Chapter 2 Airway Imaging
Principles and Practical Guide
II Imaging Modalities
A Conventional Radiography (Plain Film)
Wilhelm Conrad Roentgen, a German physicist, discovered x-rays on November 8, 1895, while studying the behavior of cathode rays (electrons) in high-energy cathode ray tubes. By serendipity, he noticed that a mysterious ray that escaped the cathode tube penetrated objects differently, and he named this the x-ray. For his work, he was awarded the first Nobel Prize for Physics in 1901.1
X-rays are a type of electromagnetic radiation; as the name implies, they transport energy through space as a combination of electric and magnetic fields. Other types of electromagnetic radiation include radio waves, radiant heat, and visible light. In diagnostic radiology, the predominant energy source used for imaging is ionizing radiation (i.e., alpha, beta, gamma, and x-rays). The science of electromagnetic waves and x-ray generation is very complex and exceeds the scope of this text. In principle, x-rays are produced by energy conversion as a fast stream of electrons is suddenly decelerated in an x-ray tube.2 The localized x-ray beam that is produced passes through the part of the body being studied. The final image is dependent on the degree of attenuation of the beam by matter.
Attenuation is the reduction in the intensity of the beam as it traverses matter of different constituents. It is caused by absorption or deflection of photons from the beam. The transmitted beam determines the final image, which is represented in shades of gray.3–5 The lightest or brightest area on the film or image represents the greatest attenuation of the beam by tissue and the least amount of beam transmitted to film. For example, bone is a high-density material that attenuates much of the x-ray beam; images of bone on x-ray films are very bright or white. A plain film image is a one-dimensional collapsed or compressed view of the body part being imaged. This information can also be presented in a digital format without the use of traditional x-ray films.
B Computed Tomography
After the discovery of x-rays, it became apparent that images of the internal structures of the human body could yield important diagnostic information. However, the usefulness of x-ray studies is limited because they project a three-dimensional (3-D) object onto a two-dimensional display. With x-rays, the details of internal objects are masked by the shadows of overlying and underlying structures. The goal of diagnostic imaging is to bring forth the organ or area of interest in detail while eliminating unwanted information. Various film-based traditional tomographic techniques were developed, culminating in the creation of computerized axial tomography or computed tomography (CT).6 The first clinically viable CT scanner was developed by Hounsfield and commercially marketed by EMI Limited (Middlesex, England) for brain imaging in the early 1970s.7 Since then, several generations of CT scanners have been developed.
As with conventional plain film radiography, CT technology requires x-rays as the energy source. Whereas conventional radiography employs a single beam of x-rays from a single direction and yields a static image, CT images are obtained with the use of multiple collimated x-ray beams from multiple angles, and the transmitted radiation is counted by a row or rows of detectors. The patient is enclosed in a gantry, and a fan-shaped x-ray source rotates around the patient. The radiation counted by the detectors is analyzed with the use of mathematical equations to localize and characterize tissues by density and attenuation measurements. A single cross-sectional image is produced with one rotation of the gantry.6 The gantry must then “unwind” to prepare for the next slice while the table carrying the patient moves forward or backward by a distance that is predetermined by slice thickness. An intrinsic limitation of this technique is the time required for moving the mechanical parts.
