Chapter 46 Airway Management in Intensive Care Medicine
II. Noninvasive Airway Management
III. Endotracheal Intubation in Intensive Care
IV. Difficult Airway Management in Intensive Care
V. Complications of Translaryngeal Intubation
VI. Airway Management for Prolonged Mechanical Ventilation (Tracheostomy)
VII. Routine Airway Care in Mechanically Ventilated Patients
I Introduction
This chapter briefly describes noninvasive and “invasive” airway management with special respect on the recommendations of the American Society of Anesthesiologists Task Force on Management of the Difficult Airway (ASA algorithm) first presented in 1991.1 The basic principles of the ASA algorithm are meticulous patient evaluation before sedation or anesthesia induction, awake intubation if problems are suspected, and preparation of an alternative approach in case of failure.
II Noninvasive Airway Management
Invasive ventilation using an endotracheal tube (ETT) was once the gold standard for ventilatory support of critically ill patients. However, longer duration of invasive ventilation increases the risk of complications such as ventilator-associated pneumonia (VAP).2 Noninvasive ventilation (NIV) preserves the patient’s ability to speak and cough and has been shown to reduce complications related to intubation, especially VAP.3–5 For this reason, use of NIV has become widely accepted, especially in patients with chronic obstructive pulmonary disease (COPD) and patients with cardiac failure, since weaning from respirator and ETT may become extremely difficult. In selected patients with respiratory failure, noninvasive positive pressure ventilation helps to reduce an increased respiratory rate, augments tidal volumes and reduces work of breathing.6,7 Therefore, NIV is a promising approach to ventilatory support of ICU patients and might become even more important as a “weaning as well as a therapeutic procedure” in the near future.
A Indications and Contraindications of Noninvasive Ventilation
1 Acute Respiratory Failure
Over the past decade, noninvasive positive-pressure ventilation (NPPV) has increased in popularity in the patient with acute exacerbations of COPD. In these patients, based on evidence derived from multiple randomized trials, NIV should be considered as first-line therapy.8–12
In a systematic review, Keenan and colleagues13 assessed the effect of NPPV on rate of endotracheal intubation, length of hospital stay, and in-hospital mortality in patients with an acute exacerbation of COPD. The addition of NPPV to standard care in patients with COPD exacerbation decreased the rate of endotracheal intubation (risk reduction [RR], 28%; 95% confidence interval [CI], 15%-40%), length of hospital stay (absolute reduction, 4.57 days; CI, 2.30-6.83 days), and in-hospital mortality rate (RR, 10%; CI, 5%-15%). However, subgroup analysis showed that these beneficial effects occurred only in patients with severe exacerbations, not in those with milder exacerbations of COPD.
A similar approach was used by the Cochrane Database group, presenting a systematic review on the effectiveness of NPPV in management of acute COPD exacerbations.14 Only randomized controlled trials (RCTs) were selected by two independent reviewers. NPPV not only decreased mortality (RR 0.41; 95% CI 0.26, 0.64), but also decreased the need for intubation (RR 0.42; CI 0.31, 0.59) and treatment failures (RR 0.51; CI 0.39, 0.67). In addition, complications associated with treatment (RR 0.32; CI 0.18, 0.56) and length of hospital stay (−3.24 days; CI −4.42, −2.06) were also reduced in the NPPV group. The reviewers concluded that NPPV should be used as the first-line intervention in all patients with respiratory failure secondary to an acute exacerbation of COPD. NPPV should be considered early in the course of respiratory failure, to avoid endotracheal intubation, reduce mortality, and avoid treatment failure.
Carlucci and associates15 evaluated the changes in the practice of NIV for the treatment of COPD patients between 1992 and 1999. In this special patients’ collective, the failure rate of NPPV was constant over the years (17%). Although the severity of acute respiratory failure (ARF) episodes increased (defined by pH and APACHE II at admission), the risk of failure for a patient with pH less than 7.25 was threefold lower in the period 1997-99 compared with 1992-96. Furthermore, a significantly higher percentage of patients (pH >7.28) were treated at the normal ward and not in the ICU, which allowed a significant cost reduction.
Based on strong evidence (level 1), recent clinical studies support the use of NIV to treat ARF related to COPD exacerbations, treat cardiogenic pulmonary edema, and facilitate weaning and extubation in patients with COPD and immunosuppressed patients.8,16 The future will show whether this trend away from “invasive” toward noninvasive ventilatory support in routine patient care will be sustained.
