Chapter 6 Airway Pharmacology
I Introduction
II Airway Pharmacology in Normal Airway Physiology
A Clinical Issues
B Physiology of the Upper Airway
Physiology of the upper airway is considered in detail in Chapter 5. In the awake patient, airway patency is maintained by muscle tone in the head and neck, particularly the pharynx and tongue. The upper airway muscles are influenced by afferent information from a number of sources (Fig. 6-1), including receptors in the upper airway and lung that respond to changes in airway pressure and a variety of chemical and mechanical stimuli.
C Effects of Drugs on the Airway
1 Direct Effect
Several types of drugs can be administered topically to the airway.
1. Local anesthetics attenuate the afferent limbs of the powerful reflexes that serve to protect the upper airway from aspiration of foreign material.
2. Sympathomimetics, such as epinephrine and phenylephrine, cause local vasoconstriction to minimize tissue vascularity or reduce edema.
3. Volatile inhalational agents cross the alveolar-capillary membrane to have an indirect effect on the respiratory tract via the CNS, but they also have direct effects, including bronchodilation and mucosal irritation.
4. Bronchodilators are commonly administered topically, as reviewed in the second part of this chapter.
2 Indirect Effect
1. Sedatives and analgesics affect central and peripheral neurologic pathways, largely motor but also sensory, to lessen muscle tone in the upper and lower airways and thereby affect patency and gas flow. Secondary effects on O2 and CO2 tension also affect airway tone.
2. Neuromuscular blocking (NMB) agents clearly affect skeletal muscle tone and have a major influence on airway management under general anesthesia.
3. Lidocaine, given intravenously, affects airway reactivity.
4. Antihistamines, used topically in the treatment of rhinitis, can be administered systemically to attenuate histamine-induced reflex bronchoconstriction.
5. Antimuscarinics, such as atropine, inhibit the parasympathetic nervous system to reduce salivary gland secretions and dry the upper airway. Upper airway tone is not significantly affected by this antiparasympathetic action, but lower airway resistance is reduced and airway reactivity is attenuated when drugs such as ipratropium bromide are given parenterally or are inhaled.
6. Respiratory stimulants, such as doxapram, are used occasionally to provoke spontaneous respiratory effort on cessation of general anesthesia. IV doxapram stimulates chemoreceptors in the carotid arteries, which in turn stimulate the respiratory center in the brainstem to increase respiratory muscle activity. Potentially serious side effects include cardiovascular instability and cerebral irritation.
III Drugs with a Direct Effect on the Airway
A Local Anesthetics
Local anesthetics produce a rapid, reversible depression of nerve conduction, particularly with regard to sensory nerves, by binding to sodium channels and interfering with their function, thereby preventing propagation of action potentials. Chemically, they usually consist of a lipophilic group connected to an ionizable group, typically a tertiary amine, by either an ester or an amide link (Fig. 6-2).
1 Complications of Airway Local Anesthesia
A reduction in upper airway patency has been demonstrated after topicalization of the airway.1,2 This occurs because of the loss of afferent feedback and the resultant decrease in motor tone, which may lead to upper airway obstruction, particularly in an airway that is already compromised. Hence, care is needed when considering an awake airway management technique in a patient with evidence of upper airway narrowing.
Systemic toxicity is extremely rare in patients receiving local anesthesia for instrumentation or examination of the upper or lower airway. Most reports suggest that plasma lidocaine levels very rarely approach the reported toxic plasma concentration of 5 to 6 µg/mL. However, one study demonstrated near-toxic levels after gargling of large volumes of lidocaine.3 Toxicity tends to involve the CNS with irritability or convulsions and the cardiovascular system with circulatory compromise. The safe maximum dose for airway administration for the most commonly used agent, lidocaine, is uncertain, but in one study in which patients received 9 mg/kg lidocaine, none had plasma concentrations in excess of 5 mg/mL.4 However, the death of a healthy volunteer due to presumed lidocaine toxicity after topicalization for fiberoptic bronchoscopy has been reported in the literature, so some consideration of the dose administered is advisable.5 The British Thoracic Society recommendation for the use of topical lidocaine with a flexible fiberoptic bronchoscope in adults is not to exceed 8.2 mg/kg.6
2 Specific Local Anesthetic Agents
d Cocaine
Cocaine, available in solutions of 1% to 10%, provides excellent topical anesthesia and has the advantage of providing intense vasoconstriction, which is often desirable as part of topicalization of the nasal passages before intubation. However, concerns about sympathomimetic toxicity and abuse potential have reduced its use in recent years, particularly in children, since a fatality was reported.7
B Sympathomimetics
The use of sympathomimetics to influence vascular blood flow in airway mucosal vessels is a technique that is commonly used in cases of acutely compromised upper airway in an attempt to reduce swelling. The most common agent used for this purpose is epinephrine, administered via a nebulizer.8 Vasoconstrictors such as phenylephrine are also used electively to reduce the potential for bleeding during upper airway instrumentation (e.g., nasotracheal intubation).
Topical vasoconstrictors such as epinephrine can also be used to minimize systemic absorption of topical local anesthetics and thereby reduce toxicity, improve efficacy, and increase duration of action.9 Unwanted effects of sympathomimetics are uncommon and largely relate to systemic absorption of the drug, which can result in an increase in cardiac work.
C Inhaled Volatile Agents
Inhaled anesthetic agents have a number of direct effects. For example, all volatile agents cause bronchodilation (i.e., increase in the diameter of the lower airways). Isoflurane appears to have the most potent effect, whereas desflurane also has the potential to cause the reverse effect and induce airway constriction.10
Airway irritation with increased salivary, laryngeal, and bronchial secretions (caused by an increase in mucus production and a decrease in mucociliary flow rates) varies among agents, with sevoflurane and halothane being essentially nonirritant and isoflurane and desflurane being potential irritants. This stimulation of airway receptors also appears to be responsible for the tachycardia and hypertension observed when desflurane is administered in high concentrations. The depressed mucociliary function seen with volatile agents is likely to contribute to impaired clearance of secretions and potentially to postoperative respiratory complications. The concomitant use of IV opioids lessens the irritant effect of inhaled volatile agents such as desflurane.11
IV Drugs with an Indirect Effect on the Airway
A Sedatives and Upper Airway Patency
The effect of general anesthetic agents on the upper airway is crucial to the practice of safe airway management, largely in terms of airway patency but also in terms of airway reactivity and airway protection against aspiration. There is a decrease in muscle tone associated with the loss of wakefulness, and this is compounded by specific drug-induced inhibition of upper airway neural and muscle activity and suppression of protective arousal responses. These processes tend to narrow the airway lumen, leading to airway obstruction. The mechanism of the reduction in muscle electrical activity relates, in part, to depressed hypoglossal nerve activity that occurs in excess of a similar depressant effect on other respiratory muscles, such as the diaphragm or intercostal muscles, which are more resistant to this dose-dependent loss of muscle tone.12 This reduction in hypoglossal nerve activity results in reduced tone in the muscles of the tongue and soft palate.
The differential suppression of respiratory muscles seems to vary among sedative agents, being less pronounced, for example, with ketamine than with other agents.13 Ketamine also has an indirect effect on the lower airways, causing bronchodilation by inducing the release of endogenous catecholamines. However, the loss of muscle tone caused by sedatives may not only relate to a specific effect of motor nerves. The depressed state of arousal results in a reduction of inputs from the reticular activating system to these motor nerves. This theory is borne out by changes in upper airway patency demonstrated during natural sleep, which show similarities to those induced by sedative drugs.
Originally, the upper airway narrowing associated with anesthesia was attributed to a posterior shift of the base of the tongue. Evidence now suggests that the soft palate and, to a lesser extent, the epiglottis are more relevant (Fig. 6-3). One study using lateral radiography demonstrated that occlusion of the airway under general anesthesia occurred most consistently at the level of the soft palate and sometimes at the level of the epiglottis, but in no patient did the tongue base come into contact with the posterior pharyngeal wall.14
Despite the assumption that loss of upper airway patency relates to a reduction in tone of the surrounding muscles, a consistent relationship between electromyographic activity and upper airway resistance has not been demonstrated. Thiopental causes measurable changes in muscular activity, but the changes are inconsistent and do not correlate with the onset of airway obstruction.15 Similarly, benzodiazepines increase upper airway resistance but again do not appear to have a consistent effect on genioglossus activity.16 Therefore, airway obstruction under general anesthesia may not relate to a simple diminution of muscular activity but to a disruption of the normal coordination of muscle activity that provides overall control of upper airway function.
The phase of the respiratory cycle is also relevant to the patency of the upper airway during spontaneous ventilation under general anesthesia. In a study by Nandi and colleagues, the movement of the soft palate during expiration was similar to that during apnea. However, during attempted inspiration, there was a significant reduction in the airway lumen at all levels, with the tongue now reaching the posterior pharyngeal wall in some cases and thus contributing further to the airway obstruction.14 This has relevance when one considers upper airway patency during anesthesia with or without muscle paralysis. The dynamic collapse of the upper airway that occurs during spontaneous inspiration anesthesia may be insignificant when pharmacologic paralysis is achieved. Similarly, the application of continuous positive airway pressure (CPAP) may also reduce this effect and help to maintain gas flow.
In summary, the increased collapsibility associated with anesthesia and sedation seems to be universal, regardless of whether the route of administration is inhalational or parenteral. Although this collapsibility lessens with decreasing anesthetic depth, the two do not necessarily have a linear relationship.17,18 The extent of the upper airway narrowing may vary subtly among agents, but the only clinically relevant difference appears to be the described lesser effect with ketamine.
At a laryngeal level, the effect of general anesthesia is probably different. One study demonstrated that the width of the laryngeal vestibule increased after administration of thiopental and succinylcholine.19
B Effect of Sedatives on Airway Reactivity and Airway Protection
Airway reflexes such as coughing, the expiration reflex, breath-holding, and laryngospasm are important in protecting the airway against contamination. These airway reflexes are usually obtunded by general anesthesia, although the exact relationship between specific agents and this suppression of activity has not been fully explored. Propofol, for example, has been shown to depress these reflexes,20 but vigorous airway reflexes have been demonstrated under baseline propofol anesthesia, suggesting that the depression with propofol as a lone agent is relatively limited in moderate doses. Combination with fentanyl, however, appears to have a significant influence on lessening airway reflexes.21
D Sedatives and the Lower Airway
All sedative agents that reduce muscular tone are likely to contribute to a reduction in a patient’s functional residual capacity (FRC) and an increased work of breathing. Benzodiazepines appear not to have a significant effect on lower airway patency, despite causing an apparent relaxation of tracheal smooth muscle and potentially attenuating reflex bronchoconstriction. The effect of IV anesthetic agents on bronchial smooth muscle is also unlikely to be of clinical significance. In vitro data suggest that propofol has a direct relaxant action.22 However, case reports and studies of IV induction agents used in asthmatics suggest that ketamine is the only agent with a significant bronchodilatory action. In contrast, volatile agents are fairly potent bronchodilators and have been used in the treatment of status asthmaticus.23,24
E Opioid Analgesics and the Airway
When fentanyl is combined with propofol, the depressant effect on upper airway reflexes is considerably more profound than with propofol alone, with the cough reflex being the most suppressed reflex and laryngospasm being the most resistant.21 The central mechanisms for this depression of reflexes are unclear and are probably complex.
