Chapter 72 Acquired Disorders Affecting the White Matter
Acute CNS Demyelination
A first demyelinating attack in childhood (referred to as an acquired demyelinating syndrome or ADS) may present either with neurological signs and symptoms attributable to a single CNS lesion (monofocal ADS), or with signs attributable to multiple CNS sites (polyfocal ADS). If polyfocal symptoms are accompanied by encephalopathy, the diagnosis of acute disseminated encephalomyelitis (ADEM) is conferred [Krupp et al., 2007].
The frequency of ADS presentations differs by patient age, and not all ADS presentations are equivalent in terms of subsequent MS risk. Data from a Canadian study of 219 children with ADS revealed the most common ADS presentation to be optic neuritis (23 percent), followed by ADEM (22 percent) and transverse myelitis (22 percent) [Banwell et al., 2009]. Children with ADEM were more likely to be under 10 years of age, whereas children with monofocal ADS (optic neuritis, transverse myelitis, and others) were more likely to be over 10 years of age.
Optic Neuritis
Optic neuritis (ON) should be considered in the differential diagnosis of any child presenting with acute or subacute visual loss (Figure 72-1). Typical clinical features of demyelinating ON include reduced visual acuity, a central visual field deficit (not always testable in young children), pain with eye movements, and red color desaturation [Lucchinetti et al., 1997]. Optic disc edema is sometimes seen, but this finding may be absent in retrobulbar ON. Concurrent or rapidly sequential transverse myelitis, magnetic resonance imaging (MRI) features of brainstem or diencephalic involvement in a pattern atypical for MS, and serum antibodies against aquaporin 4 support a diagnosis of NMO (discussed later in this chapter) [Wingerchuk et al., 2006].
In a study of 36 children aged 2–17 years, ON was found to be more commonly unilateral (58 percent) than bilateral (42 percent) [Wilejto et al., 2006]. Neurological abnormalities apart from the optic nerve were common, affecting 13 children (36 percent). Neuroimaging studies of the optic nerve were abnormal in 55 percent, and 88 percent had abnormal visual-evoked potentials.
Optical coherence tomography (OCT) is a noninvasive technique that utilizes near-infrared light to measure retinal nerve fiber thickness, yielding a quantitative measure of axonal loss. This technique is currently being explored in both adults and children with ADS and MS as a way to provide an estimate of visual prognosis following ON [Frohman et al., 2006; Yeh et al., 2009a].
Following an episode of ON in childhood, approximately 80–85 percent of children achieve a full visual recovery [Hwang et al., 2002; Wilejto et al., 2006]. Children with ON have about a 36 percent risk of subsequent MS diagnosis overall, and MS may be more common in children presenting with bilateral optic nerve involvement [Lucchinetti et al., 1997; Wilejto et al., 2006]. MRI evidence of one or more T2-weighted lesions separate from the optic nerve is strongly predictive of MS risk, with up to 68 percent of such patients being diagnosed with MS within 2 years of their initial ON [Wilejto et al., 2006].
Transverse Myelitis
Demyelination of the spinal cord, accompanied by clinical signs of partial or complete cord impairment, is referred to as transverse myelitis (TM). The typical clinical features of TM include subacute onset of bilateral lower-extremity weakness, a spinal sensory level, and impaired bowel or bladder control. (Lesions above the sacral spine usually cause urinary retention and constipation.) L’Hermitte’s symptom (pain with forward neck flexion) frequently indicates a cervical spinal cord lesion. Paresis is often initially flaccid with associated hyporeflexia, and later hyperreflexia develops below the level of the lesion. These features are helpful in distinguishing TM from other diseases affecting the spinal cord (Figure 72-2).