2 Weaning Strategy
Weaning from the ventilator is difficult in up to 20% of all patients. Already the consequent use of standardized weaning strategies (regardless of their content) has increased the success rates after extubation. In this context, Udwadia and coauthors17 described the use of NIV as a weaning strategy first in 1992. In 2003, Burns and coworkers18 performed the first meta-analysis of NIV as a weaning strategy, including five clinical trials and 171 patients. NIV showed significant benefit for duration of in-hospital-stay, total duration of ventilation, reduced mortality, and lower VAP rate compared with conventional weaning using endotracheal intubation. In a 2010 systematic review that included 12 randomized and quasi-randomized studies, Burns and others19 compared NIV and invasive weaning strategy in 530 COPD patients.19 This meta-analysis showed that NIV weaning in COPD patients is associated with decreased mortality, decreased incidence of VAP, reduced length of stay in ICU and hospital, and decreased total duration of ventilation and duration of endotracheal intubation.
Current studies on NIV weaning, predominantly focused on COPD patients, propose a potential reduction in mortality and VAP.20 In fact, further clinical research is necessary to clarify potential benefits of NIV as weaning strategy versus conventional weaning via ETTs or cannulas.
3 Difficult Airway Management
Critically ill patients are characterized by limited physiologic reserves, possibly resulting in catastrophic complications during airway management, including cardiac arrest. After a systematic review of airway management in critically ill patients in 2007, Walz and colleagues21 proposed adaptations to the ASA Difficult Airway Algorithm. In this algorithm, NIV serves as a valuable adjunct to conventional invasive airway management.
B Different Types of Noninvasive Devices
Various types of face masks and helmets are widely used for administering NIV (Fig. 46-1).
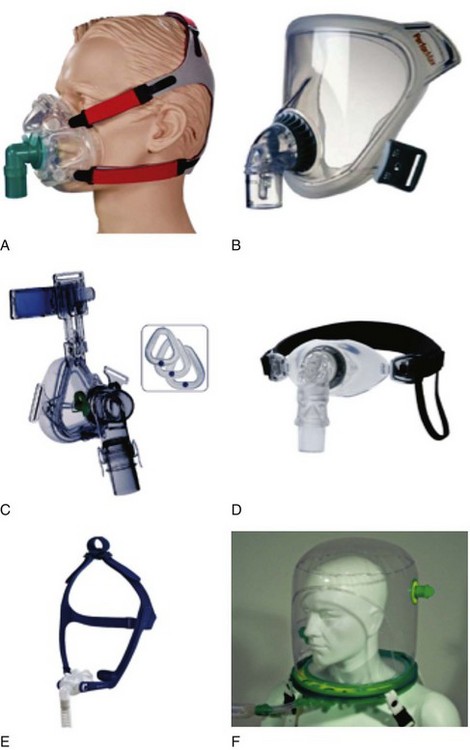
Figure 46-1 A, Full face mask; B, total face mask; C, nasal mask; D, mouthpiece; E, nasal pillows; F, helmet.
(From Nava S, Hill N: Noninvasive ventilation in acute respiratory failure. Lancet 374:250–259, 2009.)
1 Noninvasive Positive-Pressure Ventilation via Face Mask
Noninvasive PPV can be administered by face masks covering the mouth and nose (and eyes) or by nasal masks covering only the nose. Either technique includes a mask that is pressed against the patient’s face using an elastic band (e.g., Classen band). The patient may now be supported by either continuous positive airway pressure (CPAP), pressure support ventilation (PSV), or volume-cycled or pressure-cycled systems, such as bi-level positive airway pressure (BiPAP). However, dyspneic ARF patients tend to breathe through their mouth, causing air leaking and reducing the efficacy of nasal NPPV. Face masks are preferable in these patients, and application seems to be easy. However, the pressure of the face mask against the face, especially the root of the nose, causes significant discomfort and skin lesions, and several patients refused prolonged application.22,23 Further disadvantages are potential gastric inflation followed by vomiting (risk of aspiration), claustrophobia, and difficult speaking.16
2 Noninvasive Positive-Pressure Ventilation via Helmet
The helmet consists of a cylindrical transparent part that is drawn over the patient’s head (e.g., CaStar, Starmed, Italy). While the helmet is closed, the lower part contains an elastic ring to ensure a tight seal at the patient’s neck (Fig. 46-2). Two openings with sideways fittings to ventilatory tubing allow supportive ventilation such as continuous flow or pressure support. The helmet is a noninvasive means of ventilation; the patient has a free view through the transparent helmet and can even wear glasses, with no fogging from the circulating air. A new model of the Castar helmet includes an additional round opening with a diameter of about 10 cm for nursing the patient’s face. This helmet was used as first-line intervention to treat patients with hypoxemic ARF, compared with NPPV via standard face mask.24 Thirty-three consecutive patients without COPD and with hypoxemic ARF (defined as severe dyspnea at rest, respiratory rate >30 breaths/min, PaO2/FIO2 <200, and active contraction of the accessory muscles of respiration) were enrolled. The 33 patients and the 66 controls had similar characteristics at baseline. Eight patients (24%) in the helmet group and 21 patients (32%) in the facial mask group (P = .3) failed NPPV and were intubated. No patients failed NPPV because of intolerance of the technique in the helmet group, versus eight patients (38%) in the mask group (P = .047). Complications related to the technique (skin necrosis, gastric distention, eye irritation) were fewer in the helmet group compared with the mask group (no patients vs. 14 patients (21%), P = .002). The helmet allowed continuous application of NPPV for a longer period (P = .05). NPPV by helmet successfully treated hypoxemic ARF, with better tolerance and fewer complications than face mask NPPV.
