based on consideration of multiple cardiac risk factors). Of these PACs, 6.6% were planned, with the remainder placed after surgery in response to adverse intraoperative events. Multivariate analysis revealed EF, STS risk score, use of IABP, congestive heart failure, redo operation, and New York Heart Association Class IV to be independent predictors of PAC use. Based on their reported overall mortality rate (2.3%), it appears as if this highly selective approach was safe, although these data cannot necessarily be generalizable outside this particular center given multiple other process variables involved in providing care for these patients. London et al223 documented the high rate of PAC use based on analysis of 3256 CABG patients included in a larger multicenter, observational study in patients undergoing cardiac surgery in the Department of Veterans Affairs (1994 to 1996). More than 95% of all cases were monitored with a PAC, and 49% of these used the more expensive mixed venous oxygen saturation catheter. Use of this catheter was clearly center specific, and with the exception of a small reduction in number of postoperative arterial blood gas and thermodilution CO measurements, it was not associated with improvement in outcome over the routine PAC.
Based on the existing literature, it is not possible to give precise criteria for PAC use in CABG surgery (see Chapter 14). The greater the patient risk (based primarily on established preoperative clinical predictors), the more favorable is the risk/benefit ratio. Risk factors include significant impairment of ventricular function (EF < 30%), and patients with known pulmonary hypertension and/or right-heart failure. Some authors have advocated a wait-and-see approach. In a prospective observational study, Djaiani et al224 showed the safety and usefulness of delaying the insertion of a PAC until the clinical need arises in the operating room or in the ICU after CABG surgery.
Although most of the recent clinical reports of patients undergoing OPCAB have used and many recommend the use of a PAC, it is not possible to give firm recommendations on this because of the lack of evidence-based data. In a retrospective study, Resano et al225 did not find significant differences in mortality, conversion to on-pump procedure, or inotropic drug use between the group treated with a CVP versus the group treated with a PAC.
Many of the complications reported with PAC use are related to large-bore catheter central vein cannulation. With the increasing use of intraoperative TEE monitoring, PAC use may decrease even more. For a more detailed review of PAC use, see Chapter 14.
Transesophageal Echocardiography
The ASA, together with the Society of Cardiovascular Anesthesiologists, developed practice guidelines in 1996 to provide recommendations for the perioperative use of TEE.226 These guidelines were updated recently,227 and the routine use of TEE is now recommended for all cardiac or thoracic aortic surgery, which includes most patients undergoing CABG or OPCAB surgery, or both. The ASA Task Force thereby acknowledged the increasing evidence that TEE can provide important information that may impact perioperative anesthetic and surgical management and, possibly, patient outcome.
TEE is highly sensitive but not specific for myocardial ischemia.228,229 It is appreciated that the earliest signs of myocardial ischemia include diastolic dysfunction followed by systolic regional wall motion abnormalities (RWMAs), which occur within seconds of acute coronary occlusion. New RWMAs detected in the intraoperative period, however, frequently may occur because of nonischemic causes such as changes in loading conditions, alteration in electrical conduction in the heart, post-CPB pacing, myocardial stunning caused by ischemia before or during weaning from CPB, or poor myocardial preservation. Worsening of RWMAs after CABG surgery is associated with an increased risk for long-term adverse cardiac morbidity and has been suggested as a prognostic indicator of adverse cardiovascular outcome.230 The transgastric short-axis midpapillary muscle view, commonly used because of its inclusion of myocardium supplied by the three major coronary arteries, may entirely miss RWMAs occurring in the basal or apical portions of the heart. A comprehensive TEE examination recommended by the American Society of Echocardiography/Society of Cardiovascular Anesthesiologists Task Force before and after CPB or after completion of revascularization in OPCAB is, therefore, recommended.231 TEE is not perfect for ischemia monitoring because all wall segments would have to be monitored continuously in real-time and compared with preoperative findings.
A new and exciting application of perioperative TEE is now emerging. The conventional method for analyzing regional myocardial function is by visual assessment of inward radial motion and wall thickening from two-dimensional (2D) echocardiographic images. Precise and reproducible quantitative assessment is much more appealing. Early attempts at using Doppler-based techniques for this purpose have been limited by angle-dependency and artifacts.232,233 A novel quantitative approach is speckle tracking. It uses 2D images and analyzes the movement of stable acoustic markers (speckles) between frames.234 Kukucka et al235 demonstrated the feasibility of intraoperative determination of speckle tracking–derived strain from TEE images and correlated it with visual assessment of RWMA. They showed that it had better interobserver agreement than visually obtained data, allowed for determination of radial and longitudinal RWMAs, and that strain analysis and not visually obtained semiquantitative assessment of wall motion detected differences between normally perfused and ischemic segments (see Chapter 12).
The use of perioperative TEE in patients undergoing cardiac surgery is increasing, and it will be interesting to see how this trend develops with the newly adopted ASA guidelines on the perioperative use of TEE in patients undergoing cardiac surgery. Morewood et al236 obtained more than 1800 survey responses from members of the Society of Cardiovascular Anesthesiologists in 2000. Of approximately 1500 clinicians involved in CABG, only 11% reported never using TEE, but more than 30% used it frequently or always. This number is probably greater today because of the growth of OPCAB and ongoing popularity of the technology. A variety of observational studies since 1990 have supported the efficacy of this technique, although in the absence of a true randomized, controlled trial, it is impossible to conclusively prove the efficacy of intraoperative TEE on patient outcome parameters such as perioperative morbidity and mortality.237–239 Proper education and certification are critical, and guidelines about training requirements and certification have been published240,241 (see Chapter 41). The clinician must realize that there are serious complications that can and do occur (albeit rarely) with the intraoperative use of TEE and that a strong association between esophageal dysmotility and aspiration, particularly in elderly patients, has been suggested in several observational reports.242–247
Cerebral Oximetry
Cerebral oximetry monitoring, a continuous, noninvasive monitor of regional cerebral oxygen saturation, is being used increasingly during cardiac surgery. The technologic background of near-infrared spectroscopy technology has been reviewed in detail elsewhere (see Chapter 16).248 The main principles on which near-infrared spectroscopy devices rely are the facts that most biologic tissues, other than hemoglobin and cytochrome oxidase, are relatively transparent to infrared light in the range closest to the visual spectrum (700 to 1000 nm) and that the absorbance spectrum of hemoglobin depends on its oxygenation status (deoxygenated hemoglobin absorbs more red light and less infrared light than oxygenated hemoglobin). Regardless of the manufacturer, all devices emit light at wavelengths within the earlier mentioned spectrum and analyze photons returning to the transducer. The source of the light signal(s) (laser vs. diode), number of wavelengths used, and distance of the emitting light source to the receiving sensors vary among the manufacturers. Because the change in intensity of the reflected light is dependent on the oxyhemoglobin/deoxyhemoglobin ratio, oxyhemoglobin saturation can be derived.249 Because the greatest contribution to a tissue’s absorption spectrum is from blood contained within venules and veins (approximately 3:1 ratio of venous to arterial blood), these devices provide a venous weighted value.250 In comparison, pulse oximetry provides measurement of an arterial oxygen saturation reflecting oxygen supply to tissue. Therefore, near-infrared spectroscopy technology is considered complementary to pulse oximetry. Unlike pulse oximetry that requires pulsatile flow, tissue oximetry does not and is, therefore, ideal in low-flow conditions such as LCOS and/or nonpulsatile CPB or cardiocirculatory assist devices.
The use of cerebral oximetry has been suggested for cardiac surgical patients for several reasons. It is unique in its ability to continuously monitor regional tissue oxygenation even in the LCOS and nonpulsatile flow, the latter commonly seen during CPB. Multiple case reports have demonstrated that cerebral oximetry can provide early warning signs for detecting catastrophic events otherwise not detected by other monitoring devices such as pulse oximetry.251 In addition, the cerebral cortex can be seen as an index organ. Although autoregulatory mechanisms have to be considered, low cerebral tissue oxygenation correlates with measures of systemic oxygen delivery and consumption.252 Postoperative cognitive dysfunction is still one of the most frequently reported complications after cardiac surgery. It is likely multifactorial in origin, but embolization and hypoperfusion of the brain are two of the most frequently cited causative factors.253 Although cerebral oximetry, because of its localized area of interrogation, may not detect even massive particulate emboli to the brain resulting in catastrophic neurologic adverse outcomes such as stroke, these events fortunately are rare, with rates of 1% to 3% reported in the literature (see Chapter 36).254
Many studies have estimated the incidence of neurocognitive dysfunction after cardiac surgery to be greater than 50%.255 There are emerging data that a correlation exists between cerebral oxygen desaturations (measured with cerebral oximetry) and cognitive dysfunction in CABG patients.256,257 There has been an ongoing debate, however, about the clinical value of near-infrared spectroscopy monitoring as a trend monitor only or as a noninvasive tool that allows clinical decision making based on adequate correlation with absolute measurements. There still need to be data from large, randomized, controlled studies with clearly defined treatment protocols and outcome measures to demonstrate that interventions based on cerebral oximetry readings can improve neurologic outcome in CABG patients.258 Two prospective trials in CABG patients that fit those criteria have been published to date. Slater et al257 randomized 265 CABG patients to a blinded control group or an unblinded intervention group. There were no statistically significant differences in cognitive decline and major postoperative complications (cerebrovascular accident, myocardial infarction, renal insufficiency, reoperation for bleeding) between the two study groups, a result the authors attributed to poor compliance with the treatment protocol. In the multivariate analysis, however, prolonged rSo2 desaturation was an independent risk factor for postoperative cognitive decline regardless of the assigned study group. Murkin et al,259 in a similar study, demonstrated that treatment of cerebral oxygen desaturations improved outcome in patients undergoing CABG surgery. They randomized 200 patients undergoing CABG surgery to either an intervention group in which cerebral tissue desaturation was linked to a treatment intervention protocol attempting to correct those readings back to baseline values or a control group in which cerebral oximetry readings were blinded to the practitioner. The hypothesis was that most of the interventions to optimize cerebral oxygen saturation would influence systemic perfusion as well. There was no difference in the overall incidence of adverse complications; however, significantly more patients in the control group had major organ morbidity or mortality such as death, ventilation longer than 48 hours, stroke, myocardial infarction, and return for re-exploration. The results of both studies clearly demonstrated that further studies are needed to determine whether postoperative cognitive dysfunction can be reduced by treatment of intraoperative cerebral oxygen desaturations, as well as to define a clear threshold below which the risk for postoperative cognitive dysfunction is increased.