The introduction of slip-ring technology in the 1990s and the development of faster computers, high-energy x-ray tubes, and multidetectors enabled continuous activation of the x-ray source without having to unwind the gantry and also allowed continuous movement of the tabletop. This process, known as helical CT, is used in the latest generation of CT scanners. Because the information acquired using helical CT is volumetric, in contrast to the single slice obtained with conventional CT, the entire thorax or abdomen can be scanned in a single breath-hold. Volumetric information makes it possible to identify small lesions more accurately and allows better 3-D reformation. Because of the higher speed of data acquisition, misregistration and image degradation caused by patient motion are no longer significant concerns. This is especially important when scanning uncooperative patients and trauma victims. The absorbed radiation dose used in multidetector helical CT (as compared with conventional single-detector row CT) is dependent on the scanning protocol and varies with the desired high-speed or high-quality study.8
C Magnetic Resonance Imaging
MRI has become one of the most widely used imaging modalities in diagnostic radiology. In contrast to conventional radiography and CT, MRI uses no ionizing radiation. Instead, imaging is based on the resonance of the atomic nuclei of certain elements such as sodium, phosphorus, and hydrogen in response to radio waves of the same frequency produced in a static magnetic field environment. Current clinical MRI units use protons from the nuclei of hydrogen atoms to generate images because hydrogen is the most abundant element in the body. Every water molecule contains two hydrogen atoms, and larger molecules, such as lipids and proteins, contain many hydrogen atoms. Powerful electromagnets are used to create a magnetic field, which influences the alignment of protons in hydrogen atoms in the body. When radio waves are applied, protons are knocked out of natural alignment, and when the radio wave is stopped, the protons return to their original state of equilibrium, realigning to the steady magnetic field and emitting energy, which is translated into weak radio signals. The time it takes for the protons to realign, referred to as a relaxation time, is dependent on the tissue composition and cellular environment.9 The different relaxation times and signal strengths of the protons are processed by a computer, generating diagnostic images. With MRI, the chemical and physical properties of matter are examined at the molecular level. The relaxation times for each tissue type, designated T1 and T2, are expressed as constants at a given magnetic field strength. Imaging that optimizes T1 or T2 characteristics is referred to as T1-weighted or T2-weighted imaging, respectively. Tissue response to pathologic processes usually includes an increase in bound water (edema), which lengthens the T2 relaxation time and appears as a bright focus on T2-weighted images.9
III Basics of Plain Film Interpretation
A Cervical Spine Radiography
1 General Technique, Anatomy, and Basic Interpretation
The most common indications for obtaining cervical spine radiographs in today’s medical practice are for the evaluation of trauma, spinal stability, and cervical spondylosis and in the search for radiopaque foreign bodies. Different views of the cervical spine are tailored to each clinical need. The most common views are the lateral, anteroposterior (AP), open-mouth odontoid, oblique, and pillar views (Fig. 2-1). In acute cervical spine injury, cross-table lateral, AP, and open-mouth odontoid views are recommended. A lateral view reveals the majority of injuries (Fig. 2-2); however, patients who are rendered quadriplegic by severe ligamentous injuries may demonstrate a normal lateral cervical spine radiograph. When the AP and then the open-mouth odontoid views are added to the cross-table lateral view of the cervical spine, the sensitivity of detecting significant injury is increased from 74% to 82% and then to 93%.10 In today’s practice, cross-sectional imaging (i.e., CT of the spine) has become a mainstay in the evaluation of the cervical spine, especially in the setting of acute trauma. MRI is particularly useful in evaluating the spinal cord.
In brief, a normal lateral cervical radiograph should demonstrate seven intact vertebrae and normal alignment of the anterior and posterior aspects of the vertebral bodies. This is especially important for trauma victims, because 7% to 14% of fractures are known to occur at the C7 or C7-T1 level.11 The posterior vertebral body line is more reliable and must be intact. The anterior vertebral line is often encumbered by the presence of anterior osteophytes. Normal facet joints overlap in an orderly fashion, similar to shingles on a rooftop. The spinolaminar line, which is the dense cortical line representing the junction of the posterior laminae and the posterior spinous process, is uninterrupted. Relative uniformity of the interlaminar (interspinous) distances should be observed. The posterior spinal line (i.e., posterior cervical line), an imaginary line extending from the spinolaminar line of the atlas to C3 (Fig. 2-3), should demonstrate a continuous curve in parallel to the posterior vertebral body line; the distance between the two correlates with the spinal canal diameter.12