A review of 47 patients with TM showed that, at the time of maximal deficit, 89 percent of children either were unable to walk or required assistance with ventilation (or both) [Pidcock et al., 2007]. After 3 years of follow-up, 43 percent were unable to ambulate more than 30 feet, and 21 percent required a walker or ambulatory aid. Sixty-eight percent of children had residual bladder symptoms and 50 percent required bladder catheterization. Younger age at the onset of the TM event, complete paraplegia, and a shorter time (less than 24 hours) to maximal deficit have been associated with poorer functional outcomes [Dunne et al., 1986; Miyazawa et al., 2003; Pidcock et al., 2007]. The risk of MS following acute TM is approximately 2–8 percent [Dunne et al., 1986; Defresne et al., 2003; Miyazawa et al., 2003; Mikaeloff et al., 2004a; Pidcock et al., 2007].
Polyfocal Demyelination
Neurological symptoms suggesting multiple CNS areas of involvement may occur with or without concurrent encephalopathy (Figure 72-3). When encephalopathy (either behavioral change or alteration in level of consciousness) is present, the clinical syndrome is referred to as acute disseminated encephalomyelitis (ADEM) [Krupp et al., 2007]. ADEM tends to occur in younger patients, especially those under the age of 10 [Tenembaum et al., 2002]. Fever and a recent history of infection are often present [Rust, 2000; Tenembaum et al., 2002].
Recovery from ADEM can be incomplete, with 11–43 percent of children experiencing residual motor deficits [Dale et al., 2000; Tenembaum et al., 2002]. Cognitive deficits are often present, even in children who experience a full motor recovery [Hahn et al., 2003].
ADEM is most often a monophasic illness. However, approximately 10–29 percent of children with an initial presentation of ADEM will go on to have additional demyelinating attacks [Tenembaum et al., 2002; Mikaeloff et al., 2004]. The classification of further attacks of demyelination in children initially presenting with ADEM is reviewed later in this chapter.
Investigation of a Child with Acute Demyelination
Laboratory Investigations
Cerebrospinal fluid (CSF) analysis is important for excluding diseases other than demyelination, including infection and malignancy. CSF leukocyte counts in children with MS at first presentation are elevated (>4 cells/μL) in 66 percent of children and are typically below 30 cells/μL [Pohl et al., 2004]. Higher leukocyte counts are more consistent with acute infection, vasculitis, or NMO [Benseler and Schneider, 2004; Zaffaroni, 2004]. Elevated protein in the range of 100–720 mg/L may be seen, indicating disruption of the blood–CSF barrier [Pohl et al., 2004]. CSF oligoclonal bands (OCBs) are detected at the time of ADS in approximately 90 percent of children who are subsequently diagnosed with MS [Sindern et al., 1992; Ghezzi et al., 2002; Ozakbas et al., 2003; Pohl et al., 2004], which is slightly lower than the reported detection rate of 98 percent in adult MS patients [Reiber and Peter, 2001]. Isoelectric focusing methods have the highest yield for oligoclonal band detection [Freedman et al., 2005]. In contrast, CSF OCBs are detected in fewer than 10 percent of children with either ADEM [Tenembaum et al., 2002; Atzori et al., 2009] or NMO [Banwell et al., 2008; McKeon et al., 2008]. It should be noted that CSF immunoglobulin G synthesis can occur later in the disease course. Consequently, some MS patients who have initially negative CSF studies will later have detectable OCBs.
Evoked potential testing in the visual-evoked potential, brainstem auditory-evoked potential, and somatosensory-evoked potential pathways is useful for confirming the presence of demyelination and for detecting clinically silent disease. A study of 47 children with ON showed that visual-evoked potentials were abnormal in 88 percent [Wilejto et al., 2006]. In one study, almost 50 percent of 85 childhood patients with MS demonstrated clinically silent abnormalities in at least one of the evoked potential pathways, most commonly in the visual pathways [Pohl et al., 2006a].