Induction and Maintenance of General Anesthesia
It is evident that no single approach to anesthesia for CABG procedures is suitable for all patients. Most hypnotics, opioids, and volatile agents have been used in different combinations for the induction and maintenance of anesthesia, with good results in the hands of experienced clinicians (Box 18-4).
Anesthetic Agents
Thiopental had been used for decades for induction in this setting; however, it is rarely used nowadays. Its predominant hemodynamic effects include reductions in MAP and CO accompanied by a modest increase in HR. These are believed to result from a combination of direct myocardial depression, venodilation, and a decrease in central sympathetic outflow. In isolated muscle, whole-animal,260 and clinical studies (including use of load-independent measures of contractility),261,262 it generally is reported to have greater negative inotropic effects than propofol. A greater degree of vasodilation with propofol also may account for less depression of CO.263,264 A study of isolated human atrial muscle from CABG patients reported no effect of propofol, midazolam, and etomidate on contractility in contrast with strong effects for thiopental and, paradoxically, slight negative effects for ketamine.265 However, a sheep preparation, in which “site-directed” coronary arterial injection was used to isolate direct cardiac effects using doses small enough to preclude indirect effects from recirculated drug in the central nervous system, reported similar direct cardiac depressant effects for thiopental and propofol (including the thiopental enantiomer and racemate).266 Despite these findings and its use in epidemiologic studies of anesthetic techniques for CABG,267 the use of thiopental in most centers has declined substantially in favor of propofol. Adverse effects on airway resistance, a greater propensity to elicit bronchospasm, and a greater association with postoperative nausea and vomiting are other potential factors (see Chapter 9).
Administration of ketamine generally is associated with increases in HR and MAP through indirect central and peripheral sympathetic stimulation (e.g., inhibition of neuronal reuptake of catecholamines). In states associated with depletion of catecholamines and in isolated preparations, ketamine appears to have direct negative inotropic and vasodilating effects,265,268 and it may have a negative lusitropic effect decreasing diastolic compliance.269 In a double-blind, randomized, controlled trial, Zilberstein et al270 documented a potent anti-inflammatory effect (i.e., suppression of increases in superoxide anion production after CPB) with a very small dose of ketamine (0.25 mg/kg) that persisted for several days after surgery. Ketamine is used relatively infrequently in CABG patients and is reserved primarily for induction in those with severe reduction of EF.
Etomidate appears to have minimal or no direct negative inotropic effects or sympathomimetic effects.271,272 In an isolated rabbit heart preparation, Komai et al273 demonstrated that at very high concentrations, etomidate inhibited the influx of extracellular calcium but had no effect on availability of intracellular calcium required for excitation-contraction coupling. It is known to inhibit adrenal mitochondrial hydroxylase activity, resulting in reduced steroidogenesis even after a single bolus dose, although the studies are conflicting.274,275 Its use for induction in cardiac patients with impaired ventricular function is common. Myoclonic jerking can be observed in the absence of muscle relaxation. The use in patients with normal ventricular function should be carefully considered because blunting of the adrenergic response to intubation is poor and may result in hypertension and tachycardia, particularly with the low-dose opioid techniques used today. Greater associations with postoperative nausea and vomiting are other potential adverse effects seen with etomidate administration.
The clinical effects of propofol are, in general, similar to those of thiopental. However, it has numerous advantages over thiopental based on its predictable pharmacokinetics and dynamics.276,277 It often is used for sedation after CABG surgery,278 although with the recent approval of dexmedetomidine for this indication,279 its use may decline. Its isolated effects on contractility are controversial, with conflicting findings depending on the model used. A sophisticated analysis of its effects in CABG patients using TEE assessment of preload-adjusted maximal power, a load-independent measure of contractility, at four different plasma concentrations (0.6 to 2.6 mg/mL) found no direct effect on contractility, although it lowered preload and afterload.280 It previously was evaluated in numerous clinical studies for induction and maintenance with an opioid (most commonly sufentanil) compared with a volatile-opioid combination for CABG in patients with normal and depressed EF. These studies reported minimal differences in hemodynamics or in the incidence of myocardial ischemia.281–286 However, more sophisticated and larger contemporary studies closely evaluating ventricular function on weaning from CPB and perioperative release of biomarkers of ischemia consistently reported better myocardial protective properties with the use of volatile agents over a total intravenous anesthetic technique with propofol. This is apparently related to anesthetic preconditioning and postconditioning effects of volatile agents (see Chapter 9). Propofol has been reported to have strong free radical scavenging properties that in one CABG study appeared to have attenuated myocardial lipid peroxidation in atrial tissue biopsies.287 In addition, propofol may have cardioprotective properties. The PRO-TECT II (PROpofol cardioproTECTion for type II diabetics) study is investigating whether high-dose propofol can confer cardioprotection to diabetics, a population that may not benefit as much from inhalation anesthetics.288
Benzodiazepines commonly are used in combination with a narcotic to induce anesthesia for CABG. In most settings, midazolam has replaced diazepam, given its numerous advantages (particularly water solubility, a shorter half-life, and absence of metabolites capable of accumulation, prolonging the sedative effects).289 Stanley et al290,291 and Liu et al292 were among the first to report on the addition of diazepam to high-dose morphine and, shortly thereafter, fentanyl anesthesia for CABG. They reported a mild-to-moderate reduction in CO (approximately 20% relative to opioid alone, with the greatest decrease in the fentanyl group). These studies suggested that diazepam should not be used, particularly in patients with impaired ventricular function. However, with the realization that breakthrough adrenergic responses, as well as a substantial incidence of anesthetic recall with fentanyl alone, can occur, supplementation with diazepam or midazolam quickly grew in popularity together with high-dose opioids in the early to mid-1980s.
Numerous clinical series of widely different sizes and designs, reporting on the efficacy of diazepam or, more commonly, midazolam used with high-dose opioids, were subsequently published.293–304 Moderate degrees of hypotension were reported in most studies, primarily attributed to a reduction in SVR (or from the effects of the high-dose opioid itself given the potent bradycardic effects of high-dose sufentanil). With rare exception, most investigators considered it safe and effective.305
There has been relatively little research on the direct cardiac effects of midazolam. Messina et al306 reported a clinical study of 40 CABG patients in whom 0.1 mg/kg midazolam was administered after induction and intubation with thiopental, fentanyl, and pancuronium. Contractility was depressed by midazolam, although afterload was reduced simultaneously, resulting in no net change in cardiac index. Patients with depressed baseline EF had lower indices of contractility at baseline but a similar magnitude of change. This study provided clinical confirmation of the safety of midazolam in clinical practice, particularly given its experimental design. Most clinicians have reduced dosing of midazolam to the range used in the latter study. Midazolam is used widely because of clinicians concerns regarding recall. However, with the use of continuous volatile anesthesia and availability of convenient neuromonitoring techniques, it should no longer be considered a necessity, particularly with use of small amounts as a component of the premedication.
High-dose opioid anesthesia was introduced into cardiac surgery by Lowenstein et al307 in 1969, in an attempt to provide safe anesthesia without myocardial depression in patients with severe valvular heart disease and compromised cardiac function. Although this revolutionized anesthesia for patients with cardiac dysfunction, it was apparent that morphine had several disadvantages: vasodilation from histamine release, increased requirements for fluids and vasoconstrictors, and prolonged respiratory depression. When morphine was given to patients with normal LV function, with most patients undergoing CABG particularly in the previous decades, dramatic hemodynamic responses occurred with surgical stress and amnesia could not be guaranteed; the anesthesia often was inadequate. Despite these well-known adverse effects, morphine has regained attention because of its possibly unique cardioprotective and anti-inflammatory properties.308,309
In the late 1970s, Stanley and collaborators first reported on the use of high doses of the synthetic opioid fentanyl for CABG, with and without supplemental benzodiazepines.310–312 Clinicians worldwide who perceived the lack of histamine release to be a favorable property rapidly adopted it into their clinical practice.313–319 However, it was recognized early on that recall still could be a problem.320 Reports on the use of the more potent sufentanil appeared at the same time as fentanyl, although most studies were not reported until the late 1980s.321–330 It, too, was widely adopted, although there was concern over its potent bradycardic effects at high dosages, particularly when administered with nonvagolytic muscle relaxants.331,332
In the mid-1990s, remifentanil was introduced. Fueled by intense interest in fast-tracking (being promoted in the same time frame), it has been intensively investigated.333–342 Careful planning with regard to when it is terminated and adequate continuation of pain control is required. The combination with neuraxial anesthetics also has been advocated.343 There have been reports of greater degrees of hypotension compared with fentanyl or sufentanil.344,345 Remifentanil infusion was found to attenuate the perioperative endocrine stress response as compared with fentanyl boluses as an adjunct to inhalation anesthesia.346
Based on computer modeling and clinical data, sufentanil appears to have the most favorable characteristics for continuous infusion with very predictable termination of effect.347,348 This characteristic was believed to be particularly important in the early 1990s when early postoperative suppression of ischemia by “intensive analgesia” was proposed as an important treatment goal just before the widespread interest in fast-tracking.349 The substantially greater costs of sufentanil (than fentanyl) and the apparent aversion of many cardiac anesthesiologists to use continuous anesthetic infusions have limited its use. Most evidence suggests that despite documented associations of sufentanil use with shorter times to extubation, overall costs and hospital lengths of stay are unaffected relative to fentanyl.350–352 Nonetheless, it remains popular with many clinicians, many of whom infuse it continuously during surgery.
