Chapter 40 Anesthesia for Laser Airway Surgery
II. Principles of Laser Technology
VI. Anesthetic Techniques for Laser Airway Surgery
VII. Anesthesia Management for Laryngeal Laser Surgery
VIII. Anesthetic Management for Tracheobronchial Tree Laser Surgery
I Introduction
Airway surgery is unique in that it involves the anesthesiologist and surgeon working in the same anatomic field. Even without the use of a laser in a shared airway, procedures are challenging when the airway is compromised. The addition of a laser beam (i.e., ignition source) into a shared airway can cause a catastrophic fire, and to avoid these disasters, all operating room staff must recognize that a team approach is essential (Fig. 40-1).1–39 If a high-risk situation exists, all team members must proactively agree on how a fire will be prevented and managed.1 The importance of a team approach cannot be overstated.
II Principles of Laser Technology
A History
The history and development of lasers parallels our understanding of electromagnetic radiation, ions, molecules, and atoms. In 1900, Max Planck revised his earlier work on radiation and the absorption of light and heat by a black body (i.e., a perfect absorber).40 He proposed that electromagnetic energy could be emitted only in quantized form. In this process, called the photoelectric effect, electrons are emitted from matter as a consequence of their absorption of energy from short-wavelength electromagnetic radiation, such as visible or ultraviolet light. In 1905, Albert Einstein described how the photoelectric effect was caused by absorption of quanta of light (i.e., photons) and explained that the energy of these quanta was directly related to the frequency of the radiation absorbed. From these observations, Einstein developed his ideas on quantum mechanics, and in 1917, he developed the quantum theory of radiation,41,42 in which he discussed the interactions of atoms, ions, molecules, photons, and electromagnetic radiation. According to the quantum theory of radiation, electrons, atoms, molecules, and photons interact with electromagnetic radiation of quantum units by absorption, spontaneous emission, and stimulated emission.43
In 1954, Charles Townes described the first amplification and generation of electromagnetic waves by stimulated emission by using microwaves, which he called microwave amplification by the stimulated emission of radiation (MASER). In 1960, Theodore Maiman produced light amplification by the stimulated emission of radiation (LASER) using a ruby crystal and red light. Throughout the 1960s and 1970s, many substrates and ions were tried as the laser medium. Some, such as the carbon dioxide (CO2) laser, were successfully developed, found to be useful in medical practice, and are still used.44
B Physics
In 1917, Einstein postulated that if a photon released from an excited atom collided with another atom already in the excited state, the second atom would release two photons with the same wavelength, phase, and direction (i.e., identical photons). If these two photons stimulated more excited atoms, more identical photons would be released. Einstein called this process stimulated emission of radiation, and it is the basic principle of laser physics (Fig. 40-2).45
All lasers consist of a laser medium (e.g., CO2, argon) contained in an optical cavity between two mirrors, one totally reflecting and one partially reflecting mirror. An external energy source continuously excites or pumps the laser medium, causing many of the atoms to reach a higher energy level (Fig. 40-3). When more than one half of the atoms are in an excited state, a population inversion has taken place. In this state, spontaneous emission occurs in every direction, but photons emitted in the direction of the optical cavity strike the mirror and are reflected back, striking excited atoms, and resulting in stimulated emission. This process repeats itself at an increasing rate with each passage of the photons reflected off the mirrors through the laser medium, generating a huge number of identical photons that all have the same wavelength, phase, and direction. The partially reflecting mirror lets a small amount of this energy escape through the aperture, creating the laser beam.
C Laser Parameters
2 Spot Size
After the laser light has emerged from the optical cavity of a laser system, it may pass through a lens that focuses the beam to a very small diameter, or spot size, ranging from 0.1 to 2.0 mm. The optical properties of each focusing lens determine the focal length, or distance from the lens to the intended target tissue.46
3 Power
When using lasers in clinical practice, power often is measured in watts (W), although a more useful term is the power density. For a given power, the effects vary according to the spot size and the time of exposure. The relationship between power and depth of tissue injury becomes logarithmic when the power and exposure time are kept constant and the spot size is varied.47 Power density describes the amount of power (W) arriving at a surface area (W/cm2).