Magnetic Resonance Imaging
MRI is a valuable tool to demonstrate the presence of inflammatory demyelination and to exclude other diagnoses [Miller et al., 1990] (Figure 72-4). Large lesions, ill-defined lesions, and lesions present in gray as well as white matter commonly occur in younger children, especially those with ADEM [Murthy et al., 2002; Garg, 2003; Mikaeloff et al., 2004b]. Several groups have highlighted the challenge in identifying MRI features sensitive enough to distinguish ADEM from MS at first attack [Schwarz et al., 2001; Mikaeloff et al., 2004b]. Recently proposed criteria suggest that the following features may be useful in making this distinction:
Serial MRI studies have been useful in showing dissemination in space within the CNS and time, and MRI characteristics have been incorporated into the diagnostic criteria for adult-onset and childhood-onset MS [McDonald et al., 2001; Barkhof et al., 2003; Polman et al., 2005; Krupp et al., 2007]. These criteria have between 52 and 76 percent sensitivity for distinguishing childhood MS from other diseases at first attack [Hahn et al., 2004; Mikaeloff et al., 2004b; Callen et al., 2009b]. Proposed revisions to these criteria for childhood MS have suggested that fewer lesions (≥5 T2 lesions, and either ≥2 periventricular lesions or ≥1 brainstem lesion) may be able to differentiate MS from other neurological disorders at first presentation with improved sensitivity and specificity [Callen et al., 2009b], but further validation is required.
Management of Acute Demyelination
Acute demyelination in children is generally treated with corticosteroids if symptoms are severe enough to interfere with daily functioning (Figure 72-5). While there are no published standardized trials for steroid therapy in children, typically 20–30 mg/kg/day of intravenous methylprednisolone (maximum 1 g) is given as a single daily intravenous dose for 3–5 days. If there is a significant improvement in symptoms, no further treatment is required. If there is an incomplete response to treatment but the symptoms are relatively mild, a tapering course of oral steroids (starting at 1 mg/kg/day and tapering over 21 days) is often considered. If children have significant residual symptoms after a course of intravenous steroids, a second 3–5-day course may be given at the same doses as outlined above. Alternatively, treatment with intravenous immunoglobulin (IVIg) at a dose of 2 g/kg over 2–5 days may be of benefit (class IV evidence) [Hahn et al., 1996; Nishikawa et al., 1999].
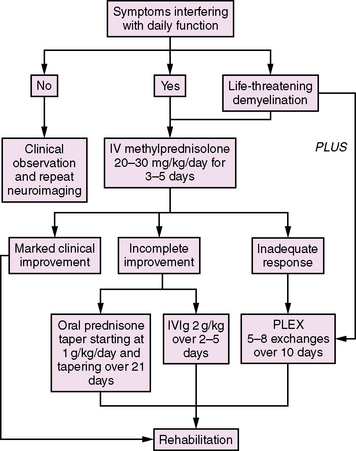
Fig. 72-5 Approach to the management of acute demyelination in childhood.
IV, intravenous; IVIG, intravenous immunoglobulin; PLEX, plasma exchange.
(Adapted from Thomas T, Banwell B. Multiple sclerosis in children. Semin Neurol 2008;28[1]:69–83.)
Rarely, acute demyelination in children can be life-threatening. Profound encephalopathy and respiratory depression may occur with brainstem and upper cervical spinal cord involvement. Plasma exchange may be useful in this situation, based on class I evidence in adult-onset MS [Weinshenker et al., 1999; Weinshenker, 2001]. A typical regimen of plasma exchange treatment would be 5–8 exchanges given over 10 days.
Acute Necrotizing Encephalopathy of Childhood
An acute necrotizing encephalopathy (ANE), with elevated serum aminotransaminases and bilateral symmetric thalamic lesions on neuroimaging, was first described in a Japanese child in 1979 (Mizuta et al., 1979), and since then there have been over 200 reports in the literature. The majority of children with ANE originate from the Far East, especially Japan, Taiwan, and Korea [Mizuguchi, 1997; Kim et al., 2004], with fewer cases reported in North America or Europe [Mastroyianni et al., 2006]. Recently, an affected kindred was described, representing the only case of male siblings with consanguineous parents [Marco et al., 2010] described in the United States. Familial (ANE1) and recurrent cases have recently been linked to mutations in RANBP2 (RAN-binding protein 2) [Neilson et al., 2009].