These drugs are pure opioid agonists, and none provides complete anesthesia as defined by predictable dose–response relations for suppression of the stress response and release of endogenous catecholamines (particularly norepinephrine), even with high serum concentrations.353–355 Hypertension and tachycardia commonly have been reported in response to induction/intubation and surgical stimuli (particularly with sternotomy) in older studies of high-dose opioid anesthesia with fentanyl or sufentanil.356,357Figures 18-14 and 18-15 demonstrate this lack of association of serum levels with hemodynamic responses. The seminal study of Philbin et al358 (see Figure 18-15) investigated the relation between opioid dose and hemodynamic effect with fentanyl or sufentanil in a randomized study of CABG patients. Forty premedicated patients were allocated to receive fentanyl (50 or 100 μg/kg) or sufentanil (10, 20, or 30 μg/kg) in bolus dosing with 100% oxygen and the relaxant metocurine, with an additional 40 patients randomized to sufentanil bolus dosing (10, 20, or 40 μg/kg, followed by continuous infusions). Plasma opioid (only for sufentanil in the bolus/continuous infusion groups) and catecholamine concentrations were obtained after intubation and after sternotomy. A hemodynamic response was defined as a 15% or greater increase in systolic blood pressure (HRs were not reported), and a hormonal response was a 50% or greater increase from control. For both opioids, the frequency of hemodynamic responders (33% to 60%) was similar between all fentanyl and sufentanil bolus groups. In the sufentanil infusion groups, the plasma concentrations were consistent and dose related, with wide spreads between the groups. Twenty-four of the 40 patients were hemodynamic responders (with 18 occurring with sternotomy), and the frequency of response was unrelated to plasma sufentanil levels. Although this study had design flaws (e.g., small sample sizes and lack of power analyses, lack of reporting of actual hemodynamics), it is widely quoted to support the contention that even high-dose opioids alone provide incomplete anesthesia. Despite this, “narcotic anesthesia” was for many years considered synonymous with a “cardiac anesthetic” that should be used in all patients with CAD. It is only recently that this erroneous association is being laid to rest as volatile-based fast-tracking techniques have become standard of care in most patients undergoing myocardial revascularization.
The usual practice to provide complete anesthesia is to supplement opioids with inhaled or other intravenous agents. This permits a reduction in the total dose of opioid and, particularly with volatile agents, more rapid return of respiratory drive, facilitating early extubation. Thomson et al359 have incorporated this approach and extended the concepts investigated by Philbin and Roscow360 in a small but sophisticated study of CABG patients whereby specific effect site concentrations of fentanyl or sufentanil were targeted by using a computer-assisted infusion pump. Three targeted concentrations for each opioid were evaluated: sufentanil 0.4, 0.8, and 1.2 ng/mL and fentanyl 5, 10, and 15 ng/mL and the end-tidal concentration of isoflurane required to control MAP and HR in the prebypass period by predetermined criteria were monitored. The sufentanil subgroups required approximately 1.9, 3.1, and 4.9 μg/kg, and fentanyl subgroups received 18.8, 33.9, and 50.4 μg/kg in this period. The average end-tidal concentration of isoflurane was significantly greater between the low and medium/high groups, with no difference between medium and high groups. Most of the responses were increases in MAP (incidentally, the prime focus of Philbin and Roscow’s360 study). Regression analysis revealed significant correlations between serum opioid concentrations and isoflurane concentrations at most of the time points sampled in the pre-CPB period. By inspecting plots of the data pairs, they were able to ascertain the inflection point at which the isoflurane concentration began to increase rapidly, indicating poor control of hemodynamics by the respective opioid. For sufentanil, this was 0.71 ± 0.13 ng/mL, and for fentanyl, it was 7.3 ± 1.1 ng/mL. Given the use for fast-tracking with approximate ranges of 20 μg/kg for fentanyl and 3 μg/kg for sufentanil for the entire case, it is unlikely these concentrations will be obtained. However, reliance on volatile anesthesia, benzodiazepines, propofol, or dexmedetomidine infusion can provide hemodynamic control. The level of preexisting β-blockade and/or calcium channel-blockade and the type of muscle relaxant used influence each patient’s response.
Neuromuscular Blocking Agents
All of the available neuromuscular blocking agents have been used to produce adequate intubating conditions and relaxation during CABG surgery (Table 18-3). Murphy et al361 conducted a national survey of more than 400 active members of the Society of Cardiovascular Anesthesiologists evaluating their neuromuscular blocking drug of choice in the cardiac surgical setting. Despite availability of newer short-acting neuromuscular blocking agents, pancuronium was still used in most patients undergoing on-pump and off-pump cardiac surgical procedures 10 years ago. Traditionally, pancuronium had been advocated for use with high-dose narcotic techniques, because it offset opioid-induced bradycardia. However, it has long been recognized that clinically significant tachycardia resulting in myocardial ischemia could occur during induction of anesthesia with high-dose fentanyl and pancuronium.362 With the increasing popularity of fast-track cardiac surgery, early extubation is now most desirable, and the longer duration of action of pancuronium is a potential disadvantage. Several studies have compared the durations of action of pancuronium and rocuronium in patients undergoing cardiac surgery. Irrespective of a single intubating dose or a continuous infusion, patients receiving rocuronium had significantly less residual neuromuscular blockade363 and shorter time to extubation.364,365
When continuous infusions of rocuronium and cisatracurium were compared in prolonged surgical procedures, recovery to 75% of the train of four was faster in patients receiving cisatracurium.366 Reich et al367 compared neuromuscular transmission recovery times in pediatric cardiac patients receiving cisatracurium or vecuronium infusions in the ICU setting. Cisatracurium was associated with significantly faster spontaneous recovery of neuromuscular function. Especially in fast-track cardiac surgery, shorter-acting neuromuscular blocking agents such as cisatracurium or rocuronium are recommended to avoid residual paralysis and to allow for early extubation and ICU discharge. Neuromuscular transmission monitoring to assess for residual blockade and use of pharmacologic reversal is advisable, especially if a fast-track anesthesia technique is used.368,369 In patients with a potentially difficult airway or emergency patients who are not NPO, succinylcholine is still the agent of choice.
Additional considerations are warranted in patients with underlying comorbidities such as chronic renal failure, which may alter pharmacokinetics. When magnesium is administered to cardiac surgical patients for prophylaxis of perioperative arrhythmias, blockade from nondepolarizing neuromuscular blocking agents may be significantly prolonged.370 There remains the question whether continuous neuromuscular blockade for cardiac surgery really is necessary. Gueret et al371 showed that a single intubating dose of atracurium or cisatracurium provided adequate paralysis and surgical conditions leading to quicker neuromuscular blockade recovery in cardiac surgical patients. Advocates of this technique also point to potential advantages with regard to prevention of recall (as indicated by patient movement). However, potential disadvantages include the possibility of greater oxygen demand and consumption, or movement during surgery.
α2-Agonists: Dexmedetomidine
The intraoperative use of α2-agonists can be a useful adjunct, given their sedative, analgesic, and hemodynamic-stabilizing properties. α2-Agonists prevent hypertension and tachycardia during intubation, surgical stimulation, and emergence from anesthesia, and decrease plasma catecholamine levels.372–375 Jalonen et al,376 in a randomized, double-blind study, administered dexmedetomidine or placebo to CABG patients starting before induction (50 ng/kg/min for 30 minutes) and continued until the end of surgery (7 ng/kg/min). Patients receiving dexmedetomidine had significantly lower plasma norepinephrine levels and more stable hemodynamics (less increase in MAP and HR during induction, less intraoperative variability of systolic arterial pressure). Dexmedetomidine administration was associated with decreased incidences of intraoperative (5% vs. 32%) and postoperative (4% vs. 40%) tachycardia when compared with placebo. Patients who received dexmedetomidine also were less likely to receive β-blocker therapy for tachycardia. Despite the findings before and after CPB, a greater incidence of hypotension (MAP < 30 mm Hg) was seen during CPB (22% vs. 0% patients, dexmedetomidine vs. placebo). These data demonstrate that dexmedetomidine is effective in attenuating sympathetic responses, although this effect may predispose patients toward hypotension.