D Laser-Tissue Interaction
When a laser interacts with tissues, the energy can be reflected, absorbed, conducted as heat, or scattered. The extent to which these processes occur depends on the type of laser used and the tissue with which it interacts. Laser wavelengths depend on the medium, and the degree of absorption depends on the target tissue components. The CO2 laser (10,600-nm wavelength) is particularly well absorbed by water, whereas the neodymium : yttrium-aluminum-garnet (Nd : YAG) laser (1064-nm wavelength) is absorbed less by water and creates more energy scatter. The argon laser (488- and 514-nm wavelengths) and potassium titanyl phosphate (KTP) laser (532-nm wavelength) are in the visible spectrum, and their energies are absorbed well by pigments such as melanin and hemoglobin. For the most commonly used lasers in medicine, absorption of laser energy excites the atoms within tissues, which produces heat (Fig. 40-4).
The CO2 laser heats the water within cells, and as the temperature rises to about 65° C, proteins denature and thermal necrosis occurs. As the heating continues and the temperature reaches 100° C, the water within the cell boils and produces water vapor; this leads to an explosive vaporization, and the cell explodes. The tissue surface in contact with the CO2 laser disintegrates and undergoes charring, with carbon debris deposited at about 350° C. There is little damage to the underlying structures. Surrounding the charred area is a zone of thermal necrosis, and around this is an area of thermal conductivity and repair.48
E Laser Classification
Class 1. These lasers are considered safe, with no risk when viewed by the naked eye. Class 1M denotes that the beam is potentially hazardous if it is viewed with an optical instrument, such as a microscope.
Class 2. These lasers emit low-power visible radiation in the range of 400 to 700 nm. An aversion reaction or blink reflex limits exposure to less than 0.25 seconds and provides protection from equipment such as a laser pointer. Class 2M devices are the same as class 2, but they may be hazardous if viewed with an optical instrument.
Class 3. A class 3R laser is considered safe with restricted beam viewing, and if the maximum permissible exposure is exceeded, the risk of injury is low. Class 3B lasers are hazardous if the eye is exposed directly, and protective eyewear is required if direct viewing of a laser beam may occur. A key switch and safety interlock must be present on all class 3B lasers.
Class 4. Most medical lasers belong in this class and pose dangers to the eye, skin, and combustible material. They are a fire risk and produce air contaminants and plumes. All class 3B and class 4 lasers must be operated by trained, authorized personnel and be equipped with a key switch and a safety interlock.
F Types of Laser
Lasers are named according to their medium, which can be a gas, solid, liquid, or semiconductor. Many types of lasers exist, and their clinical application depends on their wavelength, tissue absorptive characteristics, cost, ease of use, and safety. Lasers have differences in their emitted wavelength, output power, mode of operation (i.e., pulsed or continuous mode), and application (i.e., contact or noncontact). For laser airway surgery, the CO2, argon, Nd : YAG, KTP, and diode lasers are the most commonly used (Table 40-1).
1 Carbon Dioxide Laser
The CO2 laser has been used for many otolaryngology and head and neck surgical procedures and in gynecologic surgery for the treatment of intraepithelial neoplasms, condylomata acuminata, and other lesions. It is the most commonly used laser for airway surgery and is particularly suited to laryngeal and head and neck surgical procedures (Fig. 40-5).
The CO2 laser can be used in a continuous, pulsed, or superpulsed mode. The superpulsed mode reduces the exposure time to a few nanoseconds while delivering high energies of 400 to 500 W with each peak (Fig. 40-6). The rest time between each peak allows the tissues to cool and reduces thermal injury to adjacent tissues.