Muscle rigidity associated with opioid use has been described25; although rare, it may be the direct cause of a significant increase in lower airway resistance that can make ventilation extremely difficult. Others suggest that this rare phenomenon in fact has more to do with vocal cord closure than with decreased pulmonary compliance secondary to chest wall rigidity.26 If the increase in airway resistance is truly linked to muscle rigidity, then its clinical manifestation is unpredictable and relates to many factors, including the opioid drug, its dose, its speed of administration, the effects of concomitant sedatives and muscle relaxants, and the age of the patient.
Short-acting narcotics are used at times in place of NMB agents, in combination with sedatives, to facilitate endotracheal intubation. However, upper airway conditions for airway instrumentation may be inferior, and the risks of arterial hypotension and laryngeal trauma may be higher.27
Remifentanil merits specific discussion because it is a relatively new drug in anesthesia and its use in facilitating safe and effective airway management is increasing. It is a potent, ultrashort-acting synthetic opioid that is administered typically by continuous infusion because it is immediately metabolized in the plasma. It causes profound respiratory depression and therefore is used clinically to successfully prevent spontaneous respiratory effort when assisted ventilation is the chosen mode of gas exchange. It also reduces muscle tone considerably and can be used to facilitate endotracheal intubation in the absence of NMB drugs.28 Use of remifentanil to facilitate smooth extubation is well described, and for some anesthesiologists,29 this technique has replaced deep general anesthesia extubation. Continuing remifentanil while discontinuing sedation results in a predominant effect of suppression of upper airway reflexes, in excess of any sedative effect. This allows extubation with good endotracheal tube (ETT) tolerance and a minimum of coughing. However, care is required when using the drug because of its potency. Apnea is likely and can even occur in a patient who is receiving remifentanil alone for conscious sedation.30 As with other synthetic opioids, muscle rigidity is described but not common.31
The main effect of opioids on the lower airway is similar to that described earlier, namely reduced airway reactivity and attenuated reflex bronchoconstriction. Mechanisms of action are again a combination of a neural effect and a direct dose-dependent effect in relaxing airway smooth muscle. For example, there is evidence that morphine attenuates vagally mediated bronchoconstriction in asthmatics.32 Opioid administration has been shown to cause histamine release, and there is also evidence that they cause an increase in tracheal smooth muscle tone, but neither effect has been associated with clinically demonstrable bronchoconstriction. A condition termed wooden chest syndrome has been described after administration of IV opioids, particularly if given at high dose; in this syndrome, thoracic and abdominal muscle rigidity apparently results in difficulty with assisted ventilation. The clinical significance of this finding is uncertain, but it has not restricted the use of these drugs worldwide.
F Neuromuscular Blockade and the Airway
NMB agents have several properties that may affect airway function. They are used in clinical practice to facilitate endotracheal intubation by abolishing airway reflexes and to facilitate mechanical ventilation by eliminating spontaneous respiratory effort. Similar to the effects of sedative agents on the respiratory muscles, these drugs also demonstrate a differential effect, with the diaphragm and the adductor muscles of the larynx being more resistant to paralysis than some of the muscles affecting upper airway patency.33 This relative sparing of the diaphragm permits the maintenance of respiratory effort even during complete paralysis of peripheral muscles. However, it also means that upper airway patency may be compromised at levels of paralysis that otherwise permit maintenance of normal spontaneous ventilation. Clinically, this is most relevant at extubation. A patient with residual NMB drug may maintain adequate ventilation with an artificial airway, such as an ETT or a supralaryngeal airway (SLA), in situ. After removal of the device, airway obstruction leading to hypoxemia may develop.
Removal of protective airway reflexes can have negative consequences if there is a risk of aspiration. Similarly, elimination of spontaneous respiratory effort may be deleterious if assisted ventilation cannot be delivered. Hence, care and experience are required for the safe use of NMB agents, and in certain clinical scenarios, it may be prudent to withhold them. However, the major clinical debate regarding their use seems to relate to their effect on upper airway patency. There is evidence that paralysis of the upper airway musculature improves upper airway patency. A recent study published data indicating that NMB using rocuronium facilitated bag-mask ventilation in anesthetized patients (Fig. 6-4).34
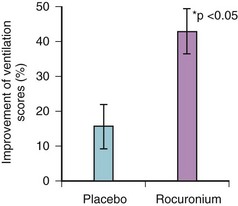
Figure 6-4 Efficacy of facemask ventilation in anesthetized patients with or without neuromuscular blockade.
(From: Szabo TA, Spinale FG, et al: Neuromuscular blockade facilitates mask ventilation. Anesthesiology 109:A184, 2008.)
Another study on the physiologic comparison of spontaneous ventilation and positive-pressure ventilation (PPV) in laryngotracheal stenosis concluded that spontaneous ventilation creates a negative inspiratory extrathoracic intratracheal pressure that causes indrawing of the mobile tracheal segments, further narrowing the airway.35 During expiration, there is positive intratracheal pressure, and a similar reduction in airflow does not occur. During PPV with muscle paralysis, however, a positive intraluminal pressure is created during inspiration that improves ventilation. Whether the conclusions of this paper can be extrapolated to the management of the upper airway under general anesthesia per se is uncertain, but several leading airway experts feel strongly that they should. The argument states that muscle relaxation makes upper airway management easier (and therefore safer) in terms of ventilation through a facemask or SLA and in terms of airway instrumentation, including intubation.36,37
Historically, there has been a fear that the prolonged effect of muscle paralysis during failing airway management under anesthesia contributes to severe hypoxic damage, but there does not appear to be any convincing evidence to support this supposition. The fear appears to relate to the loss of reversibility of the anesthetic technique, although it can never be certain whether the awakening of a patient during failing airway management is feasible before catastrophic desaturation occurs. The evidence suggests that, when faced with impossible bag-mask ventilation, very few anesthesiologists choose to discontinue the general anesthesia or avoid NMB.38 Most choose to provide oxygenation by intubating the trachea or by ventilating through an SLA device or performing an emergency cricothyrotomy. If ventilation is possible but intubation has been unsuccessful, “wake up” is strongly advocated by discontinuing any sedative drugs while reestablishing muscular tone and respiratory drive and maintaining oxygenation.
Impaired neuromuscular transmission secondary to partial pharmacologic blockade, even to a degree insufficient to evoke respiratory symptoms, markedly impairs upper airway dimensions and function. This may be explained by an impairment of the balance between upper airway dilating forces and the negative intraluminal pressure generated during inspiration. The same effect does not appear to occur during expiration.39 Furthermore, reflex glottic activity may be preserved, with a tendency toward coughing and laryngospasm, and it may also have a negative effect on gas flow. Complete muscular paralysis necessitating assisted PPV appears to have the reverse effect and increases upper airway patency.
Monitoring of the degree of NMB during anesthesia involving muscle relaxants is indicated, but there are limitations with the available techniques. The degree of blockade is frequently monitored by the response of the adductor pollicis to stimulation of the ulnar nerve, although this muscle is more sensitive than the diaphragm to paralysis.33 At present, monitoring methods that would reliably demonstrate the return of power required to maintain and protect the airway with an effective cough are lacking, and anesthesiologists tend to use a combination of monitoring and clinical assessment.
Reversal of nondepolarizing NMB before extubation and discontinuation of assisted ventilation is standard anesthetic practice. Use of a cholinesterase inhibitor, such as neostigmine, to flood the neuromuscular junction with acetylcholine and thereby restore neuromuscular function has traditionally been the only technique available for reversing the paralysis. These drugs could theoretically cause bronchospasm, but they appear to have no significant effect on airway resistance when combined with an anticholinergic drug such as atropine or glycopyrrolate. More importantly, inadequate reversal of the block, which is probably much more common than most anesthesiologists appreciate, can result in respiratory compromise, morbidity, and mortality.40 A meta-analysis of current reports of NMB in anesthesia revealed a 41.3% incidence of residual paralysis.41
G Other Drugs with Indirect Effect on the Upper Airway
IV lidocaine affects airway tone and reactivity, tending to relax smooth muscle and attenuate reflex bronchoconstriction such as that caused by intubation in asthmatics.42 However, the effect is unpredictable, and reports of IV lidocaine’s actually causing bronchoconstriction have been published.43 Historically, IV lidocaine has been used to improve intubation and extubation conditions by reducing upper airway reactivity.
V Airway Pharmacology for the Treatment of Asthma
Asthma (from the Greek, asthma, “panting”), as defined by the Global Initiative for Asthma (GINA),44 is a “chronic inflammatory disorder of the airways in which many cellular elements play a role. The chronic inflammation is associated with airway hyperresponsiveness that leads to recurrent episodes of wheezing, breathlessness and coughing, particularly at night or in the early morning. These episodes are usually associated with widespread, but variable, airflow obstruction within the lung that is often reversible either spontaneously or with treatment.”
A Overview
The complex, recursive interplay of inflammation, hyperresponsiveness, and airflow limitation determines the disease course, which over time produces structural airway changes, termed remodeling. There is presently greater recognition of the essentially inflammatory nature to asthma, with treatment directed at reducing this component over the long term as well as providing treatment to alleviate symptoms.45 An idea of the interplay of these factors is shown in Figure 6-5.
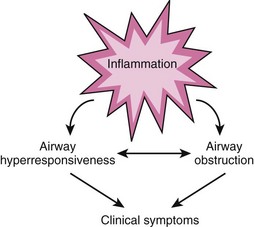
Figure 6-5 Interplay of airway inflammation, hyperresponsiveness, and obstruction.
(Adapted from National Asthma Education and Prevention Program: Expert Panel Report III: Guidelines for the diagnosis and management of asthma. Summary report 2007. J Allergy Clin Immunol 120[Suppl 5]:S94–S138, 2007.)
1 Diagnosis
Asthma is a clinical diagnosis, based on the symptoms and signs. Symptoms are variable, with one or more of the following being present: wheeze, breathlessness, cough, and chest tightness.46,47 None of these symptoms is specific to asthma. For example, wheeze is a function of any airway narrowing as a consequence of reduction of FRC. Signs of asthma include clinical verification by auscultation, spirometry, and lung function testing, which may include gas transfer measurements.48
There are no standard definitions for types of symptoms, nor for frequency or severity.47
2 Prevalence
Worldwide, asthma is estimated to affect more than 300 million people, 7% to 10% of the global population.49 About 15 million daily adjusted life-years (DALYs) are lost each year, which places asthma 20th in the current disability ranking, similar to schizophrenia and diabetes. There are about 225,000 asthma deaths each year, amounting to 1 in 250 deaths from all causes.50 In the United States, 7.3% of the population is diagnosed with asthma, and asthma accounted for 17 million physician consultations in 2006.51 The worldwide prevalence of asthma is predicted to increase by another 100 million by 2025.46
3 Distribution
There are marked differences in national and geographic distribution. Although methodologic factors may confound accurate measurement, there is widespread agreement that asthma is a disease of industrialized nations.46 Indoor aeroallergens, especially house dust mite52; tobacco smoking53; and obesity are some implicated factors.54,55
Genetic factors may contribute. Inheritance of an “asthmatic genotype” is complex with variable transmission.56 Atopy, the development of immunoglobulin E (IgE) antibodies to specific allergens, is the strongest identifiable risk for developing asthma.46,57,58 A family history of asthma, particularly on the maternal side, is a strong risk factor.59 Other inheritable traits are the forced expiratory volume in 1 second (FEV1) and response to drug therapies.56
Additionally, there are gender differences, with a higher incidence of asthma in prepubertal boys compared to girls.60 In middle age, however, there is a higher incidence among women.