In another prospective clinical investigation in a general ICU, the feasibility and safety of fiberoptic bronchoscopy (FOB) with bronchoalveolar lavage (BAL) was tested during NPPV delivered by helmet in patients with ARF and suspected pneumonia.25 Four adult patients with ARF underwent NPPV via the helmet and required fiberoptic BAL for suspected pneumonia. NPPV was delivered through the helmet in the pressure support ventilation mode. The specific seal connector placed in the plastic ring of the helmet allowed the passage of the bronchoscope, maintaining assisted ventilation. Arterial blood gas levels, pH, oxygen saturation, respiratory rate, heart rate, and mean arterial blood pressure (BP) were monitored during the study. Helmet NPPV avoided gas exchange deterioration during FOB and BAL, with good tolerance. During the procedure, heart rate increased by 5% and mean arterial BP by 7% over baseline; these levels returned to prebronchoscopy values immediately after the withdrawal of the bronchoscope. Endotracheal intubation was never required during the 24 hours after the procedure. BAL yielded diagnostic information in three of four patients. NPPV through the helmet allows a safe diagnostic FOB with BAL in patients with hypoxemic ARF, avoiding gas exchange deterioration, and endotracheal intubation.
Several special aspects of the helmet, such as the effects of direct exposure of external and middle ear to positive airway pressure, remain to be determined. Cavaliere and others26 recommended earplugs in select NPPV patients treated with the helmet, especially during long-term support at high airway pressures. NPPV has become an integral part of daily ICU care for many patients with acute and chronic respiratory failure. However, the potential effectiveness of NPPV varies among different patient populations. The greatest benefit is seen in patients with pure hypercapnic respiratory failure, as seen in COPD patients. The more severe hypoxemia becomes, the less beneficial the effects observed. Clearly, further studies are necessary to identify patients with hypoxemic ARF who might benefit from NPPV.27
The Rüsch 4Vent (Kernen, Germany) allows unrestricted field of view and minimum level of noise. Tubing and accessories are connected to the rigid ring of the 4Vent. Diffusers prevent the stream of gas from blowing directly into the patient’s face. Filters reduce the noise inside the helmet. Similar to the Castar helmet, the patient is able to speak and take liquid food and drinks. The supple material allows the helmet also to be worn lying down (Fig. 46-3).
Advantages of NPPV include few air leaks, minimal cooperation required, and absence of nasal or facial skin damage. Disadvantages include rebreathing, gastric inflation followed by vomiting, dry eyes, noise, and asynchrony with pressure support ventilation, as well as shoulder discomfort induced by the straps.16
III Endotracheal Intubation in Intensive Care
A Prelaryngoscopy Airway Assessment
Any factor in the patient’s history suggesting a difficult airway must be known before anesthesia induction, especially all diseases resulting in pharyngeal or laryngeal deformities (congenital abnormalities, surgical or traumatic deformities, laryngeal trauma, edema or tumors, goiter). As a first and rapid orientation, the simple “rule of threes” can be applied: if three fingers can be placed between the teeth, between the mandible and the hyoid bone, and between the thyroid cartilage and the sternal notch in neutral position, direct laryngoscopy should be straightforward.28 Furthermore, more or less complex tests and scoring systems have been suggested to predict a difficult airway before anesthesia induction. The most frequently used tests are the Mallampati classification, thyromental distance, mobility of the cervical spine, and combined scoring systems (e.g., Wilson test) or multivariate risk indices29–31 (Table 46-1).