Because of the decreased oxygen demand and HR seen with dexmedetomidine administration, it may be beneficial during OPCAB. Nevertheless, in a small series of OPCAB patients, hypotension (12 mm Hg below baseline) occurred shortly after its administration during rewarming in the ICU, prompting the study authors to recommend volume loading and normothermia before its use.377 Experimental data demonstrated a decrease in the inflammatory response to endotoxin-induced shock in a rat model.378
Inhalation Anesthetics and Myocardial Protection
There is steadily increasing evidence from laboratory and clinical studies that inhalation anesthetic agents have favorable properties in patients undergoing CABG surgery, particularly in comparison with total intravenous anesthetic approaches. Concurrent with the now routine use of fast-track anesthesia techniques, there has been a major resurgence in their use as the primary anesthetic in patients undergoing cardiac surgery. Inhalation anesthetics are thought to protect the myocardium against ischemia by their ability to elicit protective cellular responses that are seen with ischemic preconditioning. The latter has been shown to reduce myocardial infarction size after periods of ischemia,379 protect the heart against postischemic LV dysfunction,380 and reduce the incidence of arrhythmias after cardiac surgery.381
Murry et al382 first showed that brief periods of ischemia before 40 minutes of coronary artery occlusion significantly reduced infarction size after myocardial reperfusion. Exposing the myocardium to short periods of ischemia followed by reperfusion is called ischemic preconditioning. These brief periods of ischemia initiate signaling pathways that render the myocardium resistant to subsequent prolonged periods of ischemia (i.e., memory effect).383 After a short ischemic period (i.e., preconditioning signal), the myocardium is rendered more resistant to prolonged ischemia when the subsequent ischemic event occurs within a certain time window. This may occur in two distinct phases as early preconditioning (about 2 hours) and delayed or late preconditioning (24 to 72 hours), although not all stimuli elicit both responses. Even though multiple approaches are used to protect the heart during CPB (e.g., blood cardioplegia, topical hypothermia, pharmacologic additives), aortic cross-clamping defines a period of myocardial ischemia that often results in transient or prolonged dysfunction after reperfusion.384,385 Brief periods of aortic cross-clamping with consequent reperfusion intervals before a period of ventricular fibrillation have been shown to preserve adenosine triphosphate (ATP) content, decrease markers of ischemia such as troponin I, and improve cardiac function in patients undergoing CABG.386–389 However, this approach is controversial and cumbersome because an ischemic episode may reduce cardiac reserves and exacerbate symptoms.390 In addition, the accomplishment of brief periods of ischemia by short cross-clamping of an atherosclerotic aorta may result in embolism formation and endothelial damage, thereby increasing mortality.391 Interestingly, this protection is now believed to be conferred even when the brief period of ischemia is applied to organs or tissues remote from the heart (remote preconditioning), thus improving its applicability.392–396
Pharmacologic interventions have been sought that mimic ischemic preconditioning. There is increasing evidence that aside from potent volatile anesthetics, opioids and, in particular, morphine trigger preconditioning.397,398 Other intravenous anesthetics such as etomidate and ketamine do not appear to have the same cardioprotective properties.399,400 Pharmacologic preconditioning and ischemic preconditioning initiate similar pathways that protect the myocardial cell against ischemic damage.
The pathways associated with myocardial preconditioning involve a variety of triggering stimuli, mediators, receptors, and effectors.401 It is thought that activation of sarcolemmal and mitochondrial KATP channels play a pivotal role in the preconditioning process.402 Opening of these channels protects the myocardium by preventing cytosolic and mitochondrial Ca2+ overload. The exact mechanisms of preconditioning are still actively under investigation. After the administration of a preconditioning signal such as ischemia, inhalation anesthetics including the noble gases, morphine, bradykinin, or nitroglycerin (NTG), membrane-bound receptors (adenosine A1, adrenergic, bradykinin, muscarinic, delta-1 opioid) coupled to inhibitory G proteins are activated.403–408 Consequently, products of intracellular transduction pathways (e.g., protein kinase C [PKC], tyrosine kinases, MAP kinases) mediate the opening and stabilization of ATP-sensitive mitochondrial KATP channels, the effectors thought to be mainly responsible for the preconditioning phenomenon.409,410 Increased formation of nitric oxide (NO),411,412 free oxygen radicals,413 and enzymes such as cyclooxygenase-2414 are also involved in the preconditioning process. The role of NO recently was confirmed by Smul et al using a rabbit model.415 The delayed phase of myocardial protection, which may last well beyond the documented 24 to 72 hours, probably is based on transcriptional changes of protective proteins,416,417 which may explain the gap of time between early and late preconditioning.418 Inhalation anesthetics also preserve cardiac function after reperfusion and decrease ischemia-induced intracoronary adhesion of polymorphonuclear neutrophils and platelets.419–423
Initially, most data were based on in vitro studies or from data obtained during PCIs. Whether these results could be generalized to cardiac surgery remained questionable. Multiple studies now have investigated anesthetic preconditioning in the cardiac surgery setting and its impact on outcome parameters. Belhomme et al424 exposed patients to 5 minutes of preconditioning with a 2.5 minimum alveolar concentration of isoflurane after the onset of CPB but before aortic cross-clamping. Troponin I and creatine kinase (CK)-MB levels were not significantly different from those of the control group. Nevertheless, patients who were exposed to isoflurane had increased activity of 5-nucleotidase, a marker for protein kinase C activation, which is early evidence of preconditioning pathway activation similar to the in vitro findings. Penta de Peppo et al425 reported on myocardial function and the preconditioning effects of enflurane in patients undergoing CABG surgery. Myocardial function was assessed using the end-systolic pressure-area relation, a relatively load-independent index of myocardial contractility. Although the number of patients studied was small, myocardial function was better preserved in patients who were exposed to enflurane before cardioplegic arrest.
Julier et al,426 in a double-blinded, placebo-controlled, multicenter study, reported on the effect of sevoflurane preconditioning on biochemical markers for myocardial and renal dysfunction in patients undergoing CABG surgery. Patients who had sevoflurane preconditioning during the first 10 minutes of CPB had lower levels of biochemical markers of myocardial and renal impairment. Brain natriuretic peptide level as an indicator of myocardial dysfunction was significantly decreased in the sevoflurane group. Conzen et al427 studied randomized patients undergoing OPCAB surgery with a propofol infusion versus a continuous inhalation-based anesthetic technique with sevoflurane. Patients in the sevoflurane group had significantly lower troponin I levels, as well as better LV function. Nader et al428 added vaporized sevoflurane (2%) versus oxygen alone to a cold blood cardioplegia solution in a small randomized trial of patients undergoing CABG surgery. Markers of the inflammatory response (i.e., neutrophil β-integrins, tumor necrosis factor-α, and interleukin-6) were lower, and cardiac function (i.e., stroke work index and wall motion analysis) was better preserved in the sevoflurane group (Figure 18-16). Table 18-4 summarizes the results of the randomized, prospective clinical studies.429–440
Whether these biochemical markers of improved cardiac outcome actually translate into reduced mortality or improved long-term outcome remains a matter of debate.441 Garcia et al435 reported on the results of a prospective, randomized study of the effect of sevoflurane preconditioning (10 minutes before aortic cross-clamping) on late cardiac events. Coronary artery reocclusion, congestive heart failure, and cardiac death were assessed at 6 and 12 months after surgery. Preconditioning with sevoflurane significantly reduced the incidence of late cardiac events. In a prospective, randomized, nonblinded study, De Hert et al442 compared the inhalation anesthetics desflurane and sevoflurane with an intravenous anesthetic technique with a continuous propofol infusion in high-risk patients undergoing CABG surgery. In the volatile anesthetic group, myocardial function was better preserved, troponin I levels were lower, and ICU and hospital LOS were shorter compared with propofol or midazolam-based anesthetic regimens in this setting.443 Guarrancino et al444 showed that a desflurane-based anesthetic resulted in reduced troponin I release, inotropic drug use, and number of patients requiring prolonged hospitalization compared with a propofol-based technique in off-pump CABG.
Three recent meta-analyses examined preconditioning and mortality or long-term outcome in patients undergoing cardiac surgery. In a meta-analysis that included only studies with sevoflurane and desflurane, Landoni et al445 showed a reduction in mortality and the incidence of myocardial infarction after cardiac surgery. In two other meta-analyses that also included isoflurane, no such benefit was seen.446,447 Data from a retrospective Danish database multicenter analysis including 10,535 patients did not show any difference in overall postoperative mortality or myocardial infarction between propofol and sevoflurane-based anesthesia.448 To further investigate these controversial findings, Bignami et al449 conducted a longitudinal survey among 64 Italian cardiac surgical centers to study the correlation between the use of volatile anesthetics and 30-day mortality in CABG surgery. The results showed that risk-adjusted 30-day mortality was significantly reduced when volatile agents were used during cardiac surgery, especially when there was prolonged use of these agents. Interestingly, the most consistent results were found when isoflurane was used. In a recent multicenter trial comparing desflurane or sevoflurane with propofol-based anesthesia, De Hert et al450 were unable to find a difference in troponin release but did show that the use of inhalation anesthetics reduced hospital LOS and patients in the inhalation anesthetic group had a lower 1-year mortality.
The optimal timing and duration of inhalation anesthetic administration are still under investigation.451–454 Whereas some of the studies use brief periods of anesthetic preconditioning before aortic cross-clamping, others have reported on use of volatile anesthetics throughout the operative period. The older studies using brief periods of preconditioning showed improved cardiac function, although markers of myocardial injury such as CK-MB or troponin I often were not significantly different from the control group. De Hert et al455 showed that the best results for myocardial protection were achieved when sevoflurane was administered throughout the intraoperative period and not just immediately before the planned myocardial ischemic event. Most similarly designed studies have confirmed those findings. Bein et al,456 however, found that myocardial cell damage and dysfunction were lower in patients who received sevoflurane in an interrupted manner. Frassdorf et al457 also demonstrated that preconditioning-related myocardial protection was superior with multiple periods of sevoflurane administration applied rather than one short period. When sevoflurane was added to the anesthesia regimen after the coronary anastomoses were completed, myocardial recovery was faster compared with a propofol-based anesthetic technique. Nevertheless, patients who received sevoflurane during the entire procedure had the lowest troponin I levels, and the stroke volume changed the least compared with baseline levels.455 Most data available to date suggest not limiting the use of inhalation anesthetics to brief periods, but rather to use prolonged administration.