4 Pathology
The pathology of asthma is incompletely understood and is an area of active research.61,62
a Airflow Limitation
Airflow limitation results from a combination of bronchoconstriction, inflammatory edema, and hypersecretion of mucus with airway plugging. Blood vessel hyperemia may also narrow small airways.63,64 These factors, either individually or collectively, narrow airways with impairment of respiratory gas exchange.
Mucus has a complex and variable structure. It is composed of long chains of mucin glycoproteins covalently cross-linked by disulphide bonds, polymerized DNA (from dead cells, particularly eosinophils), and polymerized (fibrous) F-actin together with a mixture of chemical mediators, modulators, and active, inactive, dead, or dying inflammatory cells65 (Fig. 6-6).
Green sputum is common but is not always indicative of infection, rather resulting from an abundance of eosinophils.66 When infection occurs, it is usually viral. Bacterial infection is uncommon.67 Most asthma exacerbations attributed to infection are from viruses,68 particularly rhinovirus.69
c Inflammation
One crucial aspect of asthma is considered to be the relative dominance of pro-inflammatory over anti-inflammatory factors driven by a variety of cell types in complex, dynamic, and variable ways. CD4+ lymphocytes, particularly the T helper type 2 (Th2) subtype, are presently thought to have a key role, with a predominance of this population over the T helper 1 (Th1) group. Relative overactivity of the Th2 group, especially at crucial developmental (childhood) stages, with expression of inflammatory cytokines such as interleukin-4 (IL-4), IL-5, IL-9, and IL-13, is considered to play a pivotal role.70
The inflammatory cytokines recruit and activate mast cells and eosinophils, leading to cellular infiltration, the density of which is often linked to disease severity.71 Some interleukins (IL-4 and IL-13) promote B-lymphocyte production of IgE in response to inhalation of allergens (aeroallergens). IgE binds to mast cell surface receptors, conferring immunologic memory and triggering release of mast cell contents (degranulation) when exposed to the specific aeroallergen.
A variety of substances such as histamine, proteases (including mast cell tryptase, a time-sensitive marker of degranulation), and cysteinyl-leukotrienes are released.72 At least 100 mediators have been described.73 Other stimuli, such as mechanical changes around these cells, may account for mast cell degranulation in response to cold-induced and exercise-induced asthma.74
Other cells, such as natural killer cells,75 Th17 cells,76 regulatory T cells,77 dendritic cells,78,79 macrophages,80 mast cells,81 and neutrophils, may be involved.82 Neutrophil activity is associated with severe disease and sudden deterioration.83
d Remodeling
Poorly controlled asthma may be complicated by anatomic changes in airway structure, which are often correlated to worsening of the disease84 but may start before diagnosis.85 A variety of cells are implicated in causing smooth muscle hypertrophy and hyperplasia, subepithelial fibrosis, angiogenesis, and mucus gland hyperplasia—collectively called the epithelial-mesenchymal trophic unit.
Recent attention has focused on the role of pulmonary blood flow in asthmatics, which is higher at rest compared with control subjects and increases during episodes of asthma enough to interfere with airflow.86
5 Treatment of Asthma
Major guidelines on treatment have been published by the National Asthma Education and Prevention Program (NAEPP) in the United States,45 by GINA,46 and by the British Thoracic Society in association with the Scottish Intercollegiate Guideline Network (SIGN) in the United Kingdom.47 Management strategies for asthma in adults (including pregnant women), babies, and young children are covered. Treatment is broadly similar, but there are some differences in drugs chosen and doses used. Intensive care and intraoperative management of critical asthma generally lie outside the scope of these guidelines and therefore are covered in more detail in this chapter.
The guidelines aim to provide common terms describing asthma, but other terms are often used within the literature in general, and definitions vary (Table 6-1). Of note is the term status asthmaticus, which refers to a life-threatening condition that mandates immediate attention, treatment, or escalation of therapy. This term can be applied to severe asthmatic episodes that are resistant to orthodox therapy or require mechanical ventilation.
Descriptor | Features |
---|---|
Exacerbation, moderate | PF 50%-75% of predicted |
Exacerbation, severe | PF 30%-50% of predicted, tachypnea (>25 breaths/min), tachycardia (>110 beats/min) |
Life-threatening | Reduced GCS, systemic hypotension, “silent chest,” hypoxemia |
Near-fatal | As for life-threatening, plus arterial hypercarbia, need for ventilation |
Brittle | Sudden, severe exacerbation or high PF variability to 50%-75% of predicted |
Status asthmaticus | Either life-threatening or near-fatal |
GCS, Glasgow Coma Score; PF, peak expiratory flow rate.
6 Routes of Drug Delivery
Drugs for inhalation require a dedicated device for delivery.87 Three main types are available: dry-powder inhalers (DPI), pressurized metered-dose inhalers (pMDI), and nebulizers. All are designed to produce an aerosol for delivery to the lungs. An aerosol is a group of particles having a low settling volume. A practical measure is given by the mass mean aerodynamic diameter (MMAD), a product of mean diameter and square root density. Effective MMAD for therapeutic aerosols is between 0.5 and 5 µm.88
For lower values, the particles do not impact the airway and are exhaled. For larger values, impaction occurs in the upper airway,89 with loss of efficacy and potential for local complications (e.g., oral candidiasis for inhaled glucocorticoids [IGCs])90 and for systemic toxicity resulting from swallowing deposited drug. The aerosol dose reaching the lungs is called the respirable mass. Additional factors influencing drug response include patient compliance, inhalation technique, and the use of adjuncts to aid delivery from the device to the patient.
pMDIs contain drug with propellants, originally chlorofluorocarbons (CFC11 and CFC12). These chemicals contributed to atmospheric ozone depletion and have now been replaced by halogenated fluoroalkanes (HFA227 and HFA134A). Use of HFA as a propellant has allowed reduction of MMAD for steroids from 4 to 1 µm, which increases the respirable mass and allows dosage from the device to be reduced. pMDIs require breath actuation during inspiration with a sustained, but slow, inspiratory flow. Patient technique can be learned but is variable. The use of an adjunct, such as a spacer device with a face mask or mouthpiece, helps.91 Devices with breath-actuated valves are superior. Selection of the face mask is important, and both size and seal must be considered. For small children, the dead space of some face masks is greater than their tidal volume (VT).92 If the face mask seal is poor, aerosol leakage considerably reduces efficacy.93 Use of a mouthpiece produces superior drug delivery, compared with a face mask, but requires greater patient coordination.94 Older spacing devices accumulated static charge that reduced aerosol delivery. Newer versions are “charge-reduced.”95
Nebulizers vary in concept and design. The most common type is the jet nebulizer. It uses a high flow of driving gas through a narrow orifice, creating a Venturi effect, which draws the liquid drug into droplets that can be inhaled.96 The aerosol produced is a mixture of droplet sizes (polydisperse). Compared with other devices, drug delivery is less efficient overall, with proportions of the drug lost as mist. In general, however, nebulizer therapy does not require patient technique, allows drug doses to be changed, and permits delivery in O2.87 Use of a patient mouthpiece, where possible, increases efficiency.94
Newer nebulizer devices that coordinate drug delivery with inspiration are now available.97 pMDIs and nebulizers can be used to deliver drugs during anesthesia via breathing system and airway devices (e.g., an ETT).98 Use in this setting is highly inefficient with, at best, only 10% of the administered drug reaching the lungs.99 Most of the drug is deposited in the tubing or airway, termed rain out.100 Delivery can be somewhat increased by actuating the pMDI during inspiratory flow,99 prolonging inspiration, increasing VT, and using wider ETTs.101,102 For anesthesia, nebulization into the breathing system is superior to pMDI use.102 Blockage of tubes by water lock must be avoided.
Parenteral administration is reserved for severe and emergent treatment.
B Drug Treatments for Asthma
Drug treatment for asthma is complex and changing. Currently, drugs are termed relievers, which provide symptomatic relief with β-adrenoceptor agonists (BA), or controllers (preventers), which reduce risk of disease exacerbation, such as GCs. Apart from the isolated use of a BA for symptom relief, combinations of these agents are used, with a variety of dose regimens and schedules. A stepwise approach is advised,45–47 as outlined in Table 6-2. A suggested response to asthma exacerbation, with severity estimated by peak expiratory flow, is shown in Table 6-3.
Asthma Treatment Step | Drug Therapy |
---|---|
1. Mild intermittent asthma | SABA ± chromone |
2. Regular controller therapy | SABA with IGC |
3. Add-on controller therapy | LABA with IGC (LM and/or MX) |
4. Therapy for persistent poor control | Increase IGC (increase LABA or add oral BA and/or LM and/or MX) |
5. Therapy with continual or frequent systemic GC | Increase IGC (add alternative agents) |
BA, β-agonist; GC, glucocorticoid; IGC, inhaled glucocorticoid; LABA, long-acting β-agonist; LM, leukotriene modifier; MX, methylxanthine; SABA, short-acting β-agonist. For drugs listed in parentheses, consider addition of one or more agents.
TABLE 6-3 Drug Therapy for Asthma Exacerbation
Phase | Severity/Response | Treatment Options |
---|---|---|
A. First hour | If PF >60% | O2 (aim for SpO2 >90%), I SABA continuous |
If PF <60% | Move to phase B | |
B. Next hour | Moderate PF 60-80% | O2, I SABA, I AC, O GC |
Severe PF <60% | O2, I SABA, I AC, O/IV GC, IV Mg | |
C. Thereafter | Good PF >70% | O2, IV SABA, I AC, O GC |
Incomplete PF <60% | O2, I SABA, I AC, IV Mg, IV GC | |
Poor PF <30% | O2, I SABA, I AC, IV Mg; consider adding IV MX, IV SABA, IPPV |
PF, Peak expiratory flow rate; I AC, inhaled anticholinergic; I SABA, inhaled short-acting β-agonist; IPPV, intermittent positive-pressure ventilation; IV GC, intravenous glucocorticoid; IV Mg, intravenous magnesium; IV MX, intravenous methylxanthine; IV SABA, intravenous short-acting β-agonist; OGC, oral glucocorticoid; SpO2, oxygen saturation by pulse oximetry.