Mallampati classification is the most common diagnostic test and is based on the evaluation of the relationship of tongue size relative to pharynx size, as follows31:
Class I: uvula, faucial pillars, and soft palate visible
The operator should be aware that the risk of difficult airway is increased in class II and especially class III patients. In the original publication, Mallampati and colleagues31 reported excellent statistical results on the performance of the classification system. However, later investigations could not replicate these findings and report a high incidence of false-positive results.32,33
Thyromental distance (according to Patil) is defined as distance between thyroid cartilage (Adam’s apple) and the mandible with the patient’s head and neck maximally extended. The authors measured thyromental distance before anesthesia induction in 75 patients and reported a minimum distance of 6.5 cm being necessary for a successful laryngoscopic approach. At a thyromental distance less than 6.0 cm, direct laryngoscopy is impossible; between 6.0 and 6.5 cm, the operator should be prepared for a difficult airway. Sensitivity of this test varies according to definition of the “danger zone” (i.e., thyromental distance <7.0 or <6.5 cm) and is reported in the range of 30% to 90%. In most studies, specificity ranges between 80% and 97%.32,34 Thyromental distance is a useful diagnostic test with a relatively low intra- and inter-observer variation. However, the lacking stratification for patient height and weight makes data interpretation difficult.
Direct laryngoscopy without extension of the atlanto-occipital joint is impossible. Decreased mobility of the upper cervical spine (e.g., in patients with rheumatoid arthritis) is a major factor responsible for failed attempts of endotracheal intubation.35 Nichol and Zuck30 clearly demonstrated that a reduced atlanto-occipital distance is a major factor for a limited extension of head and neck. Assessment of cervical spine mobility is performed with the patient looking straight ahead and maximum mouth opening. The patient is now urged to flex and extend the head and neck. The angle between the extreme positions should be at least 90 degrees; otherwise, a difficult laryngoscopy must be assumed, with high specificity in the 90% range.
The range and freedom of mandibular movement and the architecture of the teeth have pivotal roles in facilitating laryngoscopic intubation, so Khan and colleagues36 proposed the upper lip bite test. Patients were grouped according to the following findings: lower incisors can bite the upper lip above the vermilion line (class I); lower incisors can bite the upper lip below the vermilion line (class II); and lower incisors cannot bite the upper lip (class III). In 300 elective patients, the authors evaluated the new test with the Mallampati classification. Negative predictive value for both test groups was similar (98%), but the positive predictive value of the lip bite test was better (29% vs. 13%). To improve positive and negative predictive values of those screening tests, several authors proposed the combination of various risk scores. In 1988, Wilson and associates29 published a screening test system, including patient weight, mobility of the cervical spine, and anatomic-physiologic parameters of the stomatognathic system (Table 46-2). According to the authors, difficult airways must be suspected with 2 or more cumulative points. This scoring system requires more extensive evaluation but results in improved statistical performance. In patients with a cumulative result of 2 points, sensitivity of 75% was observed.29 Oates and coauthors32 reported specificity of 92%.
Risk Factor | Criterion | Points |
---|---|---|
Body weight | <90 kg | 0 |
90-110 kg | 1 | |
>110 kg | 2 | |
Head and neck movement | >90 degrees 90 degrees (±10) <90 degrees |
0 1 2 |
Jaw movement | MMO >5 cm or LJ in front of UJ | 0 |
MMO <5 cm and LJ = UJ | 1 | |
MMO <5 cm and LJ behind UJ | 2 | |
Receding mandible | Normal Moderate Severe |
01 2 |
Buck teeth | Normal | 0 |
Moderate | 1 | |
Severe | 2 |
MMO, Maximum mouth opening; LJ, lower jaw; UJ, upper jaw.
From Wilson ME et al: Predicting difficult intubation. Br J Anaesth 61:211–216, 1988.
El-Ganzouri and associates37 proposed a multivariate risk index, similar to the Wilson test. The authors recommended the following approach:
• Airway score (AS) of 0: proceed with routine management, no difficulties are expected.
• AS of 1 to 2 points: proceed with routine management, check fiberoptic availability, and prepare a special plan B.
• AS of 3 to 4 points: have the fiberoptic scope on standby at bedside, and call for help; prepare for asleep or awake fiberoptic intubation.
• AS greater than 5 points: perform awake intubation (most practitioners prefer awake fiberoptic intubation).