The CO2 laser has advantages that make it an effective surgical tool. Its use with an operating microscope allows the surgeon to precisely destroy targets approximately 2 mm in diameter under binocular vision. This degree of precision may be impossible to achieve with conventional cautery. During CO2 laser surgery, the surgical field is usually bloodless because of the laser’s considerable hemostatic action. Vessels up to 0.5 mm in diameter usually can be sectioned without bleeding.49 The CO2 laser has been used successfully to excise vascular lesions, even in patients with a bleeding diathesis.50 The use of more traditional surgical tools, such as cautery, usually results in considerable postoperative edema. Edema does not usually occur after using the CO2 laser because of the sharp line of tissue destruction, with virtually no injury to surrounding tissue. Ninety percent of the laser’s energy is absorbed within 0.03 mm.51 There is no manipulation of tissues because laser treatment is usually a noncontact technique. Microscopic examination of tissue after CO2 laser surgery reveals a discrete line of destruction, with preservation of capillaries and normal features of adjacent tissues. The preservation of adjacent tissues is thought to account for the rapid healing, minimal scarring, and reduced pain often observed after CO2 laser surgery.49
The CO2 laser is a safe and extremely useful surgical modality in the airways and the digestive tract.52 It is best suited for widening benign tracheal stenoses and for removal of granulation tissues.53
2 Flexible-Fiber Carbon Dioxide Laser
A drawback of CO2 lasers is that they are fixed to a line-of-site delivery mechanism and cannot be transmitted through ordinary fiberoptic bundles, which limits their use to areas accessible by a straight approach. Flexible-fiber CO2 lasers (Omniguide, Cambridge, MA; Fiberlase CO2 Fiber, Lumenis, CA) have been developed for use in airway procedures, including base of tongue tumors, laryngeal tumors, tracheal tumors, laryngeal papillomas, tracheal stenosis, and laryngeal lesions.54–59
3 Potassium Titanyl Phosphate Laser
The KTP laser is strongly absorbed by hemoglobin and melanin, and it is used for otolaryngologic lesions, vascular diseases, and hemorrhages.60 The KTP laser has been used to treat several skin conditions, including port wine stains, hemangioma, telangiectasia, spider nevi, and red scars of the skin (Fig. 40-7).
The KTP laser is generated by passing the light of a rapidly pulsed Nd : YAG laser through a potassium titanyl phosphate crystal, which doubles the frequency and halves the wavelength to 532 nm, producing a beam in the bright green visible spectrum that has a tissue penetration of 0.5 to 2 mm.60 The radiation is able to pass through a flexible fiberoptic bundle and is used in a pulsed mode. It is used in vascular lesions within the airway, and because it can be transmitted through clear substances and can pass through a flexible fiberoptic fiber, it can be used in areas where a direct line-of-site laser cannot. The KTP laser has been used for laryngotracheal stenosis, laryngeal paralysis, and choanal atresia. The convenience of fiber delivery, concomitant telescopic control, and low-grade edematous reaction were the main advantages over the CO2 laser. Healing time was longer for the KTP laser than for the CO2 laser.
4 Argon Laser
The argon laser contains argon gas and produces a visible blue-green beam with wavelengths of 488 nm and 514 nm, which are absorbed selectively by hemoglobin, melanin, and other pigments that lie under the retina. The beam is readily transmitted down a fiberoptic bundle, allowing endobronchial surgery (Fig. 40-8).
Applications of the argon laser include ophthalmologic surgery, especially for retinal and anterior chamber procedures. Because the laser passes readily through fluid inside the eye without damage, it is used in the treatment of retinal vascular lesions, including diabetic retinopathy, retinal detachment, glaucoma, and macular degeneration. Its applications in dermatologic and plastic surgery include the removal of port wine stains, hemangiomas, and tattoos because of the laser’s absorption by hemoglobin and other pigments. Because the tissue penetration is 0.5 to 2 mm, it is useful for superficial coagulation of capillary vessels. Port wine stains are lightened without scarring after treatment with the argon laser.50 To vaporize tissues, the power density is increased to produce a small focal spot; this allows argon laser stapedotomy. The argon laser has been used in infants to remove obstructive endobronchial lesions due to traumatic suction catheter injuries by passing argon laser fibers through the suction port of the fiberoptic bronchoscope (FOB) and targeting the obstructive endobronchial lesion with the argon laser beam.
5 Neodymium : Yttrium-Aluminum-Garnet Laser
The Nd : YAG laser has been used for photocoagulation and deep thermal necrosis in the treatment of gastrointestinal bleeding and obstructing bronchial lesions. The Nd : YAG laser uses a clear, solid, crystalline medium that emits radiation in the infrared region of the electromagnetic spectrum; the radiation has a wavelength of 1060 nm and is invisible (see Table 40-1). Conventional fiberoptic bundles readily transmit Nd : YAG laser radiation at high power, which is applied in a continuous or pulsed mode. The radiation is more readily absorbed by dark tissue. Blue or black pigmentation enhances Nd : YAG absorption, whereas pale colors enhance its penetration.61,62 Nd : YAG radiation penetrates tissues to a depth of 2 to 6 mm and provides good homeostasis for blood vessels up to 0.5 cm in diameter. However, the depth of penetration is less predictable than that of the CO2 laser. The power density below the tissue surface depends on the color of the surface, which makes laser penetration more difficult with the Nd : YAG laser than with the CO2 laser.