For sustained control of asthma, a high degree of patient compliance is needed, which is problematic when the patient is very young or mentally or physically infirm. Indeed, the most common cause of “failed” treatment results from poor compliance or administration.103
Drug therapy for asthma is mainly based on modifications of natural substances that have been used since antiquity.104 This section provides a description of the drugs used, followed by consideration of drug therapy for an additional and critical asthma-related state, intraoperative bronchospasm.
1 β-Adrenoceptor Agonists
The medicinal plant, Ephedra, yields ephedrine, which has been used in China as Ma Huang for more than 5000 years. Inhaled ephedrine was reported as a bronchodilator in 1910.105 The in vitro bronchodilator effect of epinephrine (adrenaline) was reported in 1907, and it was first administered in clinical use as a nebulized solution by Percy Camps.104
Ahlquist demonstrated a rank order of potency for sympathomimetic agents, distinguishing α- from β-receptors, with further division into β1 (heart) and β2 (lung) receptors.106
Albuterol (salbutamol) was the first selective β2-agonist, and it remains the most commonly used bronchodilator worldwide.107,108 It is formulated as a racemic mixture of R and S forms. It has 29 times greater selectivity for β2– than β1-receptors. Subsequent side-chain modifications of the parent molecule have resulted in longer-acting agents, such as salmeterol and formoterol.104
a Pharmacodynamics
The therapeutic respiratory action of BA involves binding to membrane (β2-subtype) adrenoreceptors on smooth muscle cell in the lung, which stimulates production of the second messenger catalyst, adenylate cyclase, and increases the intracellular concentration of cAMP.109 The exact mechanism of action of cAMP remains unclear, but it probably decreases the intracellular calcium concentration and directly affects systems regulating the cellular contractile apparatus.110,111 These effects produce bronchodilation. In addition, BA may also provide beneficial effects by modulating neurotransmission in the cholinergic and perhaps other neural systems, affecting the function of inflammatory cells, modulating bronchial blood flow, stimulating mucociliary transport, and influencing the composition of mucus secretions.112 BA activity is enhanced by GCs.113,114
Duration of Action
One classification of BAs is by duration of action. Short-acting agents (<4 hours) are typified by isoproterenol (isoprenaline), which can be inhaled as a nebulized solution or given via pMDI. When it is nebulized, the adult dose is 0.5 mL of a 0.5% solution diluted in 2.5 mL of water. IV administration is not advised because of cardiovascular complications.115
The superiority of the R-isomer of albuterol (levalbuterol) compared to racemic (R and S) albuterol is controversial.116 Some studies report that levalbuterol is as effective as racemic albuterol with less tremor and tachycardia, whereas others do not support this conclusion.117–120
Terbutaline is available as an aerosol inhaler or in tablets. Inhaled terbutaline is clinically equal to inhaled albuterol.121 In the United States, it is the only selective β2-agonist available for parenteral use, with the usual subcutaneous dose similar to that of epinephrine (0.25 to 0.5 mg SQ). When used in this way, its duration of action exceeds that of epinephrine, and it may have cumulative effects on repeated administration. The elimination half-life is approximately 6 hours. About 40% is converted in the liver to a sulfate conjugate. Both conjugate and unchanged drug are excreted by the kidneys.
Pirbuterol and metaproterenol also have intermediate duration of action.
Long-acting BAs (10 to 12 hours) include salmeterol and formoterol. These agents are now combined with an IGC and are administered twice a day for long-term control of asthma symptoms. The duration of action remains unexplained.122 Bambuterol is the pro drug for terbutaline; it is activated by plasma cholinesterases, giving a longer duration of action. Use of long-acting BAs as monotherapy leads to tolerance or desensitization and a small increased risk of death.123,124 Use of BAs alone is now contraindicated.46
An ultralong-acting BA (24 hours), indacaterol, was recently described.125
Epinephrine (adrenaline) is a potent inotrope and vasopressor with actions at α-, β1-, and β2-receptors. It can be given subcutaneously, intravenously, or via a pMDI or nebulizer. Nebulized epinephrine is not superior to other inhaled BAs.126 The onset of action is rapid, making it often the first parenteral drug administered in an ambulatory emergency setting, to provide acute therapy until long-term measures can be instituted. The usual SQ dose is 0.4 mL of a 1 : 1000 solution in adults (0.005 mL/kg in pediatric patients), which may be repeated at 15-minute intervals. Side effects include hypertension, tachycardia, dysrhythmias, hyperglycemia, and acidosis. An IV dose regimen is described in the section on reactive management.
Adverse Effects
Side effects complicate BA therapy. Acutely, they include dose-related tremor, nervousness, nausea, hypertension, tachycardia, and dysrhythmias. These effects are related to stimulation of adrenergic receptors in the cardiovascular system and are more prominent with mixed agonists, although they may also occur with selective BAs, especially at higher doses. Side effects of high-dose BA therapy include hypokalemia, which is increased with use of GCs and methylxanthines (MXs). Lactic acidosis often develops; it results from increased lipolysis and leads to liberation of free fatty acids, which inhibit oxidative metabolism.127 MXs and GCs enhance β-receptor sensitivity to agonist activation and indirectly promote lactate production.128 For chronic use, there are concerns regarding the safety of BA in the longer-term treatment of bronchospasm.129
Despite use of antiasthma medications in the last decades of the 20th century, the morbidity and mortality associated with asthma increased—a situation referred to as the asthma paradox.130 Increased use of BAs has been implicated as one of the factors responsible. Studies have shown that therapy solely with β2-agonists over treatment times ranging from 15 days to 1 year may actually enhance airway reactivity.131–133 Others have found that regular use of inhaled BA bronchodilators is associated with an increased risk of death or near-death in asthmatics.134,135
It has been suggested that the chronic use of BAs may relieve symptoms without treating the underlying chronic inflammatory process that is apparently responsible for asthma.130 This process can lead to irreversible thickening of the airway wall that ultimately worsens hyperreactivity. However, others have questioned this association.136
c Utility
BAs are available in a variety of formulations, such as DPIs, nebulizer solutions, pMDIs, oral liquids, tablets, and IV preparations. BAs are used for reliever therapy by inhalation at all asthma treatment steps45–47 (see Table 6-2). For step 1, use of an inhaled short-acting β-agonist (SABA) may be the only treatment needed. If two or more aerosol inhaler canisters are used per month, escalation to step 2 is advised.46 For steps 2 and above, BAs are used as add-on therapies in combination with other agents.
Inhaler combinations of long-acting BAs with IGCs are often highly effective and may show synergistic activity.137 These are now considered to be the most effective therapy available.104
BAs have utility for emergency treatment of asthma exacerbations of varying severity. Again, other agents should also be used, particularly a course of systemic GC, which is usually given by the oral route (50 mg/day prednisolone, or equivalent, for an adult) for 5 days without tapering the dose.47,138,139 Nebulized ipratropium can also be added.140,141
For urgent cases, inhaled administration of BA is the reasonable choice, primarily with repeated doses of pMDI administered via a holding chamber, escalating to nebulized therapy. Repeated treatment at intervals of 15 to 30 minutes with doses of 5 to 10 mL/hr may be needed for adults.47
For severe acute asthma, administration of BA by continuous nebulization has efficacy equal to that of intermittent administration, with less tachycardia and hypokalemia.142 IV BA is reserved for life-threatening asthma, usually together with nebulized BA.47
Sellers and Messahel used rapidly repeated IV salbutamol (albuterol) boluses for emergency treatment of acute severe asthma in seven patients (children and young adults).143 All patients had received nebulized BA and systemic GC, and additionally two had received aminophylline by infusion. The dosage scheme was 5 µg/kg IV for children (250 µg for adults) given over 1 to 2 minutes, repeated every 2 minutes. The number of boluses given ranged from 2 to 7, and all were administered within 30 minutes. All patients showed clinical benefit.
An alternative to repeat bolus therapy was reported by Browne and Wilkins.144 They used a single bolus of salbutamol 15 µg/kg (over 10 minutes), followed by an infusion of 1 to 5 µg/kg/min. For refractory cases, IV epinephrine, 0.35 to 0.7 mg/kg, may be given over 5 minutes, followed by an infusion of 0.5 to 2 µg/kg/min.
For the treatment of severe acute asthma in the emergency setting, the addition of MX to BA therapy is not generally advised.145,146 A therapeutic response to MX in this setting is rare.4
2 Glucocorticoids
Solis-Cohen, a Philadelphia physician, demonstrated that “adrenal substance pills” were helpful in asthma.147 This effect was originally attributed to epinephrine (adrenaline), but oral preparations of natural catecholamines (e.g., epinephrine) are inactivated in the gut, so it is likely that GCs were the effective components.104
Kendall and Reichstein independently isolated and synthesized cortisol (and adrenocorticotropic hormone [ACTH]), receiving the Nobel Prize (with Hench of the Mayo Clinic) for this work in 1950.150 Boardley and colleagues reported rapid improvement in five asthma patients receiving ACTH,148 and soon oral cortisone treatment gained widespread popularity for management of a wide variety of inflammatory illnesses, including asthma.149 However, systemic side effects were common, and inhaled cortisone was ineffective. After concerted efforts, a range of topical GC preparations were introduced for application to the skin (for eczema) and to the lungs. Topical efficacy, on either skin or lungs, is related to skin blanching, now called the MacKenzie test.150
The introduction of inhaled beclomethasone diproprionate, an effective drug with a high therapeutic index, heralded the era of IGC as the mainstay for asthma control.151
a Pharmacodynamics
GCs are anti-inflammatory agents that act mainly through alteration of nuclear DNA activity, a genomic effect by which the expression of target genes is activated or repressed. This is a highly complex and coordinated set of processes involving the production of an array of inflammatory and anti-inflammatory enzymes, receptors, cytokines, chemokines, and adhesion molecules.152,153
The overall effect of GC action is decreased inflammatory cell recruitment, survival, accumulation, and activity.154,155 This takes hours to days to achieve. There are two types of GC receptors (α and β); both are intracellular. GC action is mediated by the α variant and inhibited by the β. GCs bind to the cytosolic α variant (not the β), with subsequent translocation of the complex into the nucleus, dimerization, and binding to specific domains of DNA—the glucocorticoid response elements (GREs) within promoter genes. The binding may result in activation or suppression of target gene transcription. Activation typically results in transcriptive and translative activity producing anti-inflammatory proteins.156
Suppression of gene activity results in decreased expression of inflammatory genes (switching off). This is achieved by action of the GC-receptor complex to activate histone deacetylase, an enzyme that removes acetyl groups from histone proteins involved in packing DNA. Deacetylation alters histone conformation, closing the associated DNA sequences and rendering the DNA less accessible for transcription.157
The β form of GC receptor (which does not bind with GC) can also bind to GREs, and this may provide an inhibitory pathway for overall GC activity.158 Additionally, GC may enhance degradation of messenger RNA (mRNA) coding for inflammatory proteins, a post-transcriptional mechanism of anti-inflammatory regulation.159,160
GCs also enhance the actions of BA (and MX), explaining the synergistic therapeutic effects of these agents.137 This is achieved by increased transcription of the β2-receptor genes,161,162 protection from β2-receptor downregulation, and prevention of uncoupling of β2 receptors from their G proteins (which is promoted by some inflammatory mediators, such as IL-1), thereby sustaining β2 activity.163,164 Reciprocally, BAs may increase GC activity by increasing translocation of GC receptor complexes into the nucleus.165
GCs have other nongenomic effects. These actions are rapid and are insensitive to blockers of gene transcription (e.g., actinomycin D) or protein synthesis (e.g., cyclohexidine).159,166,167 These nongenomic actions have therapeutic import. Asthma is associated with an increase in pulmonary blood flow, and this may interfere with airflow63,64; IGCs reduce this effect.168,169 Additionally, reduction in lung hyperperfusion may prolong the bioavailability of inhaled BAs, which are cleared from the lung by the pulmonary circulation.170
Side effects of GC therapy are well known. Systemic therapy risks dose-dependent suppression of the hypothalamopituitary axis, osteoporosis, osteonecrosis, systemic hypertension, diabetes mellitus, obesity, skin thinning, myopathy, cataracts, and glaucoma.46 For those patients who need systemic steroids to achieve asthma control (step 6), these complications are a serious risk, and this is the rationale for adding steroid-sparing therapies as outlined previously.