Research on pharmacologic preconditioning is not restricted only to inhalation anesthetics. There is increasing evidence that a variety of drugs that are administered perioperatively have cardioprotective properties involving preconditioning pathways. Besides inhalation anesthetics, opioids (delta-opioid receptor), adenosine (adenosine A1 receptor), and bradykinin have been investigated for their preconditioning effects, with variable results.458–461
During cardiac surgery, several of the known preconditioning triggering agents may be used and appear to be additive or synergistic in effect. Toller et al462 reported that the administration of sevoflurane and mechanical ischemic preconditioning reduced infarction size significantly compared with either stimulus alone. Ludwig et al463 demonstrated the additive effect of isoflurane and morphine on the reduction of infarction size. There are still insufficient data on how the potential beneficial effect of potent inhalation anesthetics differs between OPCAB and on-pump CABG patients, with some preliminary data that OPCAB patients may benefit more.464
Several clinical factors appear to impair the protective effects of preconditioning. Two groups of patients, diabetic and female patients, appear to have attenuated responses to mechanical preconditioning signals (during PCI).465 Intraoperative hyperglycemia blocks the preconditioning effect, although this effect may be reversed by N-acetylcysteine, an oxygen radical scavenger.466–470
The role of postconditioning is now also starting to be elucidated. Prompt treatment of myocardial ischemia is essential for limiting myocardial damage. However, the damage to the myocardium is not only a result of the ischemic time but of the reperfusion itself. Brief episodes of ischemia/reperfusion applied during the first few minutes of reperfusion after a prolonged ischemia have been shown to decrease myocardial infarct size to the same extent as that induced by ischemic preconditioning.471,472 It is thought that mitochondrial KATP channels, NO, and protein kinase C play important roles in postconditioning as well.473 Protein kinase C is one of a larger group of kinases labeled “reperfusion injury salvage kinases” that play a role in postconditioning.474–476 The beneficial effects of ischemic postconditioning also have been shown in humans during coronary angioplasty for acute myocardial infarction.477,478 As in preconditioning, postconditioning also has been found to work when applied remotely.479 Inhalation anesthetics also may be effective in blunting the deleterious effects of postischemic reperfusion injury and the inflammatory response syndrome after cardiac surgery.480,481 Thus far, most data about anesthetic postconditioning have been gathered from studies in animals and in vitro isolated human myocardium. Postconditioning has been shown to improve contractile function482 and attenuate postischemic arrhythmias.483 Recent data further suggest that the protective properties of potent inhalation anesthetics may not be restricted only to the myocardium but extend to other organ systems.484–487
In summary, there is increasing evidence suggesting that potent inhalation anesthetics should be part of the anesthetic regimen in patients undergoing cardiac surgery, particularly in patients undergoing CABG, OPCAB, and/or patients at high risk for ischemic events.488
Intraoperative Awareness and Recall
Intraoperative awareness (i.e., recall), usually defined as postoperative memory for intraoperative events, is an infrequent but well-recognized phenomenon during general anesthesia. Awareness, a conscious subjective experience (i.e., implicit memory), may be much more frequent than conscious recall (i.e., explicit memory) of intraoperative events.489 In a prospective, nonrandomized, descriptive cohort study of more than 19,000 patients undergoing surgery with general anesthesia at seven academic medical centers in the United States, awareness with explicit recall occurred in 0.13% of the cases.490 Patients undergoing cardiac surgery always have been considered to be at increased risk because of anesthetic regimens intentionally devoid of cardiodepressant inhalation anesthetics (before the era of improved myocardial preservation and fast-tracking) and because of frequent periods of light anesthesia in the presence of hemodynamic instability resulting from surgical manipulation of the heart and great vessels, depressed contractility after CPB, or bleeding. The published incidence rate of awareness in patients undergoing cardiac surgery is significantly greater than that reported for general surgery, with older reports of up to 23%.491 However, the introduction of fast-track anesthesia techniques and the more frequent use of inhalation agents have helped to reduce the risk for intraoperative awareness for this type of surgery. Ranta et al492 found definite intraoperative awareness with recall in 0.5% of patients undergoing cardiac surgery under general anesthesia, with various anesthesia regimens using inhalation or total intravenous anesthetic techniques. Greater doses of benzodiazepines, such as midazolam, resulted in a lower incidence of awareness. Phillips et al493 interviewed 700 patients after cardiac surgery with CPB and found that 8 patients (1.8%) reported intraoperative recall regardless of whether benzodiazepines or inhalation agents were used. Fast-track anesthesia techniques typically combine low-dose narcotics with short-acting anesthetics such as inhalation agents or propofol infusions. Dowd et al494 reported a low incidence rate (0.3%) of intraoperative awareness with this technique, attributing it to the continuous administration of volatile anesthetics or propofol.
Measures to prevent intraoperative awareness typically are based on anesthetic regimens that interfere with memory processing, such as the use of inhalation anesthetics and benzodiazepines. However, the anesthesia technique does not always correlate with the incidence of intraoperative awareness. In a large series of more than 11,000 patients undergoing general anesthesia, 18 cases of recall occurred (0.18%), but the analysis suggested that this number could not have been reduced with monitoring of end-tidal anesthetic gas concentrations or more frequent use of preoperatively administered benzodiazepines.495 Efforts to determine time periods that are prone to awareness or recall usually are based on physiologic or hemodynamic parameters that indicate light anesthesia, such as tachycardia, arterial hypertension, or patient movement. Nevertheless, Kerssens et al496 showed that hemodynamic variables were poor predictors of intraoperative awareness, although these parameters were able to differentiate between patients with or without conscious recall. In contrast, electroencephalogram-derived parameters were highly significant predictors of intraoperative awareness but were not able to detect patients with conscious recall.
Consequently, if hemodynamic parameters or measures of anesthetic concentrations are insufficient in preventing intraoperative awareness, the question arises whether monitoring neurophysiologic parameters such as processed electroencephalographic data decreases the incidence of intraoperative awareness or recall (see Chapter 16). Neurophysiologic monitoring is usually based on fast Fourier transformation and bispectral analysis of one-channel electroencephalographic data obtained from electrodes on the patient’s forehead. Several devices have been approved by the U.S. Food and Drug Administration (FDA).497–500 Two large studies showed a significant reduction in awareness under general anesthesia when the Bispectral Index (BIS) was monitored. Myles et al501 studied 2463 patients considered to be at high risk for awareness in a prospective, randomized, double-blinded, multicenter trial with 45% undergoing high-risk cardiac surgery or OPCAB. Patients were randomized to BIS-guided or routine anesthesia care. BIS monitoring reduced the risk rate for awareness by 82%, although the absolute number of confirmed events was small (2 vs. 11). In patients undergoing cardiac surgery, three events occurred (two routine, one BIS). “Possible” awareness events (not confirmed by the end-point committee) occurred irrespective of the use of BIS monitoring. Ekman et al,502 in a large, nonrandomized, historically controlled study, also showed a significantly reduced incidence rate of intraoperative awareness (0.04% vs. 0.18%) in the BIS monitored patients. However, in a small series of patients, Barr et al503 found no correlation between bispectral electroencephalographic analysis of anesthesia depth and conscious recall in patients undergoing cardiac surgery. This was attributed to exclusive use of midazolam and fentanyl.
Besides monitoring anesthetic depth, neurophysiologic monitoring also may help to decrease the incidence of hemodynamic disturbances, may guide titration of anesthetic agents to their effective dose, and may be associated with improved patient satisfaction.504,505 In clinical experience, the use of BIS monitoring has been helpful in more accurately gauging the need for reinstitution of volatile anesthesia after weaning from CPB. In this potentially unstable period with a depressed myocardium or untoward responses to protamine, this can be an important factor. Often the BIS remains in the low range for the first 15 to 30 minutes after weaning, especially in older patients with longer pump times. It also is helpful to ensure adequacy of burst suppression for hypothermic circulatory arrest.
In summary, published data appear to indicate that despite a substantial reduction in awareness resulting from a shift to greater use of volatile anesthetics, patients undergoing cardiac surgery are still at risk for intraoperative awareness or recall. Neurophysiologic monitoring decreases the incidence of these events, but given the low incidence, a large number of patients have to be monitored to prevent one case of intraoperative awareness. The implications of intraoperative awareness or conscious recall are not yet fully understood. These events may be unrecognized or not necessarily experienced as unpleasant,506 but they also may lead to severe post-traumatic stress disorders with chronic health impairment.507

Myocardial Ischemia in Patients Undergoing Revascularization Surgery
Incidence
In addition to providing anesthesia, a major concern of the anesthesiologist is the prevention and treatment of myocardial ischemia. Numerous laboratory and clinical studies have examined the incidence of myocardial ischemia in the perioperative period, as it relates to the administration of anesthetic drugs. Although the incidence rate of prebypass ischemia in patients appears to be between 10% and 50%, it is not at all clear that the anesthetic drug combination per se is a determinant of this incidence. Several studies have addressed whether the anesthetic technique (e.g., fast-track or early extubation, high thoracic epidural) is related to perioperative morbidity and myocardial ischemic events. An extensive meta-analysis of more than 30 randomized, controlled trials of patients undergoing cardiac surgery showed no difference in the relative risk of postoperative myocardial ischemia when early extubation protocols were compared with conventional extubation management.508
Hemodynamic Changes Related to Myocardial Ischemia
Besides ECG abnormalities, some hemodynamic changes should alert the anesthesiologist to the possibility of intraoperative myocardial ischemia. The association of tachycardia with hypotension or increased LV filling pressure, both of which reduce coronary perfusion pressure [CPP], is a particularly undesirable combination, jeopardizing the oxygen supply-demand relation. Figure 18-17 demonstrates how hypertension in the absence of tachycardia, in response to surgical stress (skin incision), can be associated with pulmonary hypertension, increased PCWP, and prominent A and V waves on the PCWP waveform.509 Although ECG changes occurred later, the early hemodynamic abnormalities almost certainly were the result of ischemic LV dysfunction. Treatment included deepening anesthesia and administering an NTG infusion.