The Nd : YAG laser is recommended for lesions distal to the larynx and for bulky, vascular endobronchial neoplasms. The advantage of the Nd : YAG laser is that the radiation can be transmitted through fiberoptic bundles. Excision of lesions that are located distal to the larynx is complicated because it is difficult to reach the tumor with the laser beam. In these situations, the Nd : YAG laser is preferred because it can be used with a rigid bronchoscope or an FOB.62 Nd : YAG lasers are less precise in cutting, penetrate the tissue deeper, and have improved photocoagulation and superior hemostasis compared with other lasers.
Dumon and coworkers,63 reporting a large series of cases, recommended the use of rigid rather than flexible bronchoscopy for treating obstructive pulmonary lesions by means of endoscopic Nd : YAG laser surgery. Brutinel and associates reported difficulty ventilating and oxygenating patients’ lungs through an endotracheal tube (ETT) during Nd : YAG surgery with an indwelling FOB in place.64 Casey and colleagues reported combustion of the FOB and ETT in a patient undergoing Nd : YAG laser airway surgery.65 The use of a rigid bronchoscope for the treatment of obstructing pulmonary lesions facilitates the removal of tissue and the treatment of complications.66 Power levels less than 50 W given in short pulsations decrease the chances of impingement on vital underlying structures.66 McDougall and Cortese reported two patients who died during Nd : YAG laser endoscopic treatment of airway obstructions using very high power.67 At high power, the penetration of tissues by the Nd : YAG laser cannot be readily controlled, and perforation of a large blood vessel is possible.
6 Ruby Laser
The ruby laser uses a solid medium of a crystal aluminum oxide (i.e., sapphire) containing chromium ions. It emits visible red radiation at a wavelength of 695 nm (see Table 40-1). The ruby laser is used only in the pulse mode. The radiation is not readily absorbed by water but is significantly absorbed by pigments such as melanin and hemoglobin. The ruby laser can easily penetrate the anterior structures of the eye. It is used to photocoagulate vascular and pigmented retinal lesions. Use of this laser has decreased with the availability of newer types, and the ruby laser is not commonly used for laser airway surgery.
7 Diode Laser
The diode laser is another addition to a growing array of tools for laser surgery. This laser is effective for treatment of hyperplastic inferior nasal turbinates and provides good hemostasis and a sufficient reduction of tumors in otolaryngology practice.60 The continuous-wave, semiconductor diode laser emits radiation at wavelengths of 810 and 940 nm in the near-infrared spectrum. The radiation is delivered by a flexible fiber coupled with an aiming beam. The diode laser penetrates tissue to a depth of 1 to 3 mm, and it is suitable for vascular lesions. It is used to remove and debulk airway pathology, including tumors throughout the airway.
III Laser Hazards
A General Laser Hazards
1 Eye Damage
Lasers can easily damage the eyes by direct or indirect exposure, causing serious corneal and retinal injuries that may be irreversible. Eye protection is essential for anyone within the operating room, including the patient, auxiliary staff, nursing staff, surgeon, and anesthesiologist (Fig. 40-9). General precautions for the patient include taping the eyes closed and avoiding the use of petroleum-based eye lubricants. Further protection includes saline-soaked eye pads, protective eye glasses, or metal eye goggles, depending on the wavelength and type of laser used.
All laser glasses should be labeled clearly with the optical density value, the wavelengths against which protection is afforded, and maximum radiant exposure, or irradiance, to which the eyewear can be exposed (Figs. 40-10 and 40-11).68 The correct protective eye glasses must be used for the type of laser used for the procedure; failure to do so provides no protection for the eyes. In hospitals where only one type of laser is in use, this is less of a problem, but care must be taken in hospitals with many types of lasers and protective eye goggles.