Inhalation therapy markedly reduces systemic toxicity but does not eliminate it.171 IGC can be absorbed in two ways—from the lungs or after swallowing oropharyngeal deposited drug. Side effects are usually apparent with large doses (>400 µg/day budenoside or equivalent in an adult). Easy bruising, adrenal suppression, cataracts, and glaucoma have been described.46 Local toxicity results in sore throat with oral candidiasis.90
b Pharmacokinetics
With optimal technique, IGCs achieve rapid and efficient delivery to the airways. With poor technique, however, drug is deposited in the oropharynx, where it can be a local irritant or be swallowed.90
c Utility
IGCs are used as controller (preventer) medications at steps 2 through 4, and systemic (oral) GCs are used for step 5 (see Table 6-2). IGCs are currently the most effective anti-inflammatory therapy available. They reduce symptoms of asthma,172 improve lung function172 and quality of life,172 and reduce airway reactivity.172,173 The frequency and severity of exacerbations are reduced,174 as is mortality.175
A wide variety of IGCs are available, either as a single formulation (pMDI or DPI) or in combination with a long-acting BA. Examples include beclomethasone (beclometasone) diproprionate, budenoside, fluticasone propionate, and mometasone furoate. Ciclesonide is a pro-drug that is activated in the lung by ester hydrolysis; its use aims to reduce oropharyngeal irritation and systemic uptake of swallowed drug. The variety of IGCs has led to advice on standardization of IGC dosage as “budenoside equivalents” in low, medium, and high daily dose regimens,46 as shown in Table 6-4.
Systemic GCs include prednisone, prednisolone, methylprednisone, methylprednisolone, betamethasone, dexamethasone, and hydrocortisone. These may be given by oral, IV, or intramuscular (IM) routes, with similar efficacies. Deflazacort and cortisone acetate are formulated for oral administration, and triamcinolone is reserved for intra-articular or IM use. The relative potencies of these drugs are shown in Table 6-5.
Glucocorticoid | Equivalent Dose |
---|---|
Prednisolone | 5 mg |
Betamethasone | 750 µg |
Cortisone acetate | 25 mg |
Deflazacort | 6 mg |
Dexamethasone | 750 µg |
Hydrocortisone | 20 mg |
Methylprednisolone | 4 mg |
Triamcinolone acetate | 14 mg |
Oral GCs are used for treatment of exacerbation. Systemic drug should be given for “all but the mildest exacerbations”46 for 5 days and may be stopped without tapering.46 Oral GCs are usually effective but take at least 4 hours to produce clinical improvement. A usual single daily dose of methylprednisone, 60 to 80 mg, or hydrocortisone, 300 to 400 mg, in divided doses is advised.46
The rapid nongenomic effect of IGC in reducing lung hyperemia may have a therapeutic role in the treatment of exacerbation.176,177 For example, one study showed equal efficacy of 3 mg nebulized fluticasone and 100 mg hydrocortisone in nonintubated patients with acute severe asthma.178
3 Methylxanthines
The MXs are a group of phosphodiesterase inhibitors. They include caffeine, present in coffee, and theophylline and theobromine, present in tea. Extracts have been used historically for treatment of respiratory disorders. In 1886, Henry Hyde Salter, a family physician in London, reported that drinking strong coffee on an empty stomach eased his asthma.179 The solubility of MXs is low and is enhanced by the formation of complexes with other compounds; for example, aminophylline is a complex of theophylline and ethylenediamine. Before the widespread adoption of BA therapy, IV administration of a soluble MX was the standard first-line treatment for severe asthma.104 Other preparations, such as salts of theophylline (e.g., oxytriphylline) and covalently modified derivatives (e.g., dyphylline), are available.
a Pharmacodynamics
MXs have multiple mechanisms of action, and the effects of clinical importance remain controversial.180,181 Originally, they were thought to act as phosphodiesterase inhibitors. Phosphodiesterases are a group of enzymes, one action of which is inactivation of cAMP, the second messenger for adrenoceptor activation. Such inhibition increases intracellular cAMP, thereby enhancing adrenoceptor activity and resulting in bronchodilation.182
The phosphodiesterase isozymes 3 and 4 are implicated,104 but the drug concentrations needed to demonstrate this effect in vitro may exceed those present at therapeutic levels in vivo.183 Moreover, not all phosphodiesterase inhibitors are effective in asthma, and theophylline-induced relaxation of airway smooth muscle in vitro occurs without changes in intracellular cAMP levels.184,185 Other mechanisms demonstrable in laboratory preparations, including antagonism of adenosine and stimulation of endogenous catecholamine release, also do not appear to be significant to the clinical action of theophylline.186,187
Some of the therapeutic actions of MXs may result from effects other than relaxation of smooth muscle. These drugs may improve mucociliary clearance, stimulate ventilatory drive,184 and increase diaphragm contractility,188,189 actions that may be beneficial in patients with reactive airways disease. MXs also have significant cardiovascular effects, including direct positive chronotropic and inotropic effects on the heart, reductions in preload and afterload, and diuresis, which may be beneficial in patients with cardiovascular disease.
There is increased evidence to support anti-inflammatory and immunomodulatory roles for MXs in asthma.190,191 Theophylline increases the activity and number of suppressor T cells and reduces the activity of many inflammatory cells implicated in asthma.192,193 More recently, MXs have been shown to stimulate histone deacetylase. The action of this nuclear enzyme results in reduced exposure of DNA elements to transcription, which may render inflammatory genes less active, an effect that is synergistic with GC.194
Because MXs have multiple systemic actions, side effects are common, mainly involving the CNS and the cardiovascular system.195 CNS effects include stimulation, insomnia, and tremor, leading to convulsions at toxic plasma levels (considered to be >20 µg/mL). For the cardiovascular system, toxic levels may produce ventricular and atrial dysrhythmias. In addition to phosphodiesterase inhibition, adenosine receptor activation may be important.104 Gastrointestinal disturbances ranging from epigastric discomfort to nausea and vomiting may also occur.
b Pharmacokinetics
All MXs are eliminated primarily by hepatic metabolism. Plasma clearance varies widely even among healthy subjects, with the elimination half-life ranging from about 3 hours in children to 8 hours in adults.196 The hepatic cytochrome P450 enzyme group (particularly CYP 1A2) clears MX. The activity of these enzymes may be enhanced, for example by smoking and by concurrent therapy with carbamazepine or rifampicin, leading to greater clearance. Conversely, the enzymes may be inhibited by drugs such as cimetidine and ciproxin, resulting in greater MX bioavailability. Disease states such as liver or cardiac failure alter clearance. Unpredicted changes in clearance may result in toxicity in critically ill patients, so measurement of plasma levels is important.
Regardless of the preparation chosen, plasma concentrations of theophylline should be monitored to ensure that levels are in the therapeutic range (5 to 20 µg/mL). For children receiving less than 10 mg/kg/day, monitoring is not considered necessary.46
c Utility
With other therapeutic advances in the pharmaceutical treatment of asthma, some have questioned the continued role of MX in the management of reactive airways.197 However, MXs still have a number of therapeutic roles, and it may become more popular again with increased recognition of the immunomodulatory and anti-inflammatory properties of these drugs.198–200
There are presently three main indications for MX use. First, for the relatively small group of patients who are unable to manage inhaler therapies, MXs may be used as primary controller therapy.46
Second, MXs have utility as add-on controller therapy to IGCs,201–203 although the therapeutic effect is usually less than that achieved by adding long-acting BAs to IGCs.204,205 When properly used, these drugs remain safe and effective for the chronic management of asthma and in some patients with chronic obstructive pulmonary disease (COPD).206 With effective clinical support and plasma drug monitoring, adverse incidents are rare. One study of 36,000 patients receiving 225,000 prescriptions over 9 years reported that the incidence of hospital admission resulting from MX toxicity was less than 1 per 1000 patient-years.207
Third, for patients with severe exacerbation of asthma whose management is problematic, add-on therapy with IV aminophylline may be considered. This is not generally advised for all patients, and response to treatment in this situation is described as rare.47,146 The initial loading dose is 5 mg/kg, administered over 30 minutes to minimize toxicity. This is followed by an infusion of 0.5 to 0.7 mg/kg/hr, which provides therapeutic levels in most patients. This loading dose and rate may need to be increased in smokers or decreased in severely ill patients and in those with liver disease or congestive heart failure. All dose recommendations are guidelines, and patients must be monitored with plasma theophylline concentrations (daily for the emergency patients).47
4 Leukotriene Modifiers
In 1938, Feldberg and Kelloway isolated substances with bronchoconstrictive and vasoactive properties from guinea pig lung perfused with cobra venom.208 In 1960, these substances were shown to be released during anaphylactic shock and were named slow-reacting substance of anaphylaxis (SRS-A).209 In 1979, Murphy and colleagues identified SRS-A as a mixture of lipids, later called leukotrienes—so named because they are produced by leucocytes and posses a carbon chain with three double-bonds.104,210 Leukotrienes were later shown to have potent bronchoconstrictor properties when inhaled by asthmatics.211 Inhibitory agents affecting this metabolic pathway, the first new class of drugs for asthma therapy in 30 years, were introduced into clinical practice in the 1990s.