The anatomy and integrity of the craniocervical junction are crucial to the anesthesiologist. To achieve successful and safe endotracheal intubation, the anterior atlantodental interval (AADI), the vertical and anterior-posterior position of the dens, and the degree of extension of the head on the neck must be considered. The anterior arch of C1 bears a constant relationship to the dens; this is the AADI or predental space. It is defined as the space between the posterior surface of the anterior arch of C1 and the anterior surface of the dens. In flexion, because of the physiologic laxity of the cervicocranial ligaments, the anterior tubercle of the atlas assumes a more normal-appearing relationship to the dens, and the AADI increases in width, greater rostrally than caudally. In children and with flexion in adults, the AADI is normally about 5 mm. In adults, it is generally accepted that the AADI is 3 mm or less (Fig. 2-4).12
The bony structures of the atlantoaxial joint provide mobility (e.g., rotational movement) rather than stability. Therefore, the ligaments play a significant role in stability. The most important ligaments in the upper cervical spine are the transverse ligament, the alar ligaments, and the tectorial membrane. If the transverse ligament is disrupted and the alar and apical ligaments remain intact, up to 5 mm of movement at the atlantoaxial joint can be seen.13 If all the ligaments have been disrupted, the AADI can measure 10 mm or larger. In atlantoaxial subluxation, the dens is invariably displaced posteriorly, which causes narrowing of the spinal canal and potential impingement of the spinal cord. The space available for the spinal cord is defined as the diameter of the spinal canal as measured in the AP plane, at the C1 level, that is not occupied by the odontoid process. In the normal spine, this space is approximately 20 mm.13
2 Pertinent Findings and Pathology
a Pseudosubluxation and Pseudodislocation
Pseudosubluxation and pseudodislocation are terms applied to the physiologic anterior displacement of C2 on C3 that is frequently seen in infants and young children (Fig. 2-5). Physiologic anterior displacement of C2 on C3 and of C3 on C4 occurs in 24% and 14%, respectively, of children up to 8 years of age.14
b Congenital and Developmental Anomalies
Occipital Fusion of C1
Important to rigid laryngoscopy and endotracheal intubation is the distance between the occiput and the posterior tubercle of C1, known as the atlanto-occipital distance (Fig. 2-6), which is quite variable from individual to individual. Head extension is limited by the abutment of the occiput to the posterior tubercle of C1. It has been proposed that a shorter atlanto-occipital distance decreases the effectiveness of head extension and contributes to difficult intubation.13,15 Occipitalization of C1 with the occiput (atlanto-occipital fusion) not only limits head extension but also adds stress to the atlantoaxial joint. Although the majority of head extension occurs at the atlanto-occipital joint, some extension can also occur at C1-C2.15 Nichol and Zuck observed that in patients with limited or no extension possible at the atlanto-occipital joint, general extension of the head actually brings the larynx “anterior,” thus limiting the visibility of the larynx on laryngoscopy.15
Nonfusion of Anterior and Posterior Arches Of C1
Ossification of the atlas begins with the lateral masses during intrauterine life. At birth, neither the anterior nor the posterior arches are fused. Fusion of the anterior arch is complete between 7 and 10 years of age. During the second year, the center of the posterior tubercle appears, and by the end of the fourth year, the posterior arch becomes complete.12 Nonfusion of the anterior or the posterior arch, or both, exists as a normal variant in adults and should not be mistaken for fracture (Fig. 2-7).
Pseudofractures of C2 and Dens
The dens ossifies from two vertically oriented centers that fuse by the seventh fetal month. Cranially, a central cleft separates the tips of these ossification centers (Fig. 2-8), and it can mimic a fracture if ossification is incomplete. The ossiculum terminale, the ossification center for the tip of the dens, may be visible on plain films, conventional tomograms, or CT scans and unites with the body by age 11 or 12 years. Failure of the ossiculum terminale to develop or failure to unite with the dens may result in a bulbous cleft dens tip. A nonunited terminal dental ossification center, called the os terminale, may be mistaken for a fracture of the odontoid tip.