LV diastolic dysfunction detected with TEE is one of the earliest changes identified after coronary artery occlusion, and it often precedes the development of abnormal systolic function. RWMAs also have been described as early signs of ischemia. They occur within seconds of inadequate blood flow or oxygen supply. RWMAs detected by TEE have been shown to be a more sensitive method of detecting myocardial ischemia in patients undergoing CABG, compared with ST-segment changes.510 Myocardial ischemia or repositioning the heart during OPCAB can be the cause of a sudden onset of mitral regurgitation or worsening of preexisting mitral regurgitation, both of which can be detected with TEE monitoring (see Chapter 12).
Intraoperative Treatment of Myocardial Ischemia
Close attention to hemodynamic control and rapid treatment of abnormalities are fundamental principles of the intraoperative management of the patient with CAD. If there is a hemodynamic abnormality that is temporally related to the onset of ischemia, it should be treated accordingly. Hemodynamic treatment to ensure an adequate CPP (diastolic blood pressure minus left ventricular end-diastolic pressure [LVEDP]) should be a priority, as should control of HR, the single most important treatable determinant of myocardial oxygen consumption. Table 18-5 summarizes the treatment of acute perioperative myocardial ischemia. The earliest objective evidence of successful treatment of ischemia may be the return to normal of the PAP and the PCWP waveforms. In the following section, some of the routinely used pharmacologic interventions for intraoperative myocardial ischemia are discussed.
TABLE 18-5 Acute Treatments for Suspected Intraoperative Myocardial Ischemia
Associated Hemodynamic Finding | Therapy | Dosage |
---|---|---|
Hypertension, tachycardia* | Deepen anesthesia | |
IV β-blockade | Esmolol, 20–100 mg, +50–200 μg/kg/min PRN | |
Metoprolol, 0.5–2.5 mg | ||
Labetalol, 2.5–10 mg | ||
IV nitroglycerin | Nitroglycerin, 33–330 μg/min† | |
Normotension, tachycardia* | Ensure adequate anesthesia, change anesthetic regimen | |
IV β-blockade | β-blockade, as above | |
Hypertension, normal heart rate | Deepen anesthesia | |
IV nitroglycerin or nicardipine | Nicardipine, 1–5 mg, +1–10 μg/kg/min | |
Nitroglycerin, as above | ||
Hypotension, tachycardia* | IV α-agonist | Phenylephrine, 25–100 μg |
Norepinephrine, 2–4 μg | ||
Alter anesthetic regimen (e.g., lighten) | ||
IV nitroglycerin when normotensive | Nitroglycerin, as above | |
Hypotension, bradycardia | Lighten anesthesia | |
IV ephedrine | Ephedrine, 5–10 mg | |
IV epinephrine | Epinephrine, 4–8 μg | |
IV atropine | Atropine, 0.3–0.6 mg | |
IV nitroglycerin when normotensive | Nitroglycerin, as above | |
Hypotension, normal heart rate | IV α-agonist/ephedrine | α-Agonist, as above |
IV epinephrine | Epinephrine, as above | |
Alter anesthesia (e.g., lighten) | ||
IV nitroglycerin when normotensive | Nitroglycerin, as above | |
No abnormality | IV nitroglycerin | Nitroglycerin, as above |
IV nicardipine | Nicardipine, as above |
Ensure adequacy of oxygenation, ventilation, and intravascular volume status, and consider surgical factors, such as manipulation of heart or coronary grafts.
IV, intravenous; PRN, as needed.
* Tachyarrhythmias (e.g., paroxysmal atrial tachycardia, atrial fibrillation) should be treated directly with synchronized cardioversion or specific pharmacologic agents.
† Bolus doses (25–50 μg) and high infusion rate may be required initially.
Intravenous Nitroglycerin
Since the introduction in 1976 by Kaplan et al511 of the V5 lead to diagnose myocardial ischemia, and intravenous NTG to treat it,512 NTG has been one of the mainstays in the treatment of perioperative myocardial ischemia. Intravenous NTG acts immediately to reduce LV preload and wall tension, primarily by decreasing venous tone in lower doses. In larger doses, it also may decrease arterial resistance and epicardial coronary arterial resistance.513,514 NTG has been shown to consistently decrease LV filling pressures, systemic BP, and myocardial oxygen consumption and to improve LV performance in patients with severe dysfunction.515 It is most effective in treating acute myocardial ischemia with ventricular dysfunction accompanied by sudden increases in LVEDV, LVEDP, and PAP. These increases in LV preload and wall tension further exacerbate perfusion deficits to the ischemic subendocardium and usually respond immediately to NTG (Figure 18-18).
Before surgery, NTG often is used to treat patients with unstable angina or ischemic mitral regurgitation and limit the size of an evolving myocardial infarction, reduce associated complications, and reverse RWMAs.516,517 In the pre-CPB period and during OPCAB, NTG is used to treat signs of ischemia such as ST-segment depression, hypertension uncontrolled by the anesthetic technique, ventricular dysfunction, or coronary artery spasm (Box 18-5). During CPB, NTG can be used to control the MAP with a time to onset of effect ranging from 4.1 ± 0.8 to 7.8 ± 2.8 minutes at doses of 1.7 ± 0.3 to 2.9 ± 0.7 μg/kg/min.518 However, NTG is not always effective in controlling MAP during CPB (approximately 60% of patients respond) because of alterations of the pharmacokinetics and pharmacodynamics of the drug with CPB. Factors contributing to the reduction of its effectiveness include adsorption to the plastic in the CPB system, alterations in regional blood flow, hemodilution, and hypothermia. Booth et al519 have shown that different oxygenators and filters sequester up to 90% of circulating NTG during CPB. After revascularization, NTG is used to treat residual ischemia or coronary artery spasm and reduce preload and afterload. It may be combined with vasopressors (e.g., phenylephrine) to increase the CPP when treating coronary air embolism (Box 18-6).
Studies of the prophylactic role of NTG in preventing perioperative myocardial ischemia have shown mixed results, with most studies showing no effects of NTG infusions on the incidence of perioperative myocardial ischemic events.520–522 In a prospective, double-blinded, placebo-controlled study, Zvara et al523 randomized patients undergoing CABG surgery using a fast-track anesthesia technique to 2 μg/kg/min NTG or placebo starting before induction and continuing until 6 hours after extubation in the ICU. They found a similar incidence, severity of symptoms, and duration of myocardial ischemia, regardless of whether the patients received NTG or placebo (37% vs. 35%, respectively). Even though there were more patients in the placebo group with positive enzymes and ECG signs of myocardial infarction, these findings were not statistically significant. Similarly, in a prospective, randomized, controlled study, 0.5 to 1 μg/kg/min NTG administered after aortic cross-clamp release in patients undergoing CABG surgery did not decrease the incidence of postoperative myocardial ischemia.524
Intravenous NTG has been compared with other vasodilators such as nitroprusside and the calcium channel blockers during CABG and in other clinical situations. Kaplan and Jones525 demonstrated that NTG was preferable to nitroprusside during CABG. Both drugs were shown to control intraoperative hypertension and to decrease myocardial oxygen consumption; however, NTG improved ischemic changes on the ECG, but nitroprusside did not. The lack of improvement in the ischemic ST segments with nitroprusside was thought to result from a decrease in CPP or the production of an intracoronary steal. When NTG was compared with calcium channel blockers in patients undergoing CABG surgery, the results depended on the class of calcium channel blocker, the usage of arterial conduits, and the administered doses.
Calcium Channel Antagonists
The calcium antagonists are a structurally diverse group of drugs that inhibit the passage of calcium through the slow channels of the cell membrane. These agents collectively relax arterial smooth muscle, with little effect on most venous beds. Despite areas of commonality, however, the calcium antagonists differ in their actions and hemodynamic effects. For example, nifedipine acts primarily on vascular smooth muscle, with little effect on the atrioventricular node. In contrast, verapamil acts mainly on the cardiac conduction system and has less effect on vascular smooth muscle and the myocardium. Neither diltiazem nor verapamil has been found to significantly increase CBF or to consistently decrease coronary vascular resistance. Verapamil and diltiazem have been shown to produce significant hemodynamic changes with myocardial depression and conduction disturbances during anesthesia.526,527 This limits their use in the treatment of perioperative myocardial ischemia. Nevertheless, the perioperative use of nicardipine and recent studies with clevidipine showing a reduced incidence of cardiac events in patients who received calcium antagonists during cardiac surgery have led to an increased interest in this diverse group of drugs in patients presenting for CABG or OPCAB surgery.528,529
Calcium antagonists have been found to be cardioprotective against reperfusion injury. This is due to their energy-saving actions of negative inotropy and chronotropy. These antagonists also have been shown to reduce reperfusion arrhythmias and attenuate myocardial stunning. In a review of methods to reduce ischemia during OPCAB, Kwak530 included the calcium antagonists along with newer drugs such as NO-releasing agents, free radical scavengers, and Na+/H+ exchange inhibitors in the management of ischemia/reperfusion injury.