a Pharmacodynamics
The cysLTs are formed in a sequential pathway involving initial conjugation of LTA4 with the tripeptide glutathione to form LTC4, which is exported out of the cell, where it rapidly changes to LTD4 (by loss of a glutamyl residue) and to LTE4 (by loss of a glycine residue). A number of variations in the enzymatic pathway exist. Genetic variation, or polymorphism, has been associated with aspirin-induced asthma (AIA).212
The cysLTs bind to a number of transmembrane-spanning G protein–coupled receptors found on a variety of leucocytes, smooth muscle cells, glandular epithelial cells, and endothelial cells. The target receptors are called cysLT1R and cysLT2R. Activation of cysLT1R (in the potency order LTD4 > LTC4 > LTE4) results in bronchoconstriction, edema, and glandular secretion.213–215 Activation of cysLT1R on fibroblasts may promote proliferation, a possible factor in airway remodeling and inflammatory cell recruitment.216 CysLT2R activation has effects on endothelial permeability and leucocyte activity.217,218
LTB4 binds to the receptors BLT1R and BLT2R. BLT1R activation has been implicated in stimulation of T lymphocytes and mast cells.219,220
LMs are generally well tolerated, the most common complaints being abdominal discomfort, muscle weakness, and pain. Initial concerns that montelukast therapy was associated with mental disorder and suicidal ideation have not been supported.221 Zileuton has been associated with liver toxicity, and liver function monitoring is advised.222 There is an apparent association of LMs with Churg-Strauss syndrome,223 but this may be related to the drugs’ ability to spare steroid.224,225
c Utility
LMs may be used as controller medications in conjunction with other agents. They have a small, variable bronchodilator effect with anti-inflammatory activity and may reduce asthma exacerbations.226–228 They may reduce cough229 and may be useful for symptom control in viral-associated bronchospasm230–233 and AIA.234–236 When used alone as controller therapy, LMs are usually less effective than IGCs,237 but when used in conjunction with IGCs, they can provide a steroid-sparing effect.238
LMs are not effective for management of acute asthma. A multicenter, randomized trial of high-dose zafirlukast performed in an emergency department showed only a small reduction in admissions in the treatment group.239
5 Anticholinergic Agents
Anticholinergic drugs are bronchodilators, pulmonary vasodilators, and inhibitors of tracheobronchial secretion. They lack anti-inflammatory action.104 They also decrease salivary, gastric, and intestinal secretions. The parent agent is atropine. Other tropane alkaloids include hyoscine, ipratropium, oxtropium, glycopyrrolate, and tiotropium.
a Pharmacodynamics
Parasympathetic vagal efferent (autonomic motor) nerves form ganglia around large- and medium-diameter airways.240,241 Postganglionic fibers supply bronchial smooth muscle, blood vessels, and mucus glands. Muscarinic receptors are located on the target cell membrane and are G protein–coupled receptors. Three types of muscarinic receptors are reported: M1, M2, and M3.242–244
M3 receptors are located at postganglionic terminals at the effector sites; antagonism of their effects may be beneficial. The overall effect of MRAs depends on the balance of receptor activation, and those drugs with M3 selectivity (e.g., tiotropium, glycopyrrolate) should therefore have greater utility.104
b Pharmacokinetics
Ipratropium bromide is a quaternary ammonium derivative of tropane and is positively charged at physiologic pH. It is poorly absorbed from the lungs (<1%), does not readily cross the blood-brain barrier, and can be administered by inhalation at high dose and with minimal systemic side effects.245,246 This and similar agents have slow onset times, up to 90 minutes.247,248 Tiotropium, although not licensed for use in asthma, has a long duration of action, up to a week.104
c Utility
Ipratropium is a clinically useful bronchodilator, but its slow onset limits efficacy as reliever medication, and BAs are first-line agents. Ipratropium may be useful as additive step-up therapy for patients with moderate impairment (FEV1 50% to 70% predicted).45 Ipratropium is available as a pMDI (18 µg/puff). The usual dose is 2 puffs given four times daily. For patients with COPD that includes a reversible component of bronchospasm, nebulized ipratropium may have benefits over BAs, including a longer duration of action and efficacy in some patients in whom BAs have little effect.249
In severe asthma, a combination of nebulized ipratropium and BA is superior to BA alone for adults and children.250–252 A meta-analysis of 16 randomized, controlled trials comparing salbutamol and ipratropium versus salbutamol and placebo, showed reduction in hospital admission with improved lung function.253 Multiple doses of ipratropium are superior to single use.254
Ipratropium may also be useful for treatment of bronchospasm induced by beta-blockers.250 Tiotropium bromide is currently approved for bronchodilator therapy in COPD but not in asthma. In a recent trial in patients with moderately persistent asthma, tiotropium was reported to be equivalent to either doubling of the dose of inhaled beclomethasone or addition of inhaled salmeterol.255 This finding shows therapeutic promise.
6 Chromoglycates (Chromones)
Cromolyn sodium, disodium cromoglycate, and nedocromil sodium are chromoglycates (chromoglicates) or chromones; they are clinically used as controller drugs with modest efficacy.46 The parent compound, khellin, is derived from the medicinal plant, Amni visnaga, which is found around the eastern Mediterranean. They are useful only in the prevention of bronchospasm, not treatment.
a Pharmacodynamics
Cromolyn contains one chromone ring, nedocromil two. They are often called chromones, but nedocromil is structurally termed a pyranoquinolone. The first drug, chromolyn, was marketed as Intal (inhibitor of allergy).256 Chromolyn and nedocromil inhibit release of inflammatory mediators from various cell types associated with asthma, especially lung mast cells, particularly in response to airway irritants such as cold air or sulfur dioxide.257,258
The mechanism of action is not fully known, but two actions are proposed104: phosphorylation of a moesin-like membrane protein (a natural terminator of granule release) and inhibition of chloride-channel opening in response to mechanical (structural or osmotic) stresses imposed on mast cells.74,259
c Utility
Chromones are controller drugs, useful both as a single preventive treatment, when taken 15 minutes before exercise or exposure to antigens, and as therapy as an alternative to IGCs.45,260,261 Efficacy in individual patients varies widely, and at present a therapeutic trial is the only way of determining which patients will benefit. The two drugs are equally effective in clinical use.258 Neither agent has been studied as an adjunct to airway management in the perioperative period.
7 Alternative Agents
For refractory asthma, with relapse despite maximal therapies resorting to chronic or multiple courses of systemic GC, immune-suppressant and immune-modulating drugs have been used, as have novel inhalation therapies. These drugs may allow reduction of the systemic steroid dose, but they are generally limited by modest and variable response, by side effects such as opportunistic infection, and by uncertainty over long-term safety. In general, a 3- to 6-month trial is advised.262,263 For some agents, treatment numbers have often been small and study design may be questionable.
Anti-IgE therapy with omalizumab is an option for those patients who need chronic systemic GC or multiple rescue courses.264 Omalizumab is generally well tolerated.265 In the United Kingdom, serum IgE levels higher than 700 IU/L need to be demonstrated.47
Methotrexate given for 3 months allows a 20% reduction in systemic steroid dose for about 60% of patients, but the risk-benefit ratio does not usually support its use.266–268 Opportunistic infection is a risk, and there have been reports of Pneumocystis pneumonia.266,269
Cyclosporine, a lipophilic cyclic 11-residue peptide, also has steroid-sparing effects in about half of patients with severe asthma. The primary target is T cells. Cyclosporine binds to intracellular cyclophilins, forming a complex that inhibits a calcium/calmodulin-dependent phosphodiesterase and reducing transcription of a group of nuclear family proteins. This results in decreased cytokine production, particularly IL-2, but also IL-4 and IL-5. Cyclosporine may also promote apoptosis (cell death) of CD4+ T cells.270 IL-1 production from monocytes and macrophages is also reduced.
A systematic review (of three trials) showed that cyclosporine reduces daily steroid use by 1 mg or less.271 In one study of five children taking more than 10 mg of prednisone daily, cyclosporine allowed weaning of steroid in three children and small improvements in lung spirometry.272 Cyclosporine has a narrow therapeutic index, and drug level testing is needed. Opportunistic infection, renal injury, and hypertension are the main side effects.
Tacrolimus is a fungal macrolide that has effects similar to those of cyclosporine.
Macrolide antibiotics have antimicrobial activity and also anti-inflammatory actions through reduction in the expression of IL-8, a neutrophil activator. Some asthma patients may be chronically infected with Chlamydophila or Mycoplasma susceptible to macrolide treatment.273–275 In addition, some patients with severe asthma that is resistant to conventional treatment may have a neutrophilic disease process (as opposed to increased eosinophil activity) and may benefit from macrolide therapy.276 However, a systematic review of the use of macrolides (clarithromycin, troleandomycin, and roxithromycin) in asthmatic patients and did not find enough evidence to form a meta-analysis.277 Liver toxicity is a risk.
Gold therapy results in a small reduction in systemic steroid use. Proteinuria is a side effect.278
Colchicine is used to treat gout and familial Mediterranean fever with few side effects. A prospective, multicenter study of its use in patients with asthma did not show benefit of this drug over placebo.279
Hydroxychloroquine has established uses as an antimalarial agent and for connective tissue disease management. Retinopathy is the major risk. Its efficacy in asthma management is controversial, with conflicting reports of efficacy.280,281
Dapsone is an anti-inflammatory agent that is used in leprosy treatment. One small study showed therapeutic promise in asthma treatment, with a large steroid-sparing effect but complicated by anemia.282 There are currently no published data from well-designed trials, leading to a conclusion that strong evidence supporting its use in asthma is lacking.283
Intravenous immunoglobulin has anti-inflammatory actions, but large trials have not supported its use in asthma, and side effects such as fever and urticaria are common.284,285
Antifungal therapy is another potential treatment of asthma. Environmental fungal exposure is virtually ubiquitous. Many asthma patients show IgE responses (sensitization) to common fungi such as the dermatophyte Trichophyton. This may be incidental to the overall disease process for asthma, or it may in certain patients be a major pathologic problem, called severe asthma with fungal sensitivity (SAFS).286 In general, asthma severity is associated with specific IgE responses to Trichophyton; a trial in 2009 showed modest spirometric improvement during long-term antifungal treatment,287 but this was not sustained on discontinuation of antifungal therapy.288
One disease, allergic bronchopulmonary aspergillosis, is characterized by lung colonization and major allergic response to the common fungus, Aspergillus fumigatus. Many of these patients have an asthma-like disease. Itraconazole is an effective antifungal treatment.289
Tumor necrosis factor α (TNF-α) is implicated in asthma.290 Anti-TNF-α agents include etanercept, a soluble TNF-α receptor fusion protein that binds to TNF-α, as well as infliximab and golimumab, monoclonal antibodies that bind to TNF-α. Trials with etanercept,291 infliximab, and golimumab have not supported their use.292,293
Interleukin antibody therapy is another potential treatment of asthma. IL-2 and its receptor, IL2R, are produced by Th2 cells in response to allergen exposure and stimulate sensitized Th2 proliferation. Monoclonal antibody to IL2R (daclizumab) inhibits this response. Intravenous daclizumab therapy resulted in small improvements in asthma control.294
IL-5 promotes eosinophil activation, and this is an important part of the disease process for a group of patients with eosinophilic asthma.295 In this group, treatment with antibody to IL-5 (mepolizumab) has been shown to have clinical utility,296 but not in the general asthma population.297
Heparin has anti-inflammatory and anti-eosinophilic properties.298 Nebulized heparin may have therapeutic promise for asthma treatment.299 Coagulopathy does not complicate therapy.