Hypoplasia of C2
The position and anatomy of the dens with respect to the anterior arch of C1 and the foramen magnum are worthy of attention. Congenital anomalies of the odontoid process, such as hypoplasia, can result in a loss of the buttressing action of the dens during extension and subsequent compression of neural elements. Examples of conditions that are associated with odontoid hypoplasia are the Morquio, Klippel-Feil, and Down syndromes; neurofibromatosis; dwarfism; spondyloepiphyseal dysplasia; osteogenesis imperfecta; and congenital scoliosis.13,16 Patients with these conditions are predisposed to atlantoaxial subluxation and craniocervical instability, and hyperextension of the head for intubation should be avoided. In addition, congenital fusion of C2 and C3 (Fig. 2-9), whether occurring as an isolated anomaly or as part of Klippel-Feil syndrome, places added stress at the C1-C2 junction.
c Acquired Pathology
Cervical Spondylosis
Cervical spine radiographs are obtained for the evaluation of cervical spondylosis (Fig. 2-10). The hypertrophic bone changes associated with this condition are well depicted on radiographic studies. Large anterior osteophytes that project forward may cause dysphagia and difficult intubation. The bone canal and neural foramina are assessed for stenosis; if stenosis is present, precautions can be taken when hyperextending the neck and positioning the patient to avoid exacerbation of baseline neurologic symptomatology. Calcification and ossification are well depicted on radiographic studies.
Ossification of the anterior longitudinal ligament and diffuse idiopathic skeletal hyperostosis have been reported as causes of difficult intubation.17 This can be readily appreciated on plain films. Another condition that may signal difficult intubation is calcification of the stylohyoid ligament (Fig. 2-11).18
Inflammatory Arthropathies
Inflammatory arthropathies involving the atlantoaxial joint with subluxation are classically seen in patients with rheumatoid arthritis or ankylosing spondylitis. However, the underlying causes of atlantoaxial subluxation are quite different in these two entities. Ankylosing spondylitis is characterized by progressive fibrosis and ossification of ligaments and joint capsules. In rheumatoid arthritis, bone erosion, synovial overgrowth, and destruction of the ligaments occur. Patients with rheumatoid arthritis are not only susceptible to AP subluxation at the C1-C2 junction but also at risk for vertical subluxation of the dens. Whether this condition is referred to as “cranial settling,” superior migration of the odontoid process, or basilar invagination, the end result is the same.12 The odontoid process protrudes above the foramen magnum, narrowing the available space for the spinal cord and potentially leading to cord compression with the slightest head extension (Fig. 2-12).13
In response to the effective foreshortening of the spine that occurs secondary to the superior migration of the odontoid process from inflammatory or degenerative disease, there is acquired rotational malalignment between the spine and larynx.19 The larynx and the trachea, because they are semirigid structures and as a result of the tethering effect of the arch of the aorta as it passes posteriorly over the left main bronchus, are predictably displaced caudally, deviated laterally to the left, rotated to the right, and anteriorly angulated. The effective neck length can be affected by superior migration of the dens, severe spondylosis with loss of disc space, or iatrogenic causes secondary to surgery. The soft tissues of the pharynx become more redundant owing to the relative shortening of the neck, which further obscures the view of the larynx. On laryngoscopy, the vocal cords are rotated clockwise. A rotated airway is suspected when the frontal view of the cervical spine demonstrates a deviated tracheal air column.
d Anthropologic Measurements
Historically, bony landmarks other than the spine that can be appreciated on a lateral cervical spine radiograph have been used in the anesthesia arena to preoperatively predict difficult laryngoscopy and endotracheal intubation on the basis of anatomic factors. Mandibular size, the ratios of the various measurements, and their relationship to the hyoid bone have been proposed as predictors of difficult laryngoscopy (Fig. 2-13).20 These measurements are meant to reflect the oral capacity, the degree of mouth opening, and the level of larynx.21,22 It is apparent that the causes of difficult laryngoscopy and endotracheal intubation are multifactorial. Combined with the clinical examination, anatomic measurements and findings assessed by x-ray studies can help alert the anesthesiologist to a potentially difficult airway. In this way, difficult laryngoscopy and endotracheal intubation can be anticipated and not unexpected.