Statistical results demonstrate a relatively weak performance in clinical routine. Even combinations of tests or scoring systems combining several risk factors are prone to misjudgment for the individual patient.34 The upper airway is a complex structure and consists of many anatomically relevant variables. Also, statistical performance of these tests is still unsatisfying; the operator might be warned of trouble. Closely evaluating the patient before anesthesia induction might prevent catastrophic “cannot intubate, cannot ventilate” situations.
B Oral vs. Nasal Endotracheal Intubation
Oral intubation and especially nasal intubation are characterized by special advantages and complications, with orotracheal intubation being currently favored by most anesthesiologists. Box 46-1 lists the contraindications for nasal intubation in critically ill patients.
The most common complication of nasotracheal intubation is nasal bleeding, occurring in about 45% of patients.38 The incidence of bleeding can be reduced with adequate preparation (vasoconstrictors). In most cases, bleeding is self-limited, and no further intervention is needed. Other complications are necrosis of the tip or wing of the nose (up to 4% of patients) and complications induced by the impaired drainage of paranasal sinus secretions.39 Nasotracheal intubation impairs the drainage of the maxillary sinus, resulting in congestion of secretions, followed within a few days by bacterial overgrowth and sinusitis. This typical complication of intensive care occurs within 8 days in 25% to 100% of transnasally intubated patients.40,41 The incidence is closely related to the duration of endotracheal intubation, from 37% after 3 days to 100% after 1 week, with the majority resolving within 1 week after extubation.42 Therefore, in all ICU patients presenting with fever of unknown origin, a high index of suspicion for sinusitis must be maintained. Radiologic or ultrasound studies, including computed tomography (CT) scans, may be necessary to demonstrate the presence of fluid or inflammation. Bacterial sinus infection may ultimately lead to bacteremia and systemic inflammatory response. These infections tend to be polymicrobial, but often display a predominance of gram-negative bacilli (particularly Pseudomonas aeruginosa), Staphylococcus aureus, or fungi. Treatment includes removal of all nasal tubes and institution of appropriate antibiotic therapy, along with decongestant therapy. In some cases, surgical drainage may be necessary. Serious, but rare complications after nasotracheal intubation are accidental turbinectomy, intracranial placement, and retropharyngeal dissection.43–45
Oral intubation, on the other hand, interferes greatly with oral and pharyngeal hygiene, and even small lesions of oral soft tissues may result in extensive bacterial or viral soft tissue infections (e.g., herpetic lesions).46 Most authors agree that all complications typically seen with nasal intubation may also occur during orotracheal intubation, although at a significantly lower incidence. Therefore, oral endotracheal intubation is the preferred route of tube passage, and nasal intubation is reserved for special indications, mainly short-term ventilatory support in oral surgery. For patients requiring intubation for more than 7 days, the nasotracheal route should always be avoided.47
C Choosing the Correct ETT Size
According to literature, there is a clear association between endotracheal tube diameter and the incidence of laryngeal complications. Injury is located mainly in the posterior part of the glottis, where pressures up to 200 or 400 mm Hg are exerted by poorly deformable ETTs.48 This pressure is reduced by the use of softer ETTs with a smaller diameter. In routine anesthesia patients, use of a smaller ETT reduces the incidence of postoperative sore throat, presumably because of the decreased pressure at the ETT-mucosal interface. Stout and associates49 studied 101 patients randomized to larger (9 mm for male, 8.5 for female) or smaller (7 mm for male, 6.5 mm for female) endotracheal tubes, and the incidence of postoperative sore throat was 48% (large ETT) versus 22% (small ETT). No ventilatory difficulties were observed in either group.
The limiting factor for minimizing ETT diameter is the increased flow resistance and work of breathing. The pressure gradient required to generate gas flow can be calculated according to the Hagen-Poiseuille relationship, in which the rapidity of gas flow is directly proportional to the square of the ETT diameter (D) and the pressure (P) and indirectly to the ETT length and gas viscosity (valid only in laminar flow conditions). In other words, the pressure gradient through the airways proportionally rises with flow, viscosity, and ETT length but increases exponentially when ETT radius decreases. Viscosity of the gas administered has clinical consequences; with lower viscosity, a lower pressure gradient necessarily results in a lower airflow resistance. For example, gas mixtures with a high percentage of helium are often used to overcome upper airway obstruction (e.g., from tracheal compression or stenosis) and to treat the most severe status asthmaticus.50,51
In all patients, endotracheal intubation artificially increases airway resistance, because ETT inner diameter (ID) is smaller than tracheal diameter. Usually, ETT length exceeds length of the natural airways. Resistance is further increased by the curved design of the tubes necessary to resemble the patient’s airway. Resistance measured using curved tubes is about 3% higher compared with straight tubes.52 Therefore, the pressure gradient to generate gas flow is minimized by using an ETT with a larger diameter, short length, and straight design. Flow resistance can be translated in work of breathing, which is inversely proportional to ETT diameter (Fig. 46-4). The non-elastic work of breathing is increased twofold by using 7.0 mm–ID ETT and onefold by using an 8.0 mm–ID ETT versus a 9.0 mm–ID ETT.53 With respect to gas flow, it is warranted to use as large an ETT as is practical for patients presenting with respiratory dysfunction; a short tracheal cannula with ID of 9.0 to 10 mm is best.