For CO2 lasers, the damage to the eye is limited to the cornea because its radiation is largely absorbed by water, and the cornea is more than 75% water.61,69 There is no risk to the retina. For airway use, the patient’s eyes should be taped, protective saline-soaked eye pads placed, and surgical drapes applied and covered with another layer of saline-soaked swabs placed over the drapes. For the operating room staff, CO2 laser–protective eyeglasses should be worn. Contact lenses protect only the area covered and provide inadequate protection. Regular conventional eyeglasses can protect against the CO2 laser beam if the beam has been directed or reflected straight onto the eyeglass; however, if the beam is reflected accidentally into the side of the eyeglasses, damage to the eye can occur. When working with CO2, lasers, all operating room staff should use eyeglasses that wrap around the eye, protecting from CO2 laser entry from the side. The surgeon does not need to use CO2 laser protective eyeglasses when working with the operating microscope because the optics of the microscope provide protection70; however, if the surgeon uses the CO2 laser with a handpiece, the surgeon must also wear protective eyeglasses.
For Nd : YAG lasers, unprotected eyes may absorb and focus laser light at the ocular fundus, resulting in irreparable damage. Radiation from the KTP and other lasers such as the Nd : YAG can penetrate the cornea and lens of the eye, resulting in severe retinal damage. The endoscopist is at greatest risk because of the Nd : YAG laser’s potential for backscatter.62 The patient and all operating room staff should wear protective eyeglasses. Filters that absorb the Nd : YAG wavelength of 1.06 µm can be placed into rigid and flexible bronchoscopes. If the fibers break during laser surgery, the beam may be deflected to anywhere in the operating room.
For KTP and argon lasers, all operating room personnel require protective amber-colored eyeglasses, and for laser procedures on the face, metal goggles are used (Fig. 40-12). For rigid and flexible endoscopes and operating microscopes, filters that absorb the KTP wavelength can be introduced.
2 Skin and Drape Damage
Laser burns to the skin can occur, and the face or exposed areas should be protected with wet towels and drapes. For laser airway procedures involving a suspension laryngoscope, all of the area around the surgical laryngoscope and face should be completely covered with wet towels, which must be kept wet throughout the procedure. Care should be taken, particularly around the draping of the proximal portion of the surgical laryngoscope, to ensure that the lips and nose are fully protected because this region is more likely to be struck by a reflected laser beam from the proximal rim of the surgical laryngoscope (Fig. 40-13).
Preparation solution should not contain alcohol. Oxygen saturation monitors are a standard of care and should be used because detection of cyanosis is difficult with the patient’s face covered. Disposable surgical drapes are a potential fire hazard. They are treated with flame-retardant chemicals and are water resistant, but all types of surgical drapes are potentially flammable. Many cases of drapes catching fire have been reported. When the drapes catch fire, it is difficult to extinguish because the drapes are water resistant, and the water rolls off them. A CO2 fire extinguisher should be available. Drapes, once ignited, go up in flames immediately, causing the operating room to be inundated with smoke and making it difficult for everyone to see and breathe.71–73 It is important to keep the towels moist, or they can become flammable.
3 Laser Plume
The plume of smoke produced by vaporization of tissues in electrocautery or laser surgery may be hazardous. The smoke contains fine particles (mean size, 0.31 µm; range, 0.1 to 0.8 µm) that can be efficiently transported and deposited in the alveoli.74 In rat lungs, the deposition of laser plume particles could produce interstitial pneumonia, bronchiolitis, a reduction in mucociliary clearance, inflammation, and emphysema.75,76 The laser’s smoke plume acting as a vector for viruses is a controversial idea. Viral DNA has been detected in plumes from condylomas and skin warts but not from laryngeal papillomas.77–80 CO2 lasers seem to produce the most smoke from vaporization of tissue. Operating room personnel can be protected by using an efficient smoke evacuator at the surgical site (Fig. 40-14).81,82 Ordinary operating room masks can filter particles no smaller than 3.0 µm. Laser masks that are more efficient should be used to protect the operating room personnel from plume particles.