B Soft Tissue Neck Radiography
1 General Technique, Anatomy, and Basic Interpretation
The lateral cervical spine study with bone and soft tissue technique allows an incidental view of the aerodigestive tract and a gross assessment of the overall patency of the airway. Useful ossified cartilage or bony landmarks of the pharynx and larynx that can be appreciated on the lateral neck radiograph are the hard palate, hyoid bone, thyroid, and cricoid cartilages (Fig. 2-14). The hard palate is a bony landmark used to separate the nasopharynx from the oropharynx. The larynx can be thought of as being suspended from the hyoid bone. Muscles acting on the hyoid bone elevate the larynx and provide the primary protection from aspiration. The largest cartilage in the neck is the thyroid cartilage, which along with the cricoid cartilage acts as a protective shield for the inner larynx. The cricoid cartilage is the only complete cartilaginous ring in the respiratory system. It is located at the level where the larynx ends and the trachea begins.
Normal air-filled structures seen on lateral plain films are the nasopharynx, oropharynx, and hypopharynx. Air in the pharynx outlines the soft palate, uvula, base of the tongue, and nasopharyngeal airway (Fig. 2-15). Any sizable soft tissue pathology results in deviation or effacement of the airway. The tongue constitutes the bulk of the soft tissues at the level of the oropharynx. In children, and sometimes in adults, prominent lymphatic tissues such as adenoids and palatine tonsils may encroach on the nasopharyngeal and oral airways. Lingual tonsils are located at the base of the tongue above the valleculae, which are air-filled pouches between the tongue base and the free margin of the epiglottis.
The epiglottis is an elastic fibrocartilage shaped like a flattened teardrop or leaf that tapers inferiorly and attaches to the thyroid cartilage. The epiglottis tends to be more angular in infants than in adults. During the first several years of life, the larynx changes its position in the neck.23,24 The free edge of the epiglottis in neonates is found at or near the C1 level, and the cricoid cartilage, representing the most caudal portion of the larynx, is at the C4-C5 level. By adolescence, the epiglottis is found at the C2-C3 level and the cricoid is at the C6 level. The adult epiglottis is usually seen at the C3 level, with the cricoid at C6-C7. However, the position of these structures in the normal population varies by at least one vertebral body level.
Sometimes visualized by a cervical spine radiographic study with soft tissue neck technique or on the CT scout view or the MR sagittal view of the neck is a transversely oriented, air-containing lucent stripe, located just below the base of the aryepiglottic folds, which indicates the position of the air-filled laryngeal ventricle (Fig. 2-16). This marks the position of the true vocal cords, which are just below this lucent stripe. Lateral to the aryepiglottic fold is the pyriform sinus of the pharynx. This anterior mucosal recess lies between the posterior third of the thyroid cartilage and the aryepiglottic fold. The extreme lower aspect of the pyriform sinus is situated between the mucosa-covered arytenoids and the mucosa-covered thyroid cartilage, at the level of the true vocal cords. The air column caudally represents the cervical trachea. On the AP view, the false and true vocal cords above and below the laryngeal ventricles may be identified, as well as the subglottic region and the trachea.
In the lower neck (C3 to C7), the prevertebral soft tissue shadow differs from that in the cervicocranium because of the presence of the beginning of the esophagus and the prevertebral fascial space, which are recognized on the lateral radiograph as a fat stripe. By standard anatomic description, the esophagus begins at the level of C4; however, in vivo, the esophageal ostium may normally be found as high as C3 or as low as C6 and varies with the phase of swallowing and the flexion and extension of the cervical spine.25 The prevertebral soft tissue thickness, the distance between the posterior pharyngeal air column and the anterior portion of the third or fourth vertebra, should not exceed one half to three quarters of the diameter of the vertebral body. In the opinion of Harris and Mirvis, only the measurement at C3 is valid, and it should not exceed 4 mm (Fig. 2-17).12
2 Classic Plain Film Diagnosis
a Acute Epiglottitis
The findings on plain film are swelling or enlargement of the epiglottis. On the conventional lateral radiograph of the neck, thickening of the free edge of the epiglottis can be appreciated and is referred to as the “thumb sign” (Fig. 2-18). The width of the adult epiglottis should be less than one third of the AP width of the C4 body. Cross-sectional imaging is superfluous. However, the degree of airway compromise can theoretically be quantified by 3-D reformation.