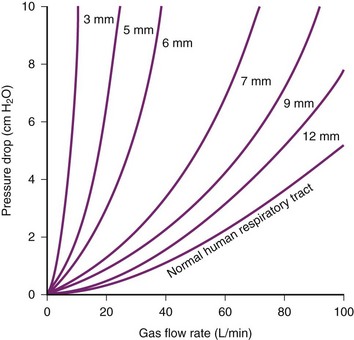
Figure 46-4 Driving pressure required to produce a flow rate of air versus internal diameter (ID) of endotracheal tubes.
(From Nunn JF: Applied respiratory physiology, ed 3, Boston, 1987, Butterworths.)
Clinical Implications
An interesting aspect of ETT selection is airway management for professional singers. Powner54 sent a written survey to all physician members of the Voice Foundation on airway management in professional singers. A strong consensus (76%) favored a smaller ETT for singers (6- to 7-mm ID for males and 6.0-mm ID for females) via the oral (46%) versus nasal (36%) route. Intubation/extubation by the most expert/experienced personnel was emphasized to minimize laryngeal trauma. Preferences for an early tracheostomy (6 days) versus their usual time (10 days) were approximately equal (44% vs. 50%, respectively).
The tracheal cuff has also been implicated as a cause of tracheal damage following long-term ventilatory support. Red-rubber ETTs equipped with low–residual volume, high-pressure cuffs exert high pressures to the tracheal mucosa and are thought to be damaging.55 Using an experimental study design in rabbits, Nordin and coauthors56 investigated tracheal mucosal perfusion and cuff-to-tracheal wall pressures exerted by low–residual volume, high-pressure cuffs and compared the results to those obtained with high-volume, low-pressure cuffs. Blood flow to the tracheal mucosa adjacent to the high-pressure cuff ceased beyond 30 mm Hg. Using high-volume cuffs, mucosal blood flow did not cease up to intracuff pressures of 80 to 120 mm Hg. However, cautious recommendations were made, and cuff pressure should be maintained below 30 cm H2O.55
Prolonged airway support using ETT or tracheal cannula might result in severe damage to the tracheal mucosa and increased incidence of late complications. Tracheal stenosis might be induced by direct trauma or by the pressure exerted by the ETT cuff. The presence of an ETT sutured to canine tracheal mucosa induced erythematous laryngeal mucosa in less than 1 day, proceeding to mucosal ulceration and loss of airway architecture in less than 1 week.57 Damage was generally severe after 1 week, but no further tendency to aggravation after 1 week was observed.
The tracheal vascular supply to the tracheal submucosa is oriented in a circumferential direction anteriorly and longitudinally in the posterior part of the trachea. Seegobin and van Hessalt58 investigated tracheal mucosal blood flow in 40 routine surgery patients using an endoscopic photographic technique while varying cuff inflation pressure. They suggested that tracheal blood flow is almost normal at 25 cm H2O. Increasing cuff pressure results in a pale appearance of the tracheal mucosa at 40, blanched at 50, and flow is completely absent at 60 cm H2O. Thus, it was recommended that cuff inflation pressure should not exceed 30 cm H2O (22 mm Hg).
Joh and colleagues59 observed similar results, measuring tracheal mucosal blood flow in an experimental model using the hydrogen clearance method. No significant depression in mucosal blood flow was observed with the cuff inflated to a maximum of 20 mm Hg. Increasing cuff pressures to 30 or even 45 mm Hg resulted in significant reduction in mucosal blood flow, and the authors concluded that cuff pressures should be kept at or below 20 mm Hg. Incorrect cuff pressure settings may result in tracheomalacia and subglottic stenosis (too high pressures) or late-onset nosocomial pneumonia (insufficient pressures). Several authors suggested that microaspirations might thereby be facilitated and recommended the use of automatic cuff-pressure regulators.60 In a randomized study in 130 patients, Pothmann and associates61 reported a significant reduction in late-onset pneumonias by a microprocessor-controlled automatic cuff-pressure regulator. However, the few studies covering small numbers of patients means that the devices described cannot currently be recommended for routine patient care.60,62
D Drugs Used for Sedation and Analgesia
The presumption of a “full stomach” in emergency intubations dictates the use of a rapid-sequence induction (RSI) technique. Cricoid pressure and laryngoscopy at the earliest possible moment place increased demands on the physician preparing to intubate the patient.63 The ICU patient presents with even more risk factors than the routine OR patient. With facial distortion, swelling, secretions, and mandibular or cervical spine injury, ICU patients present the most challenging airway management problems.