4 Gas Embolism
Gas embolism has been reported with Nd : YAG laser resection of tracheal and bronchial tumors, and it may be a risk factor for flexible-fiber CO2 lasers introduced into the bronchial tree.83,84 Gas embolism has been reported in laparoscopic surgery that used Nd : YAG laser probes that required a gas cooling system.85,86
5 Misdirected Laser and Laser Protocol
Lasers should always be set to the standby mode, except when they are ready to fire, to prevent inadvertent actuation. They should be used in the pulsed (shuttered) mode rather than the continuous mode whenever possible to limit the energy delivered by the laser and to allow the area being lasered to cool between firings. The laser radiation should never be allowed to strike highly polished or mirror-like surfaces. Most instrumentation for use with lasers has a dull or matte finish, and blackened instruments are widely used. This is important because reflection of the coherent laser beam may not disperse it, and injury to the patient or operating room personnel is possible, even from a reflected laser beam. Any instruments that become hot as a result of laser radiation may cause burns.68,87 Tracheal laceration, tooth damage, injury to soft tissue, and cutaneous burns to operating room personnel have been described during laser surgery.88
The Nd : YAG and argon lasers can penetrate glass, and any windows in the operating room should have an opaque covering to prevent penetration by laser radiation. A warning sign should be placed on the operating room door so that anyone entering is informed that the laser is in use (Figs. 40-15 and 40-16). To prevent personnel inadvertently entering the operating room during laser surgery, the doors may be automatically locked when the operating room is in laser mode (Fig. 40-17). Extra goggles should be available for personnel entering the operating room.
B Airway Laser Hazards
The high energy of the laser and its potential for combustion can cause an airway fire when the surgical field is near to the airway (Fig. 40-18). When a laser strikes the unprotected external surface of a tracheal tube during laser airway surgery, the surface starts to disintegrate and can catch fire. If the fire is not recognized and the laser continues to be applied, it can produce a hole in the tracheal tube and expose the burning surface to the oxidant-rich gas within the anesthesia system. At this stage, an explosive blowtorch-like fire may occur and rapidly spread in a distal and proximal manner. Any airway fire is a life-threatening complication, but the blowtorch fire is especially feared (Fig. 40-19).
If the cuff of an ETT is punctured, the oxidant-rich gas within the circuit becomes exposed to the external surface of the ETT, and the risk of an airway fire, including a blowtorch fire, is increased significantly. Examination of an ETT after a blowtorch laser fire reveals total or near-total destruction of the ETT, with molten material, smoke, and other particulate material spreading out from the distal end of the tube (Figs. 40-20 and 40-21).
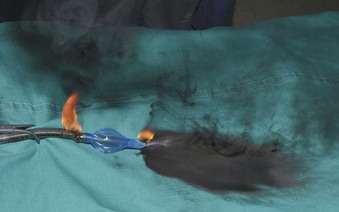
Figure 40-20 Smoke, molten material, and other particulate material spread out after an airway fire.
It is thought that operating room fires are under-reported and that there are probably 100 to 200 operating room fires in the United States per year. Of the reported fires, 20% result in serious injury to the patient. One or two deaths per year are caused by airway fires.89
Many options are available for anesthesia management during airway laser surgery. Selection of the ventilation method and type of laser depends on the nature and location of the lesion, the condition of the patient, and the availability of equipment and expertise. Different anesthesia management techniques have been described in the treatment of recurrent respiratory papillomatosis using the CO2 laser.90 In a 1995 survey, 92% of otolaryngologists preferred using a CO2 laser for removal of recurrent respiratory papillomas. However, there is no consensus with respect to anesthesia management. About 46% preferred using a laser-safe ETT, 26% favored using jet ventilation, 16% preferred an apneic technique, and 12% preferred spontaneous ventilation.91 Other options include awake with topical anesthesia, general anesthesia through an ETT, rigid bronchoscopy with general anesthesia, and general anesthesia by a laryngeal mask airway (LMA). The possibility of complete airway collapse or an inability to ventilate must be taken into consideration when deciding on spontaneous or positive-pressure ventilation.
If ventilation through an ETT is proposed, prevention of an ETT fire or explosion requires the use of special techniques and appropriate ETTs. Surveys of otolaryngologists active in this type of surgery concerning the complications of CO2 laser laryngeal surgery have found ETT fires or explosions to be the most common major complication.52,92 Historically, the estimated incidence of airway fires was between 0.4% and 0.57% of the patients undergoing laser airway surgery.39 The one or two deaths per year are caused by airway fires. As reported by Cozine and colleagues, patients have died because of combustion of an ETT during CO2 laser surgery.93
IV Preventing Airway Fires
A Fuel Source Considerations
1 Use of Metallic Foil Tapes to Protect Endotracheal Tubes
Metal foil tape wrapped around a standard ETT that is used during laser surgery in adults is of historical interest, but it is not commonly used for laser airway surgery. Foil tapes were first suggested as a simple, inexpensive means of protecting the shafts of combustible ETTs from laser beams by Strong and Jako in 1972.49 Their use during laser surgery was described during the 1970s and 1980s,94–97 when specially manufactured laser-resistant ETTs were not available or performed poorly.