b Laryngotracheobronchitis or Croup
In laryngotracheobronchitis or croup, the subglottic larynx is involved. This condition affects younger children and has a less fulminant course than acute epiglottitis. The swelling of the soft tissues in the subglottic neck can be appreciated on an AP view of the neck (Fig. 2-19). There is usually a long segment narrowing of the glottis and subglottic airway with loss of the normal angle between the vocal cords and the subglottic airway. This has been referred to as the “steeple sign.” The hypopharynx is usually dilated because of the airway obstruction distally.
c Foreign Body
Plain films are usually obtained in the initial assessment of suspected foreign body ingestion. In children, up to 50% of witnessed foreign body ingestions are asymptomatic.26 Most foreign bodies are radiopaque, but wood and plastic usually are not visible on plain films. The radiopacity of ingested fishbone varies with the type of fish.27 In the neck, ingested foreign bodies most often lodge at the level of the pyriform sinus (Fig. 2-20).
C Chest Radiography
1 General Technique
Before the advent of CT, chest radiography was routinely ordered to assess pulmonary and cardiovascular status, and it is still a cost-efficient examination that yields a great deal of general information. The most common views of the chest are the posteroanterior (PA), anteroposterior (AP), and lateral projections (Fig. 2-21). The PA chest view is obtained with the patient’s anterior chest closest to the film cassette and the x-ray beam directed from a posterior to an anterior direction. Alternatively, the AP chest view is done with the patient’s back closest to the film cassette and the x-ray beam directed in the anterior to posterior direction. The part of the chest closest to the film cassette is the least magnified; therefore, the cardiac silhouette is larger on the AP projection. The lateral projection is most often performed with the patient’s left chest closest to the film cassette for better delineation of the structures in the left hemithorax, which is more obscured by the heart on a PA projection.
It is useful to train one’s eyes to analyze the chest radiograph systematically to cover the details of the chest wall, including the ribs, lungs (field and expansion), and mediastinal structures such as the heart and the outline of the tracheal-bronchial tree. On an adequate inspiratory film, the hemidiaphragms are below the anterior end of the sixth rib, or at least below the 10th posterior rib, and the lung expansion should be symmetrical. The right hemidiaphragm is usually half an interspace higher than the left, which is depressed by the heart (see Fig. 2-21A). Without doubt, the art of chest radiograph interpretation has diminished since the advent of CT, which demonstrates chest pathology with unparalleled clarity. However, chest radiography can still provide a composite survey of the chest at one quick glance. One can easily compare the lung volumes, identify the position of the mediastinum, determine the presence or absence of major airspace disease, and make a gross assessment of the cardiac status.
2 Interpretation of Pertinent Findings
a Level of Diaphragm
A high hemidiaphragm implies reduced lung volume, which can result from phrenic nerve paralysis, thoracic conditions causing chest pain that leads to splinting, or extrapulmonic processes such as an enlarged spleen or liver, pancreatitis, or subphrenic abscess. The presumed level of the hemidiaphragm is seen as an edge or transition between the aerated lungs and the opacity of the organs in the abdomen. If the thin leaves of the hemidiaphragm are outlined by air, a pneumoperitoneum should be considered (Fig. 2-22).