Nicardipine is a short-acting dihydropyridine calcium antagonist similar to nifedipine, but possessing a tertiary amine structure in the ester side chain. Nicardipine is stable as a parenteral solution and, therefore, can be administered intravenously.531 It has highly specific modes of action, which include coronary antispasmodic and vasodilatory effects and systemic vasodilation. Among the calcium antagonists, nicardipine is unique in its consistent augmentation of CBF and its ability to induce potent and more selective vasodilator responses in the coronary bed than in the systemic vascular bed. Other important hemodynamic effects include reductions in BP and SVR, and increases in myocardial contractility and CO.532,533 Nicardipine also produces minimal myocardial depression and significant improvement in diastolic function in patients with ischemic heart disease.534,535 Intravenous doses of 5 to 10 mg of nicardipine administered to patients with CAD produce therapeutic plasma levels. Plasma concentrations decline in a biphasic manner, with an initial half-life of 14 minutes and a terminal half-life of 4.75 hours.536 Clearance of nicardipine results mainly from its metabolism by the liver, and excretion is primarily through bile and the feces. It undergoes rapid and extensive first-pass hepatic metabolism with the production of inactive metabolites.
The rapid onset and cessation of action of nicardipine make it an attractive drug for the perioperative management of hypertension or myocardial ischemia.537 It has been administered to control hemodynamics during and after vascular surgery and CABG. Begon et al538 demonstrated that 5 mg of nicardipine was effective in treating intraoperative hypertension. The MAP decreased by 35%, with the peak onset of action in 6 minutes and duration of action of 45 minutes. Van Wezel et al539 used a 3 to 12 μg/kg/min infusion of nicardipine and compared it with a 1 to 3 μg/kg/min infusion of nitroprusside. Both drugs were found to be equally effective in controlling BP; however, there was a 24% incidence rate of ST-segment depression in the nitroprusside group versus 9% in the nicardipine group. Van Wezel et al540 also compared intravenous NTG with intravenous administration of verapamil or nifedipine in patients undergoing CABG. NTG was found to be the drug of choice because it controlled BP while not producing as much tachycardia (as nifedipine) or myocardial depression and conduction blockade (seen with verapamil). Apostolidou et al541 randomized patients undergoing CABG surgery with CPB to nicardipine (0.7 to 1.4 μg/kg/min), NTG (0.5 to 1 μg/kg/min), or placebo. Immediately after coronary revascularization (after aortic cross-clamp release until end of surgery), there were significantly fewer episodes of myocardial ischemia in the nicardipine group (0% vs. 10% vs. 24%, respectively). During the following postoperative period, there was no difference regarding myocardial ischemia among the drug groups. Apart from preventing myocardial ischemia, nicardipine effectively controls arterial hypertension in the postoperative period.542,543 From these studies, nicardipine appears to offer significant advantages over other drugs such as nitroprusside in the intraoperative and postoperative management of hypertension and myocardial ischemia after CABG surgery.
More recently, clevidipine has been introduced for the treatment of perioperative hypertension.544 Clevidipine is an ultrashort-acting intravenously administered dihydropyridine calcium channel blocker. Clevidipine acts as an arterial-selective vasodilator, and its action is rapidly terminated by blood and tissue esterases. In a randomized, double-blinded, placebo-controlled multicenter trial in patients undergoing cardiac surgery, clevidipine effectively reduced arterial blood pressure.545 The Evaluation of Clevidipine In the Perioperative Treatment of Hypertension (ECLIPSE) trial compared clevidipine with NTG, sodium nitroprusside, and nicardipine for acute hypertension treatment in cardiac surgery patients.546 Clevidipine effectively maintained the arterial blood pressure within a prespecified range. Compared with nitroprusside, clevidipine-treated patients had a significantly reduced mortality (P = 0.04).
Esmolol
Hypertension, tachycardia, arrhythmias, and myocardial ischemia from sympathetic stimulation are common occurrences in the perioperative period. Despite the benefits of early use of β-blockers in the treatment of myocardial ischemia, the relatively long half-life and prolonged duration of action of previously available β-blockers have limited their usefulness during surgery and the immediate postoperative period.547 The introduction of esmolol, an ultrashort-acting cardioselective β1-blocker with a half-life of 9 minutes because of rapid esterase metabolism, provides a β-blocker that is extremely useful in the perioperative period. Esmolol has been shown to be effective in treating patients with acute unstable angina or during acute coronary occlusion. A mean esmolol dose of 17 ± 16 mg/min, with a range of 8 to 24 mg/min, was found to be effective in alleviating chest pain while increasing CO in patients with unstable angina.548 LV diastolic function was shown to improve with β-blockade. Kirshenbaum et al549 showed that esmolol was effective in treating acute myocardial ischemia even in patients with poor LV function (increased PCWP of 15 to 25 mm Hg). Esmolol was infused in these patients in doses up to 300 μg/kg/min and produced decreases in HR, BP, and CI. However, the PCWP was not significantly altered by the drug infusion. These results suggested that even in the presence of moderate LV dysfunction, esmolol can safely reduce BP and HR in patients with acute myocardial ischemia. During percutaneous transluminal coronary angioplasty, esmolol also was found to reduce the amount of ST-segment elevation and the onset of RWMAs.550 Esmolol has been used during CABG in a prophylactic manner to prevent hypertension, tachycardia, and myocardial ischemia.551 Before the introduction of newer stabilizing mechanical devices, it had been used frequently during OPCAB procedures to slow the HR during the surgical procedure. Esmolol also has been used to treat intraoperative hypertension, tachycardia, and myocardial ischemia. Bolus doses of 1.5 mg/kg have been found to be effective in treating ST-segment changes in patients with CAD.552 More commonly, a smaller bolus dose is used and is combined with an infusion of esmolol. Bolus doses ranging from 0.5 to 1.0 mg/kg have been used, followed by infusions of 50 to 300 μg/kg/min.553 These doses have been found to effectively treat increases in HR that occur during surgery and to block the β-adrenergic effects of catecholamines associated with surgical stress. In patients with poor LV function, doses as small as 20 mg have been found to be effective.

Weaning Patients from Cardiopulmonary Bypass after Coronary Revascularization
Chapter 32 provides a detailed discussion about the various predictors and techniques of weaning a patient successfully from CPB. Some factors and concerns are more specific to patients undergoing myocardial revascularization. Obviously, a good surgical technique, not only in the quality of anastomoses, but in preserving the heart during aortic cross-clamping, is key to cardiac function immediately after separation from CPB. The administration of cardioplegia can be problematic in patients with CAD, and various techniques are used including a combination of antegrade and retrograde cardioplegia. In patients with normal preoperative function, minimal, if any, inotropic support is usually required. In patients with impaired ventricular function, TEE evaluation immediately before weaning off CPB can provide invaluable information in choosing an inotropic or vasoconstrictive agent. In patients who present to the operating room with an IABP inserted, this support typically is continued into the postoperative period. In patients with poor ventricular function, the insertion of an IABP to support ventricular function during weaning from CPB can be helpful.554,555

Immediate Postoperative Period
Sedation
α2-Adrenergic receptor agonists have unique properties (Box 18-7) that explain their increasing use in cardiac surgical patients. Although the FDA approved clonidine in 1974, it was available only as an oral formulation in the United States, limiting its widespread use. In 1999, the FDA approved dexmedetomidine for continuous (up to 24 hours) intravenous sedation in the ICU setting. It is seeing increasing use in the operating room and ICU settings. Dexmedetomidine is a more selective α2-adrenoceptor agonist than clonidine (approximately eight times greater). It exhibits both central sympatholytic and peripheral vasoconstrictive effects. Intravenous infusion of dexmedetomidine (0.2 to 0.7 μg/kg/hr) causes transient increases of MAP and SVR because of stimulation of α- and β2-adrenergic receptors in vascular smooth muscle. This is followed by decreases in MAP, HR, SV, CO, and plasma catecholamine levels.556,557 Greater doses of dexmedetomidine result in more profound sedation and analgesia with a persistent increase in MAP, SVR, and PAP.558
There are limited data from animal studies demonstrating potential coronary vasoconstrictive and cardiodepressant effects.559–562 However, coronary vasoconstriction was seen mainly with bolus doses of 10 μg/kg and greater. At the recommended loading doses (0.5 to 2 μg/kg) and maintenance (0.2 to 0.7 μg/kg/hr), dexmedetomidine most likely has a favorable effect on myocardial perfusion.563 Roekaerts et al564,565 showed an increase in endocardial-to-epicardial blood flow ratio in the postischemic myocardium, with an overall reduction of myocardial oxygen demand after experimentally induced myocardial ischemia in dogs. As expected, the greatest reduction in oxygen demand is seen when baseline HR and arterial BP are increased. Intravenous dexmedetomidine exhibits a rapid distribution phase with a distribution half-life of about 6 minutes. Dexmedetomidine shows high protein binding (94%) and undergoes extensive biotransformation in the liver with direct glucuronidation and cytochrome P450–mediated metabolism. Although dexmedetomidine mainly is excreted renally (95%), no dose adjustments are necessary in patients with renal insufficiency, although slightly prolonged sedation should be expected.566 Severe hepatic impairment may necessitate a dose reduction.