Metallic foil tapes provide protection only from the direct impact of the laser beam. Indirect combustion caused by sparks or heat from gaseous or tube combustion is still possible because the ETTs used typically were combustible and usually had an enriched concentration of oxygen flowing through them. Problems with the use of metallic foil tape have included obstruction of the airway when the tape came loose from a wrapped ETT98,99 and aluminum foil tape becoming trapped in the trachea after laser airway supraglottoplasty.100
No metallic foil tapes are manufactured for medical applications, and the U.S. Food and Drug Administration has not sanctioned their use. The physician who wraps an ETT with metal foil tape incurs some product liability risk as a noncertified “manufacturer” if injury occurs.101 One manufacturer, when questioned, cautioned against the use of its aluminum tape for medical purposes.102
Wrapping the metal foil tape in a spiral, overlapping manner should cover the shaft from the cuff to the most proximal region possible and should include the pilot tube. Poor wrapping may cause some areas of the shaft to be unprotected, and bending of the tube may expose unprotected areas. Foil-wrapped tubes may loose flexibility, and their foil edge surface may traumatize. The type of metallic tape used to protect combustible ETTs is important.102
The possibility of changes in the composition of any metallic foil tape requires every batch of tape to be evaluated for its incendiary characteristics before use.95 Metal foil tape wrapped around a standard ETT is not recommended for laser airway surgery.
3 Prevention of Endotracheal Tube Cuff Fires with Saline
During the 1970s and 1980s, attempts were made to improve the laser-resistant properties of the shaft of combustible ETTs. In 1982, LeJeune and colleagues suggested that filling ETT cuffs with saline could protect them from the CO2 laser, because a laser strike on the cuff results in a jet of water that acts as a “built-in fire extinguisher” (Fig. 40-22).103 The saline also can act as a heat sink. Sosis and Dillon compared air-filled and saline-filled cuffs, and they found the saline-filled cuffs,104 although perforated by the laser beam as rapidly as air-filled cuffs, were significantly slower to deflate, allowing more time before reaching the point at which airway pressure could no longer be maintained. Saline-filled cuffs prevented ETT ignition by the CO2 laser set to 40 W (Table 40-2) in a statistically significant number of cases compared with the control group of air-filled cuffs, and saline filling of ETT cuffs was recommended for laser airway surgery. A small amount of dye, such as methylene blue, should be added to the saline so that laser-induced ETT cuff perforation becomes obvious to the surgeon, who can immediately terminate operation of the laser. Further protection of the ETT cuffs can be obtained by placing moistened pledgets above them and keeping the pledgets moist throughout the procedure.105
TABLE 40-2 Incidence of Endotracheal Tube Combustion with Air- and Saline-Filled Cuffs with the Carbon Dioxide Laser Set to 40 W
Cuff Type | Number of Cases | Combustion (%) |
---|---|---|
Air | 5 | 100* |
Saline | 5 | 20* |
* P < 0.05, Mann-Whitney U test.
From Sosis MB, Dillon FX: Saline-filled cuffs help prevent laser-induced polyvinylchloride endotracheal tube fires. Anesth Analg 72:187, 1991.
4 Special Endotracheal Tubes for Laser Airway Surgery
Laser proof implies that irrespective of the oxidant environment and the power of the laser, the ETT cannot catch fire. With a laser-proof ETT, a continuous laser strike with extremely high power in a 100% oxygen environment does not produce a fire of the tube. Only one laser-proof tracheal tube (Norton) has been designed, and it is discussed later.106
All other manufactured ETTs for laser airway surgery are only laser resistant. Laser-resistant tubes provide some degree of protection against a laser strike, but these tubes vary in their materials, protective coating, relative size, and number of cuffs used. Any laser-resistant tube can result in an airway fire or blowtorch fire (Fig. 40-23) if it is used outside of its limits, such as a laser strike on an unprotected area between the tube shaft distal and the cuff, at the unprotected proximal part of the shaft, or at the cuff itself. The anesthesiologist should appreciate the maximum power settings for which a laser-resistant tube has been tested, the range within which ignition does not occur, and the effect the oxidant environment has on the laser resistance characteristics. All laser-resistant tubes are not laser proof, and they must not be used outside of their limits.