b Lung Aeration
A well-expanded lung should appear radiographically lucent but be traversed by “lung markings,” thin threads of interstitium consisting of septa and arterial, venous, and lymphatic vessels. In most normal individuals, the lungs appear more lucent at the top owing to the distribution of the pulmonary vasculature, the effect of gravity, and overlying soft tissues such as breast tissues. In patients with congestive heart failure or pulmonary venous hypertension, this pattern is reversed, with “cephalization” and engorgement of the pulmonary veins in the upper lung zones (Fig. 2-23; also see Fig. 2-21D). In general, any process such as fluid, pus, or cells that replaces the airspaces of the lungs causes the x-ray beam to be more attenuated, allowing less of the beam to be transmitted through the patient to the film. This causes the affected areas to appear less dark or more opaque (white) on the film. A whole host of diseases could be responsible, depending on the clinical picture, including pleural effusion, pulmonary edema, pneumonia, lung mass, lung collapse or atelectasis, lung infarct or contusion, and metastatic disease (Fig. 2-24). The key from an anesthesiologist’s point of view is not to make the correct pathologic diagnosis but to note the abnormality, which may affect ventilation, and adjust the anesthetic practice accordingly.
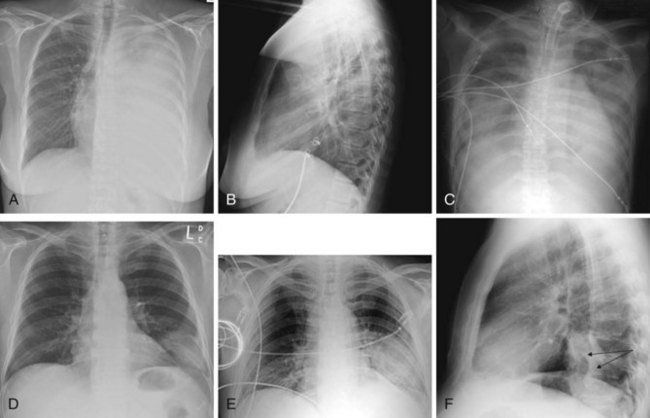
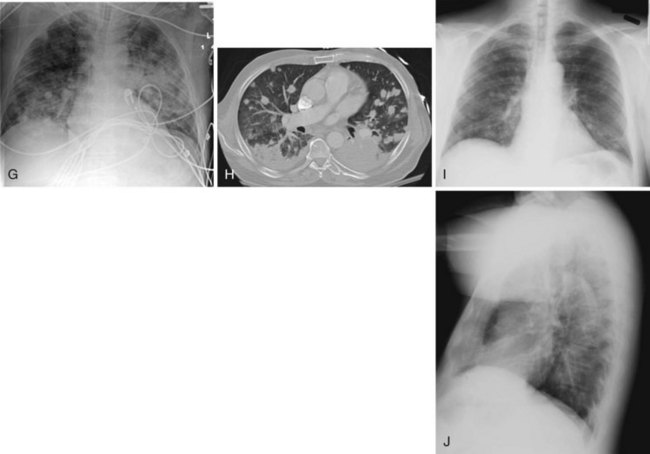
Figure 2-24 A and B, Left pleural effusion. Posteroanterior (PA) view of the chest (A) shows almost complete “white-out” of the left hemithorax and minimal residual aerated left upper lung zone. There is a mass effect with deviation of the trachea to the right. On the lateral view (B), the pleural effusion is less apparent. The tipoff is the lack of the expected lucency overlying the spine at the base (compare Fig. 2-21, C and D). C, Pulmonary edema. Anteroposterior (AP) view of the chest demonstrates bilateral hazy lung fields with air bronchogram. A tracheostomy tube is present. D through F, Left lower lung mass. Notice that although the inspiratory effort is the same on both the PA (D) and the AP (E) view (i.e., hemidiaphragm below ninth posterior rib), the cardiac silhouette and the left lower lobe mass appear larger on the AP view by virtue of the film geometry and magnification factor. The lateral view (F) helps to localize the disease process to the lateral segment of the left lower lobe. A mass is noted with postobstructive atelectasis (arrows). G and H, Aspergillosis. AP chest radiograph (G) shows nodular densities in both lungs. The differential diagnosis includes inflammatory and neoplastic processes. Notice that the tip of the endotracheal tube is in a good position, above the carina, and there is a central line on the right. Axial computed tomogram of the chest (H) better demonstrates the nodular pattern of lung involvement. I and J, Melanoma metastases to the lungs. The PA (I) and lateral (J)