ANE typically affects children under the age of 5 [Mizuguchi, 1997; Mastroyianni et al., 2006]; in one series of 62, predominantly Japanese, patients, 46 percent of children were between 6 and 18 months of age [Mizuguchi et al., 1995]. In this same series, symptom onset occurred in winter months (December through February) in 51 percent of cases. Boys and girls appear to be equally affected [Mizuguchi et al., 1995; Mastroyianni et al., 2006].
A prodromal febrile illness is reported in up to 90 percent of children [Mizuguchi et al., 1995] and concurrent influenza A, influenza B, human herpesvirus (HHV)-6, Mycoplasma pneumoniae, herpes simplex, or measles infection has been documented in some patients [Mizuguchi, 1997; Mastroyianni et al., 2006]. Symptoms of an acute encephalopathy typically evolve 12–72 hours later and include decreased level of consciousness (28 percent), vomiting (20 percent), and seizures (40 percent) [Mizuguchi, 1997]. The disease is rapidly progressive and coma may ensue in less than 24 hours. Upper motor neuron signs, including hyperreflexia and extensor plantar responses, are seen acutely in two-thirds of patients [Mizuguchi, 1997; Mastroyianni et al., 2006].
Laboratory findings and neuroimaging features are helpful in distinguishing ANE from other conditions that may present in a similar manner, including ADEM (see earlier in this chapter). Laboratory findings in ANE include elevated serum aspartate aminotransferase, alanine aminotransferase, and lactate dehydrogenase in 50–95 percent of children [Mizuguchi, 1997; Mastroyianni et al., 2006]. Elevations in serum ammonia and bilirubin are uncommon. Metabolic acidosis may be present over 80 percent of children [Mizuguchi, 1997]. CSF examination demonstrates an opening pressure greater than 15 cm H2O with elevated CSF protein (>40 mg/dL) in the absence of pleocytosis in more than two-thirds of patients [Mizuguchi, 1997; Mastroyianni et al., 2006].
Neuroimaging characteristically reveals bilateral symmetric thalamic lesions, often accompanied by brainstem tegmentum, periventricular white-matter, putaminal, and cerebellar lesions. These lesions are initially hypodense on computed tomography (CT) and hypointense on T2-weighted MRI, later evolving into concentric lesions with a central area of hemorrhage that appears hyperdense on CT or hyperintense on T1-weighted MRI images [Mizuguchi et al., 1995; Mizuguchi, 1997; Mizuguchi et al., 2002]. Contrast enhancement around the area of hemorrhage becomes apparent by 2 weeks [Wong et al., 2006], and cavitation occurs in 50 percent of lesions [Kim et al., 2004; Wong et al., 2006]. Areas of diffusion restriction within acute lesions have been described [Albayram et al., 2004].
The outcome from ANE has historically been considered poor, with death in the acute phase occurring in up to one-third of patients [Mizuguchi, 1997]; however, more recently, patients with milder disease and fewer residual neurological sequelae have been reported [Kim et al., 2004; Okumura et al., 2006]. Patients may be left with residual neurological signs, including focal weakness, extraocular movement abnormalities, extrapyramidal movement disorders, or ataxia related to the sites of brain involvement visualized on MRI [Mizuguchi, 1997; Mastroyianni et al., 2006; Wong et al., 2006]. A normal CSF protein in the acute phase and lack of brainstem lesions on neuroimaging appear to be correlated with a more favorable long-term neurological outcome [Charney et al., 1979; Mizuguchi, 1997; Yoshikawa et al., 1999; Weitkamp et al., 2004; Wong et al., 2006].