a Norton Laser Endotracheal Tube
The Norton ETT (V. Mueller, Baxter Healthcare Corp., Niles, IL), first described in 1978 by Norton and De Vos, was the only laser-proof tracheal tube produced (Fig. 40-24).106 It was constructed from interlocking, spiral-wound, stainless steel parts. It is no longer manufactured. Because it was made of steel, it was extremely resistant to multiple uses and autoclaving, and it may still exist in some hospitals.
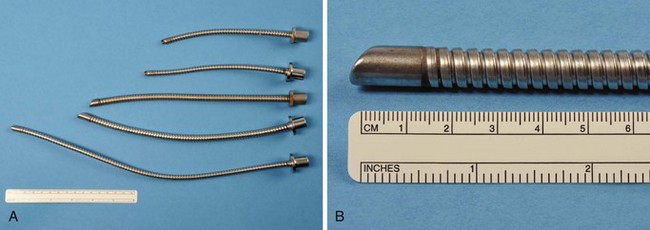
Figure 40-24 A and B, In the selection of Norton laser tubes, notice the absence of a cuff and ribbed exterior.
The Norton ETT had a matte or sand-blasted finish, rather thick walls, and a ribbed exterior. It came in three sizes: 4.0-, 4.8-, and 6.4-mm internal diameter (ID) (Table 40-3).107 The matte finish diffused reflected laser beams.106 A 4.8-mm-ID Norton ETT has a wall thickness of 1.4 mm. The thick wall of this ETT is considered a disadvantage because the tube can obscure the surgeon’s view more than an ETT with the same ID but with thinner walls—an important consideration during laser airway surgery. This ETT’s large size and stiffness may make surgical exposure and laryngoscope positioning difficult, and it can make passage through the glottis traumatic. It is usually necessary to use a stylet to introduce the Norton ETT.
TABLE 40-3 Internal Diameter, External Diameter, and Wall Thickness for the Norton Laser Endotracheal Tube
The relatively small diameter and ridged internal surface produce turbulent airflow and an increased resistance to airflow. Woo and Strong suggested Venturi ventilation through the Norton tube in an attempt to overcome the ventilation issues of increased resistance,108 airflow, and air leaks.
b Xomed Laser-Shield I Endotracheal Tube
The Xomed Laser-Shield I ETT (Xomed-Treace, Jacksonville, FL) is no longer manufactured and is of historical interest only. The silicon rubber tube was coated with a silicon elastomer to which metallic particles had been added, and it was designed to be used only with a CO2 laser. Several airway fires were reported with its use.109–112 It is a useful reminder of the limitations of a laser-resistant ETT and the dangers of using a device outside of its intended range.
c Bivona Laser Endotracheal Tube
The Bivona (Gary, IN) laser ETT is no longer available in many parts of the world and is mainly of historical interest. It was designed to limit the effect of cuff perforation by a laser strike, because the polyurethane foam cuff with its silicone envelope maintained the cuff seal even when penetrated. The Bivona laser ETT was designed to be used only with a CO2 laser. Blowtorch airway fires were reported with this tube.112
d Xomed Laser-Shield II Endotracheal Tube
The Xomed Laser-Shield II ETT (Xomed Surgical Products, Jacksonville, FL) has a laser-resistant overwrap of aluminum foil tape and a Teflon cover. The proximal and distal ends of the silicon elastomer shaft and cuff are not protected and therefore are not laser resistant (Fig. 40-25). The metallic tape provides protection against laser impact. The Teflon tape gives the tube a smooth surface to minimize mucosal injury from tube manipulation. No adhesives are used because adhesives with metallic tape increase the risk of an ETT fire.98 Dry methylene blue dye has been placed in the cuff inflation valve to enable detection of a cuff rupture. The Xomed Laser-Shield II comes packaged with neurosurgical cottonoid, which is used wet to protect the distal shaft and cuff for an added margin of safety.