Nebulized lidocaine also has potential,300 but the therapeutic effect remains controversial.301
The 2007 Expert Panel Report of the National Asthma Education and Prevention Program gave recommendations for use of alternative agents.45 The report stated that “the data at present are insufficient” to recommend macrolide therapy. Moreover, the “current evidence does not support” the use of methotrexate, IL-4 receptor antibody, antibodies to IL-5 and IL-12, intravenous immunoglobulin, colchicine, troleandomycin, gold, or cyclosporine.
8 Investigative Drug Therapies
Investigative drug therapies provide an active and fast-changing area of research. It is uncertain which agents, if any, will be of practical patient benefit. As of early 2011, some drug targets include ADAM8 (a matrix metalloproteinase),302 peroxisome proliferator-activated receptors (PPARs),303 arginase,304 and adenosine receptor.305
Nucleotide synthesis inhibitors (mycophenolate, leflunomide, and brequinar) may have clinical utility. They interfere with DNA replication, and lymphocytes are considered to be relatively more vulnerable.262
9 Other Agents
The mucolytic N-acetyl-L-cysteine (NAC), inhaled or ingested, has no proven efficacy in the management of asthma (or COPD or cystic fibrosis).87 The aerosol form has a pH of 2.2, provokes airway secretions, and is an airway irritant.306
Other mucolytic agents have been used in the treatment of cystic fibrosis, including aerosol formulations of a DNase (dornase alfa) that hydrolyzes the DNA component of mucus. There are isolated reports of dornase delivered via a bronchoscope in status asthmaticus.307 A newer agent, thymosin-β4, has hydrolytic activity for the fibrous form of actin in mucus.65
Antihistamines do not have clinical efficacy for asthma treatment.46,104 It is ironic that the first isolated mediator in asthma, histamine,308 is not amenable to blockade.
C Anesthetic Management of Asthma
Given the worldwide prevalence of asthma, many patients present for surgery, planned or otherwise. A core feature of anesthetic practice is provision of airway control, often with instrumentation. Because asthmatic patients have, as a primary component of their disease, airway hyperreactivity, they are at risk for perioperative exacerbation, especially intraoperative bronchospasm (IOB). IOB develops when offensive factors (collectively termed noxious stimuli), such as airway instrumentation or surgery (e.g., incision, manipulation, traction) are greater than defensive factors provided by preoperative drug management or anesthetic technique. Surgery within major body cavities, especially cardiothoracic or abdominal surgery, is considered to pose a greater risk for IOB.46
Patients who do not have optimal control of their asthma and those who have a history of a previous perioperative exacerbation are also considered to be at risk for IOB.100 Conversely, those patients with optimal control are not considered to pose an extra risk.46
1 Proactive Management: Prevention of Bronchospasm
a Optimization
Smoking cessation 2 months before surgery is advised.309 Control of gastroesophageal reflux is advised.310 Patients with asthma have been reported to have three times the prevalence of reflux disease compared with patients without asthma. Long-term treatment, however, has not improved overall asthma control.311,312
b Preoperative Prevention
Systemic Glucocorticoids
A preoperative course of systemic GC therapy (oral prednisolone, 40 to 60 mg/day for 5 days, or IV hydrocortisone, 100 mg three times daily) is advised for those patients with an FEV1 less than 80% of predicted.309 Treatment can be stopped without tapering (weaning the dose).46
For patients who have received systemic GC within the previous 6 months, a course of supplemental GC is advised (e.g., IV hydrocortisone 300 mg/day in divided doses) before surgery of intermediate or major intensity.46 This can be stopped after 24 hours.313
Bronchodilators
Short-acting inhaled bronchodilators are also advised.309 pMDIs have efficacy equal to that of nebulized therapy provided technique is optimal. A preoperative combination of GC and BA is advised for patients with severe or brittle asthma and for those needing major surgery.313,314
GC and BA therapy, singly or combined, may result in electrolyte imbalances, particularly potassium and magnesium deficiencies. This should be anticipated and prevented by supplementation or corrected.309
Anxiolytics
Fear (and pain) can be potent triggers for bronchospasm. Effective treatment with anxiolytics reduces this risk. Benzodiazepines do not provoke bronchoconstriction. There are reports of either no alteration in bronchial tone or decreased tone caused by direct effects in the lung (mediated by γ-aminobutyric acid [GABA] receptors) or by indirect effects in the CNS.315–318
Kil and associates reported no clinically measurable effect of oral midazolam 0.5 mg/kg on cardiorespiratory function in treated children with mild or moderate asthma who were presenting for dental extractions.319 Benzodiazepines are therefore a reasonable choice. α2-Agonists (dexmedetomidine or clonidine) provide dose-dependent sedation and bronchodilation with drying. These agents have therapeutic appeal but have not been formally investigated in the setting of perioperative asthma management.100
Analgesics
Naturally sourced opiates, such as morphine, have a traditionally considered capacity to provoke mast cell degranulation, but strong evidence for consistently provoking bronchospasm is lacking. Whereas morphine provokes degranulation from skin mast cells, it does not do this for mast cells in the lung.320 Morphine may have bronchodilator effects in asthmatic patients.321
The use of nonsteroidal anti-inflammatory drugs (NSAIDs) for analgesia is traditionally controversial. They are generally considered safe to use in patients with well-controlled asthma and no known sensitivity.322 The notable exception is in patients with AIA. This is a clinical syndrome of asthma that is worsened with aspirin and nasal polyposis, termed Samter’s triad. Aspirin inhibits the cyclooxygenase pathway, diverting arachidonic acid metabolites into the lipoxygenase pathway and leading to an increase in cystLTs. Some estimate that up to 20% of asthmatics carry this risk.323 AIA is associated with cigarette smoking.324 Patients may have cross-reactivity with other NSAIDs, and referral to an allergy clinic has been advised.325
c Perioperative Prevention
A regional technique, either with sedation or with general anesthesia, may be appropriate. Systemic absorption (from a regional technique) of local anesthetic can attenuate bronchoconstriction. Shono and colleagues reported a case of a patient with asthma whose wheeze was reduced after extradural anesthesia with 2% lidocaine and returned after discontinuation of the drug.326
For general anesthesia, the principles of balanced anesthesia with hypnosis and reflex suppression apply. Induction is a time of risk. Combinations of synergistic agents, such as hypnotics with one or more opioids, benzodiazepines, α2-agonists, or ketamine, can provide a smooth and sustained induction profile. Rapid administration of opioids has been reported to provoke the so-called rigid chest, even at low doses; this may, in part, result from laryngospasm.26,327 These complications may be misinterpreted as bronchospasm. The antitussive effect of opioids is, however, reported to be therapeutic.314
Airway instrumentation is a risk factor for bronchospasm,328 particularly with endotracheal intubation.329 The effect of endotracheal intubation even in symptom-free asthmatics was shown in 10 volunteers who underwent the procedure with topical anesthesia. Intubation resulted in falls in FEV1 in up to 50% of the subjects. This fall was attenuated in subjects receiving inhaled BA.328 The response to mechanical stimuli is mediated by the parasympathetic nervous system and by direct local stimulation with release of mediators330 such as neurokinin A and substance P from peripheral nerve terminals of C-fiber afferents.331 Whereas endotracheal intubation is provocative, laryngeal mask airway insertion is not.332
Induction
Preoperative anticholinergic therapy may protect against bronchospasm, and nebulized therapy has been reported to have utility.333
Inhaled lidocaine can attenuate airway reflexes in asthmatics and normal volunteers receiving inhaled histamine, but its efficacy is controversial.300,301,334 A combination of topical lidocaine and BA has been used to attenuate reflexes for awake intubation in normal volunteers.329 Lidocaine inhalation may provoke minor airway irritation, such as cough, breath-holding, or, more seriously, bronchospasm,301,335–339 which can be attenuated with coadministration of IV lidocaine.340 Lidocaine-provoked airway irritability can be reduced by anticholinergic or BA treatment.336
Propofol has been shown to have bronchodilator properties in animals and isolated human airway preparations.22,341,342 Propofol is generally considered to be the most reasonable agent for induction,343 superior to thiopental or etomidate.20,344,345 Propofol modulates the slow inward calcium influx in smooth muscle and also reduces production of cytokines IL-1, IL-6, IL-8, and TNF-α. It is generally a bronchodilating agent,346–348 but there are isolated reports of bronchospasm developing in association with propofol.349 Formulations with preservatives are implicated, and those with irritable airways, such as smokers (and asthmatics), may be at greater risk.350
In contrast, thiopental may provoke bronchospasm when given to asthmatic patients. Wheeze was demonstrated in 48% of asthmatic patients receiving thiopental.351
Ketamine is a bronchodilator; it has a direct relaxant effect on airway smooth muscle through potentiation of catecholamines and inhibition of neutrally provoked bronchoconstriction.348,352 Ketamine has been advised as an induction agent for asthmatic patients. In a case series of 40 patients with medically controlled asthma who received induction with ketamine, 26 patients had reduced bronchospasm.353 For patients with severe asthma (persistent asthma or exacerbation) presenting for surgery, ketamine is advised as the hypnotic drug of choice for induction.354 A case series of 5 patients with status asthmaticus needing emergency intubation reported rapid improvement of arterial CO2 content after IV induction with ketamine.355
Inhalational induction is an option for motivated patients. Sevoflurane is a reasonable choice. An inhalation induction with either isoflurane or desflurane causes initial upper airway irritation and may provoke coughing, breath-holding, and laryngospasm.356
Lidocaine, given systemically before instrumentation, may be bronchoprotective. In asthmatic volunteers, IV lidocaine (up to 2 mg/kg) reduced histamine bronchoprovocation. Groeben and colleagues showed that either IV lidocaine or inhaled albuterol reduced histamine-provoked wheeze and concluded that both agents should be used before induction of anesthesia.357 This conclusion was not supported by another study of 60 asthmatic patients,358 and bronchospasm after IV lidocaine has been reported.43
NMB agents without bronchoconstrictive properties should be chosen. Release of mast-cell histamine has been reported with atracurium, increasing airway resistance and provoking bronchospasm.359,360 Mivacurium is also implicated.361 Atracurium also enhances vagal activity, which may be involved in increases in bronchomotor tone.362
Suxamethonium increases airway resistance and sensitivity to acetylcholine in animal models.363,364 There are rare case reports of suxamethonium associated with bronchospasm and anaphylaxis.365–367
NMB drugs that have activity at the M2 receptor group, such as rapacuronium, pipecuronium, and gallamine, can provoke bronchoconstriction.309,368 Rapacuronium, initially a promising, rapidly acting blocker, was withdrawn because of this problem.369
Vecuronium, rocuronium, cisatracurium, and pancuronium are considered reasonable choices,370 but anaphylaxis371—albeit rare—is a risk.