Stimulation of the airway with a laryngoscope and ETT presents an extremely noxious stimulus.64 This stimulation causes “pressor response” and results in hypertension and tachycardia. In critically ill patients with limited reserves for adequate tissue oxygenation, this pressor response may induce myocardial and cerebrovascular injury.65 This physiologic stress after airway management may unmask relative hypovolemia or vasodilatation and may result in post-intubation hypotension.66 Endotracheal intubation also can provoke bronchospasm and coughing, possibly aggravating underlying conditions such as asthma, intraocular hypertension, and intracranial hypertension. Patients at risk for adverse events from airway manipulation benefit from pre-induction drugs.67
We prefer the combination of low-dose midazolam (0.1 µg/kg) and an opioid such as fentanyl (2 µg/kg). Fentanyl is the most frequently used opioid because of its rapid onset and short duration of action. Fentanyl and its derivatives, sufentanil and alfentanil, can cause rigidity of chest wall, but this rigidity seems to be associated with higher doses and rapid opioid injection. Fentanyl can be antagonized rapidly using naloxone,68 whereas midazolam can be antagonized using flumazenil.
Remifentanil is a novel µ-receptor agonist that has been used in surgical and obstetric analgesia for more than a decade. Its pharmacokinetics suggests that remifentanil may be an ideal opioid. Notably, its unique features include rapid onset of action (~1 minute), rapid degradation by tissue and plasma esterases, and half-life of approximately 3 minutes.69,70 Infusion of 0.05 to 0.1 µg/kg/min produces blood concentrations of 1 to 3 ng/mL. Rapid onset and elimination of remifentanil facilitate its effective and safe use during airway management in the ICU. Several studies focused on remifentanil as a sole sedative and analgesic agent in the ICU setting. Puchner and coworkers71 prospectively studied remifentanil (0.1-0.5 µg/kg/min) alone versus fentanyl plus midazolam for fiberoptic intubation. Fiberoptic nasal intubation was possible in all patients, with a better-suppressed hemodynamic response and tolerance of ETT advance in the remifentanil group. Recall was significantly more common in the remifentanil group. A combination of remifentanil and midazolam or propofol is a valuable approach.72
Lallo and coworkers73 recently studied 60 patients requiring fiberoptic nasotracheal intubation, randomized to target-controlled infusions of propofol or remifentanil. Both agents can be rapidly titrated to achieve good intubating conditions and patient comfort. In addition, remifentanil preserves patient cooperation, making it safer when spontaneous ventilation is paramount. Yeganeh and associates74 enrolled 22 patients who had cervical trauma and semi-elective maxillofacial surgery and administered remifentanil with target-controlled or manually controlled infusion techniques. They recommended remifentanil infusion to provide good conscious sedation, but target-controlled remifentanil infusion seems to provide better conditions compared with manually controlled remifentanil infusion and is easier to use.
Alternative drugs suitable for ICU airway management and even awake fiberoptic intubation are (S+)-ketamine and propofol.75 Propofol is a rapid-acting, lipid-soluble induction drug that induces hypnosis in a single arm-brain circulation time. In patients with cardiac comorbidities and limited physiologic reserve, the use of propofol can be associated with significant hypotension. Causes of hypotension are reduced systemic vascular resistance and possibly depressed inotropy.76 In an analysis of 4096 patients undergoing general anesthesia, Reich and associates77 reported that the use of propofol was a statistically significant predictor of hypotension. Furthermore, in 2406 patients with retrievable outcome data, prolonged in-hospital stay and death were more common in those who experienced hypotension.77 Therefore, use of propofol may have special risks in high-risk patients with known cardiac dysfunction.78
Ketamine is a rapid-acting, dissociative anesthetic agent (similar to phencyclidine) with potent amnestic, analgesic, and sympathomimetic effects.67 Ketamine can cause realistic hallucinations and extreme emotional distress. This may be prevented with the obligatory combination of a benzodiazepine. The sympathomimetic effects of ketamine may produce cardiac ischemia by increasing cardiac output. Consequently, use of ketamine should be avoided in patients with cardiac dysfunction. Leykin and others79 found that the administration of ketamine resulted in excellent intubation conditions in patients undergoing elective surgery compared with sodium pentothal. In a prospective, randomized clinical trial, Ledowski and Wulf80 reported that the combination of ketamine with rocuronium and etomidate resulted in superior intubation conditions. This combination may be useful in treating hemodynamically unstable patients in the ICU setting, when succinylcholine is contraindicated.21 The use of ketamine in patients with increased intracranial pressure is only recommended in mechanically ventilated patients.81 Ketamine is associated with realistic and truthful nightmares. In this context, the combination with midazolam seems to be an obligatory recommendation. Currently, an S(+) enantiomer of ketamine is available. This enantiomer seems to be free of these clinically relevant side effects although use of these S(+) enantiomers is still recommended in combination with midazolam.