Dexmedetomidine may be a useful agent in the early postoperative period because its sedative properties are associated with minimal respiratory depression and, in this regard, appear to mimic natural sleep patterns.567 When administered continuously in postsurgical patients, it caused no statistical differences in respiratory rate, oxygen saturation, arterial pH, and arterial carbon dioxide tension when compared with placebo.568 These patients usually were effectively sedated but still arousable and cooperative to verbal stimulation.569 Because of its analgesic properties, it significantly reduced additional opioid analgesia requirements in mechanically ventilated patients in the ICU.570–572 The α2-agonists have been used successfully in patients with postoperative delirium and withdrawal symptoms in alcohol or drug addicts, and they are associated with a low rate of shivering.573,574
Propofol has been used extensively intraoperatively, as well as for sedation in the ICU. Several studies compared propofol and dexmedetomidine in the postoperative period after surgery. Venn et al575 randomized a small number of patients to propofol versus dexmedetomidine sedation in the immediate postoperative period. Dexmedetomidine reduced the requirement for opioid analgesia, but importantly for patients after myocardial revascularization, it reduced HR more than propofol, whereas the arterial blood pressure did not differ between the two groups. In a multicenter, randomized study, Herr et al576 compared a dexmedetomidine-based sedation regimen with propofol sedation after CABG in the ICU. Although there were no differences in time to extubation, the investigators found a significantly reduced need for additional analgesics (i.e., propofol-sedated patients required four times the mean dose of morphine), antiemetics and diuretics and had fewer episodes of tachyarrhythmias requiring β-blockade (ventricular tachycardia in 5% of the propofol-sedated group vs. none in the dexmedetomidine group). In this study, however, hypotension was more common in the dexmedetomidine group compared with the propofol-sedated patients (24% vs. 16%; Figure 18-19). Approximately 25% of the dexmedetomidine-associated hypotension occurred in the first hour of the study (starting at sternal closure), particularly during or within 10 minutes after the loading infusion of 1 μg/kg. The combination of reduction of preload during sternal closure and the loading dose would seem to indicate this was not the optimal manner in which to use this agent. Loading doses are infrequently administered in today’s clinical practice to avoid hypotension seen with a large loading dose of dexmedetomidine, but a continuous maintenance dose started earlier to achieve appropriate plasma levels at the time of patient transfer from the operating room.
With regard to patient satisfaction, insufficient data are available to clearly favor one of the two agents. Corbett et al577 randomized 89 adult patients after CABG surgery to either dexmedetomidine or propofol. Patients were interviewed regarding awareness, recall, generalized comfort, level of pain, ability to interact with healthcare providers and family, feelings of agitation and anxiety, ability to sleep and rest, and overall satisfaction with ICU stay. The level of awareness and additional morphine and midazolam requirements did not differ between the groups. Patients favored propofol to sleep and rest, there were more patient discomfort and pain in the dexmedetomidine group, and the authors concluded that dexmedetomidine did not offer any advantages over propofol for short-term sedation after CABG surgery. The increased incidence of pain in the dexmedetomidine group is surprising, and most studies show reduced opioid requirements with α2-adrenoceptor agonists. Barletta et al578 compared propofol and dexmedetomidine after CABG and/or valve surgery in a fast-track recovery room setting. Patients (n = 100) were matched according to surgery type and left ventricular function. Dexmedetomidine resulted in lower opioid requirements, but this did not result in shorter duration of mechanical ventilation, improved quality of sedation, or rate of adverse events.
Coronary Artery and Arterial Conduit Spasm
Since 1981, when Buxton et al579 first reported coronary artery spasm immediately after CABG, there have been numerous descriptions of this problem. Spasm usually has been associated with profound ST-segment elevation on the ECG, hypotension, severe dysfunction of the ventricles, and myocardial irritability. Many hypotheses have been put forward to explain the origin of coronary artery spasm; some of the mechanisms that may play a role are demonstrated in Figure 18-20.580 The mechanism of postoperative spasm may or may not be the same as that underlying Prinzmetal’s variant angina, but the same stimuli seem to be present and therapy is usually effective with a wide range of vasodilators such as NTG, calcium channel blockers, milrinone, or combinations of NTG and calcium channel blockers in both situations. Arterial grafts such as the LIMA, and particularly radial artery grafts, are prone to spasm after revascularization, and its prevention and recognition are crucial to prevent serious complications.581 He et al582 tested the reactivity of ring segments of human IMAs in organ baths to various constrictor and dilator agents. It was found that thromboxane was the most potent IMA constrictor, followed by norepinephrine, serotonin, phenylephrine, and potassium chloride. NTG, NO, papaverine, and the calcium channel blockers nifedipine, verapamil, and diltiazem, as well as milrinone, all produced relaxation. In a similar study, Mussa et al583 observed the vasodilating properties of topically applied phenoxybenzamine. It prevented vasoconstriction with a long-lasting effect (>5 hours) in response to various vasoconstrictors, followed by verapamil/NTG (5 hours) and papaverine (1 hour). In vivo, for prophylaxis of IMA spasm, the calcium antagonists, especially diltiazem, were thought to be as useful as NTG.584–586
Nevertheless, prevention and treatment of arterial conduit spasm with diltiazem may cause serious adverse effects such as low CO or conduction abnormalities. Diltiazem is a more expensive drug compared with NTG. Shapira et al587 showed in vitro (radial artery, IMA, saphenous vein) and in vivo (radial artery) that NTG was a superior vasodilator compared with diltiazem. The same investigators monitored patients undergoing CABG surgery using radial artery grafts who were randomized to receive NTG or diltiazem intravenously after induction of anesthesia followed by an NTG patch or oral diltiazem after surgery for 6 months. There was no significant difference in outcome (morbidity, myocardial infarction, CK-MB) between the two groups. Nevertheless, the 6-month diltiazem treatment was associated with 16-fold greater costs and significantly more patients requiring cardiac pacing compared with NTG (28% vs. 13%, respectively).588 In a double-blind, randomized study, Mollhoff et al589 compared milrinone (0.375 μg/kg/min) with nifedipine (0.2 μg/kg/min) in patients with impaired LV function undergoing CABG surgery. ST changes after revascularization (including the use of the IMA graft) indicative of myocardial ischemia occurred in 33.3% of the milrinone group compared with 86.6% in the nifedipine group. Biochemical markers of myocardial damage (CK-MB and troponin I) after 24 hours were significantly greater in the nifedipine group.
Fast-Track Management for Coronary Artery Bypass Grafting Surgery
Efforts to reduce resource consumption for patients undergoing CABG have received considerable attention by a variety of interested payers since the 1990s. With the changing pattern of reimbursement based on the ever-escalating costs of health care, payers have drastically reduced incentives to expend unnecessary resources, with hospitals and clinicians attempting to minimize consumption yet maintain patient safety. The financial pressures are illustrated by the observation that Medicare costs for CABG increased from $2.8 billion in 1990 to $7.3 billion in 1997, whereas case volume increased only 40% during that period. In 1990, Krohn et al590 reported a study of 240 patients undergoing CABG at a private southern California hospital between 1984 and 1986, describing a clinical pathway emphasizing early extubation, rapid mobilization, intraoperative fluid restriction, and steroid administration. This program was associated with a median postoperative LOS of 4 days and in-hospital mortality rate of only 2%. This program itself was forged out of intense economic competition with the first major incursion of managed care into this area’s competitive market. This particular pathway is widely recognized as the first formal report of what is now called fast-tracking for cardiac surgery. In a similar time frame, reports from the financially constrained U.K. health care system, in which formal ICU care was “bypassed” based primarily on rapid early extubation (with apparent success), appeared.591,592 The publicity associated with these reports contributed to Medicare’s interest in cost reduction, leading to the Medicare Participating Heart Bypass Center Demonstration conducted between 1991 and 1996, in which seven participating hospitals agreed to a single sharply discounted rate for CABG (in return for preferential market share, a concept that ultimately has not materialized).593 Over the 5-year period, it is estimated that $50.3 million dollars were saved. In their summary report, reduction of cost and LOS by retooling processes of care is emphasized (and reducing time to extubation was considered a key factor). In the participating centers, LOS, together with mortality, declined annually despite increased severity of case mix. Observational data by Engelman et al,594,595 from the Baystate Medical Center of a large case series of fast-track patients, generated additional widespread publicity in the surgical community (particularly among members of the influential Society of Thoracic Surgery).
Although the fast-track clinical pathway encompasses a variety of perioperative (and after-hospital discharge) management strategies, early extubation is the one that has received perhaps the greatest attention (Box 18-8) (see Chapter 33). Because it is a simple, continuous variable (e.g., hours to extubation), it is one that many observational reports, a smaller number of randomized, controlled trials, and meta-analyses have reported to be safe and effective.596,597 Early extubation is acknowledged as a key component of the fast-track clinical pathway and one that was considered perhaps the most radical change in practice during the peak of scrutiny of the fast-track pathway (middle to late 1990s; Box 18-9). Reports of prolonged ventilatory management after cardiac surgery first appeared in the late 1950s (for valve surgery as CABG was not yet performed), and those from the 1960s (including the first reports of CABG patients) strongly advocated its routine use.598 This was further emphasized with the adoption of high-dose morphine and, subsequently, fentanyl and sufentanil, at the end of that decade.599,600
BOX 18-8. Perioperative Goals of Fast-Track Management