Maintenance
Airway gases and vapors should be warmed and humidified. Volatile agents are usually used for the maintenance phase of anesthesia. In general, these agents depress contractility of the smooth muscle of the lower airways and are potent bronchodilators. They reduce responses to bronchoconstricting stimuli in both humans and animals10,372–376 and also reduce baseline airway resistance in animals.10,372–377 Effects on baseline airway resistance in human subjects are more difficult to interpret because of confounding influences such as endotracheal intubation and decreases in lung volume associated with general anesthesia, but it appears that these agents may also reduce resting airway smooth muscle tone in humans.378,379
Multiple mechanisms contribute to the relaxation of airway smooth muscle produced by volatile anesthetics attenuating reflex bronchoconstriction, in part by depressing neural pathways that mediate these reflexes.380–382
Bronchodilation has been observed with the use of high-speed computed tomography.383 The exception is desflurane, which lacks bronchodilator activity.384 Whereas sevoflurane is a popular choice for maintenance anesthesia, its bronchodilating efficacy is presently uncertain.
Rooke and coworkers compared the bronchodilating effects of sevoflurane, isoflurane, and halothane, concluding that sevoflurane reduced airway resistance more than the others did.385 However, Habre and colleagues studied the effect of sevoflurane anesthesia on children with or without asthma and concluded that respiratory resistance increased only in the asthmatic group386 and could be prevented by preoperative salbutamol (albuterol) therapy.387
Sevoflurane anesthesia (in pigs) may increase the production of inflammatory mediators such as LC4 and nitrogen oxides in the lungs.388 Because tone in airway smooth muscle helps regulate the matching of ventilation to perfusion, suppression of normal airway smooth muscle tone may contribute to impaired gas exchange in the perioperative period with all volatile agents. Animal studies have shown that sevoflurane anesthesia (in rats) may result in impaired gas exchange within the lung as a result of changes in peripheral lung compliance.389
Completion
Completion of surgery and anesthesia is a crucial time when airway reflexes return, although in a modified state. Removal of airway devices (especially ETTs), coupled with clearance maneuvers such as suction, is provocative. Options include airway interventions done during deep anesthesia, but these may compromise airway patency and protection and should be performed after a risk-benefit analysis. Changing an ETT for an SLA can permit satisfactory emergence conditions—the so-called bridge to extubation, described when one is weaning a patient with resolved status asthmaticus from invasive ventilator support in critical care.390,391 IV lidocaine may attenuate airway irritation at this stage.392
Reversal
Reversal of NMB with neostigmine (a cholinesterase inhibitor) has the potential to provoke bronchospasm by enhancing acetylcholine concentration. When it is given with antimuscarinic agents (atropine or glycopyrrolate), there is no reported effect on airway resistance.393 However, bronchospasm has been reported, and this is a risk for patients with renal impairment.100,394 Some authors advise allowing NMB to wear off without recourse to reversal agents.100,309 Sugammadex offers an alternative method for reversal of rocuronium.395
In the postoperative phase, warmed, humidified O2 therapy, physiotherapy, incentive spirometry, and motivated mobilization are important factors. Restoration of optimal drug therapy is also important. A course of nebulized SABA may be helpful. Temporary noninvasive ventilation has been described.100 Relief of pain and fear is a major goal.
2 Reactive Management: Treatment of Intraoperative Bronchospasm
IOB develops when the noxious stimuli of anesthetic or surgical techniques are not counteracted by planned protective anesthetic strategies. The reported incidence ranges from 1.7% to 16% of a general surgical patient population.100 Isolated IOB may be the only recognized manifestation of anaphylaxis; it has been reported in 19% of patients subsequently shown to have anaphylaxis.396 Recognized risk factors include reactive airways disease (asthma and COPD), tobacco smoking, and endotracheal intubation.397 A gradation of severity can occur, from mild and transient to severe and sustained with threat to life. Critical IOB is an emergency and mandates immediate treatment. This is equivalent to the intensive care scenario of status asthmaticus,346 from which much experience in management has been gained.
Treatment should be matched to severity, bearing in mind that the time course of IOB is variable and unpredictable. Escalation of drug therapies may be necessary. Rehearsed responses, with drugs available and doses calculated, are wise preemptive moves. A six-step reactive strategy outlines the necessary responses (Box 6-1).
a Recognition
Initial visible signs of IOB include rise in airway pressures, falls in delivered VT, and decreased chest movement. Auscultation may reveal noisy breath sounds with expiratory wheeze predominating, but when IOB is severe, these signs are lost: The “silent chest” is dangerous. Auscultation of the expiratory limb of the breathing system may be helpful if the patient is isolated from direct examination.100
Bronchoconstriction results in an increase in airway resistance. If this increase is sufficient to increase the overall expiratory time constant beyond the maximal expiratory time, the lung relaxation volume cannot be reached, and inspiratory gas trapping (“breath stacking”) occurs. Residual volume, FRC, and total lung volume are increased,398 and these effects may be directly visible. With critical IOB, pneumothorax can ensue. Barotrauma is associated with high inspiratory and plateau pressures during the respiratory cycle.399 Whereas an inspiratory pressure greater than 50 cm H2O is considered dangerous, it is not as indicative as plateau pressure.400–402 Longer expiratory time and a lower VT and rate are advised.346 This results in hypercarbia but is far less dangerous than barotrauma resulting from attempts to “normalize” CO2 tension.402 Dynamic hyperinflation, termed pulmonary tamponade or a “tourniquet to the right heart,” can compromise right ventricular function.100,346,403 Cardiac arrhythmia may complicate the situation. It results from a combination of hypoxia, hypercarbia, acidosis, and sympathetic drive, either from the patient or due to drugs.404 These immediate concerns should be communicated to the team.
c Request Help
Severe IOB is complex, risky, uncertain, and dynamic.405 Skilled help and support can make a real difference to outcome.
d Rescue
No single therapy can be reliably predicted to effectively treat IOB, especially when it is critical. A major distinction between IOB and severe asthma in awake, self-ventilating patients is the difference in bioavailability of inhaled drugs. Patients with IOB invariably have an airway device (face mask, SLA, or ETT) connected to a breathing system. The great majority of any aerosolized drug is deposited in this device, with only 3% to 9% of drug reaching the lung. This is about 10 times less efficient than administration in self-ventilating patients.99,100 A combination of inhaled and IV drugs may confer additive or synergistic effect, and a number of drug interventions are available for use in an escalating sequence.
Use of a Volatile Agent
A volatile agent may be used to increase the agent in use or to change to an alternative.100 Diethyl ether,406 halothane,24,407,408 enflurane,409 and isoflurane410 have been used. Use of sevoflurane, either for induction or in conjunction with other agents,411–413 has also been reported. Failures have been described (i.e., with isoflurane).414 Desflurane is not regarded as a bronchodilator.384
Inhaled Bronchodilators
Inhaled short-acting bronchodilators are given, either as a nebulized solution (using an in-line nebulizer) or from a pMDI via an airway adaptor. Because most of the drug will deposit in the breathing system99,309 (rain out), about 10 canister activations are initially used for an adult.100
Intravenous Bronchodilators
The use of intravenous bronchodilators has received the most support in the management of severe asthma (in nonintubated patients).415 Intravenous therapy is reported to be superior to the inhalational route.416 Sellers and Massahel report giving 5 µg/kg IV albuterol to children, 250 µg to adults; the dose can be repeated.143 Browne and Wilkins advised use of a single bolus of albuterol (salbutamol), 15 µg/kg over 10 minutes, followed by an infusion of 1 to 5 µg/kg/min.144
For refractory cases, parenteral epinephrine may be used. Cydulka and colleagues described the effective use of subcutaneous epinephrine in the emergency department.417 For adults, they advise 0.3 mg SQ epinephrine every 20 minutes. Intramuscular doses for adults range from 0.5 to 1.0 mg. Intravenous epinephrine may also be given. Suggested doses for adults are 10 to 100 µg IV, repeated as needed. A suggested infusion scheme is 0.35 to 0.7 mg/kg IV epinephrine given over 5 minutes, followed by an infusion of 0.5 to 2 µg/kg/min.
These treatments produce profound cardiovascular stimulation with tachycardia and hypertension, alleviated by concurrent use of magnesium.418 Lactic acidosis is a recognized complication of the treatment of severe asthma and IOB with high dose BAs.127,128 Hypokalemia and hypomagnesemia complicate high-dose BA therapy.47
Intravenous Glucocorticoids
Intravenous GC may provide delayed effect a number of hours later.100,309,419
Inhaled Glucocorticoids
Recognizing that GC can have therapeutic effect by nongenomic mechanisms, such as decreased airway hyperemia and mucosal edema,168,169 nebulized IGCs may be useful.346 One study showed equal efficacy of 3 mg nebulized fluticasone and 100 mg hydrocortisone in nonintubated patients with acute severe asthma.420
Ketamine
Most experience with ketamine comes from anecdotal management of severe acute asthma or status asthmaticus.414,421–426 Doses between 0.5 and 2 mg/kg IV are described, followed by an infusion of 0.5 to 2 mg/kg/hr. In a placebo-controlled, double-blind trial of 14 mechanically ventilated patients with bronchospasm, 7 patients received 1 mg/kg IV ketamine with improvement in oxygenation; CO2 clearance was unchanged in the treated group but increased in the control group.424 One study on use of 3 mg/kg/hr has been published.426
One investigation did not support superiority of ketamine in the treatment of severe acute asthma in awake patients. This was a randomized, double-blind study of 53 consecutive patients. The protocol was an IV bolus of 0.2 mg/kg ketamine, followed by an infusion of 0.5 mg/kg/hr. Dysphoric reactions in the first 9 recruits prompted the reduction of the bolus dose by half.427
Magnesium
Intravenous magnesium therapy used in the treatment of severe acute asthma was found to be beneficial and safe,428 based on the results of a multicenter, randomized, controlled trial in the emergency department giving 20 to 40 mg/kg IV over 10 minutes.429 Tachycardia provoked by sympathetic stimulants is reduced, and NMB is prolonged.418,430 Inhaled magnesium (sulfate) has been used therapeutically in the treatment of severe asthma,431 but it can be an airway irritant. Other systematic reviews of inhaled and IV magnesium therapy have concluded that it can have therapeutic benefit.432
Helium-Oxygen Carrier Gases
Helium-oxygen (heliox) mixtures are not bronchodilators, and the potential for improvement in gas exchange and respiratory mechanics is limited to their duration of use. They improve transport of gases, vapors, and aerosols by sustaining laminar flow in breathing systems (including nebulizers) and airways,433 but a major limitation is reduced inspiratory fraction of O2.47,100,346 Heliox mixtures have been used in the setting of severe acute asthma and status asthmaticus.434–437 Use of heliox for critical IOB in ventilated patients is limited.47,346,437 Systematic reviews have not provided firm evidence to support widespread use.438,439
Methylxanthines
MXs were used traditionally, but recent evidence does not support their general use for IOB, and response in the setting of severe acute asthma is rare.47
f Recovery
The place and time for recovery should be carefully considered, depending on IOB severity and response to treatment. Postoperative deterioration (biphasic effect) has been described in up to 20% of patients, especially those with bronchospasm associated with anaphylaxis.450,451 Critical care admission is a realistic option.100
If the episode of IOB was unpredicted, testing for anaphylaxis should be considered.371,452