Etomidate provides good intubation conditions with only moderate hemodynamic depression in patients undergoing RSI.82 Etomidate inhibits adrenosteroid production through the inhibition of mitochondrial hydroxylase, after continuous or even single administration.83–85 Cuthbergson and colleagues86 recruited 500 patients to a multicenter, randomized, double-blind, placebo-controlled trial. The use of bolus etomidate was associated with an increased incidence of inadequate response to corticotrophin and is likely associated with an increase in mortality. Therefore, the authors recommend extreme caution in the use of etomidate in critically ill patients with septic shock.86 Roberts and Redman87 and Bloomfield and Noble88,89 also questioned any use of etomidate in critically ill patients.
Dexmedetomidine is increasingly used as a sedative for monitored anesthesia. Advantages include its analgesic properties, “cooperative sedation,” and lack of respiratory depression. Dexmedetomidine is also increasingly used in RSI and awake intubation, with promising studies showing a beneficial effect.90,91 However, long-term observational studies are still lacking, and the current scientific level is too weak to recommend routine use of dexmedetomidine.
Succinylcholine is a very-rapid-acting, polarizing muscle relaxant enabling excellent intubation conditions in less than 1 minute.92 Unfortunately, it has serious side effects, including the possibility of triggering malignant hyperthermia. The use of succinylcholine is contraindicated in patients with acute major burns, upper or lower neuronal lesions, prolonged immobility, massive crush injuries, and various myopathies.21 Benumof and associates93 clearly demonstrated that even the short duration of action of succinylcholine is too long, and critical hemoglobin desaturation will occur before return to an unparalyzed state, even after a 1-mg/kg dose.93
Rocuronium is the most widely used non-depolarizing neuromuscular blocking agent. The recommended dosage of “normal” intubation is 0.6 mg/kg. For RSI, higher dosages up to 1.2 mg/kg are necessary and provide excellent intubation conditions within 1 minute.94 A meta-analysis by the Cochrane Collaborative Group concluded that succinylcholine created superior intubation conditions compared with rocuronium.95 The only absolute contraindication against the use of rocuronium is allergy to aminosteroid neuromuscular drugs. A major advantage of rocuronium is the possibility of rapid and complete reversal of even deep neuromuscular block by the administration of sugammadex.96 Based on a systemic review by Chambers and others,97 the use of sugammadex in combination with high-dose rocuronium is efficacious.
E Awake Endotracheal Intubation
Once the patient is properly prepared, any of the intubation techniques listed in Box 46-2 can be chosen according to the operator’s preference.98–102
Box 46-2 Nonsurgical Techniques for Awake Intubation
A valuable alternative approach is the insertion of the intubating laryngeal mask airway (ILMA) under local anesthesia and light sedation. Intubation is then performed blindly or using a fiberscope inserted through the LMA. Langeron and coworkers103 prospectively compared ILMA intubation with fiberoptic intubation in patients with difficult airway and reported similar success rates (>90%) and durations for both techniques. Another interesting approach is the combination of Fastrach and lighted stylet. Dimitriou and associates104 studied 44 of 11,621 patients in whom three attempts of direct laryngoscopy failed. In all these patients, an ILMA was inserted and sufficient ventilation was possible in all patients after a maximum of two attempts. Thereafter, a well-lubricated silicon ETT loaded with a flexible lightwand was introduced. Lightwand intubation was successful at the first attempt in 38 of 44 patients, at the second attempt in three patients, and at the third to fifth attempt in two. Intubation failed in only one patient. Finally, if all those alternatives fail, the establishment of a surgical airway should be considered.