8. Acid-Base Homeostasis and Oxygenation*
Amy M. Wood and M. Douglas. Jones Jr.
Examination of arterial blood gases and interpretation of acid-base balance are essential to proper diagnosis, management, and outcome in an ill neonate. 3,17,28 The measurement of arterial blood gases allows analysis of two interrelated but separate processes: acid-base homeostasis and oxygenation. 1,16,21 This chapter describes the parameters that designate these processes, their measurements, and the effects of proposed treatment on homeostasis. 1,28 Common abbreviations and their meanings are listed in Box 8-1.
BOX 8-1
A Alveolar
a Arterial
D Difference
F Fraction
I Inhalation, inspired
P Partial pressure (tension, driving force)
pH Negative log of hydrogen ion concentration
Combined Abbreviations
Pa o2Partial pressure of arterial oxygen
F io2Fraction of inspired oxygen
P io2Partial pressure of inspired oxygen
Components of arterial blood gases include (1) actually measured values (Pa o2, Pa co2, and pH) and (2) calculations from these values (oxygen saturation, bicarbonate concentration, and base excess). Some analyzer systems also estimate hemoglobin concentration. The pH, Pa co2, base excess, and bicarbonate components are used to assess acid-base homeostasis, 16,21,31 whereas Pa o2, saturation (Sa o2), and hemoglobin1,3 are used to assess adequacy of oxygenation (Table 8-1).
Blood Gases | Values |
---|---|
pH | 7.35-7.45 |
Paco 2 | 35-45 mm Hg |
![]() |
18-26 mEq/L |
Base excess | (−5) to (+5) |
Pao 2 | 60-80 mm Hg |
O 2 saturation | 92%-94% |
PHYSIOLOGY
Acid-Base Homeostasis
To review basic chemistry, an acid is a hydrogen ion donor and a base is a hydrogen ion receptor. The pH refers to the concentration of hydrogen ions [H +] in blood and reflects the acid-base balance in blood. 31 The quantity of hydrogen ions is minute, approximately 0.0000001 mole/L. Therefore the negative log of the hydrogen ion concentration is used to define pH and create a positive, workable number (pH = 7) (Equation 1). A pH of 7 represents a neutral solution, a pH of less than 7 represents acidity, and a pH greater than 7 represents alkalinity:
(1)
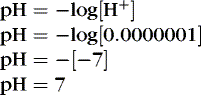
The Henderson-Hasselbalch equation describes pH as equal to a constant (pK) plus the logarithm of the ratio of the base-to-acid concentration (Equation 2). 3,17 Thus if there is an increase in the concentration of hydrogen ions (reflected in the denominator), the blood pH value decreases and acidemia results. Conversely, if there is less acid or more base, pH increases and alkalemia results. 3
(2)

The first step in determining acid-base homeostasis is measurement of pH. Normal human pH is between 7.35 and 7.45. Acidemia and acidosis are often used interchangeably, but strictly speaking, pH of less than 7.35 is acidemia and the process that caused it is acidosis; a pH of greater than 7.45 is alkalemia and the process that caused it is alkalosis. 17Arterial carbon dioxide (Pa co2) and bicarbonate [
] values represent the two main components of acid-base homeostasis: (1) respiratory contribution (Pa co2) controlled by alveolar ventilation,1,8,11and (2) nonrespiratory or metabolic contribution controlled primarily by renal excretion, retention, or production of [
]. 1,15,26Other factors that affect nonrespiratory components of acid-base balance cause a change in [
] ; thus [
] is an indicator of the nonrespiratory component. 15,19,26




RESPIRATORY CONTRIBUTION
Carbon dioxide is produced by each cell as a product of metabolism. 1,23 As carbon dioxide is produced, it dissolves in intracellular fluid and can be measured as the partial pressure (P) of the dissolved gas (CO 2). As the pressure of the dissolved gas increases inside the cell, carbon dioxide moves out of the cell into the blood. Blood transports dissolved carbon dioxide (some combined with hemoglobin as carboxyhemoglobin, most as bicarbonate) to the lung, where the partial pressure in the pulmonary capillary is greater than in the alveoli, 21 causing carbon dioxide to move into the alveoli. Ventilation is the only method of removing carbon dioxide. The amount of carbon dioxide in the blood is the net result of the body’s metabolism (production) and alveolar ventilation (clearance). Because metabolism does not change greatly and CO 2 diffuses easily across membranes, the only clinically important limitation to CO 2 removal is at the lungs. Thus Pa co2 reflects alveolar ventilation.3,8,11,17
In the red blood cell, the enzyme carbonic anhydrase promotes combination of a fraction of dissolved CO 2 with water to form carbonic acid (H 2CO 3), which then dissociates into a hydrogen ion [H +] and a bicarbonate ion [
] 1,23:

(3)

Therefore an increase in Pa co2 causes pH to fall. Hypoventilation causes an increase in carbon dioxide. This is called respiratory acidosis because the respiratory system is responsible for regulating Pa co2 as the lung regulates the amount of carbon dioxide in the body.8 A decrease in Pa co2 results in less acid in the blood and causes pH to rise. A pathophysiologic process that causes hyperventilation and reduces dissolved carbon dioxide is known as respiratory alkalosis.
NONRESPIRATORY (METABOLIC) CONTRIBUTION
Nonrespiratory (metabolic) derangements can also disturb acid-base homeostasis. Normal metabolism produces hydrogen ions. Blood pH is maintained within normal limits by renal mechanisms for excreting hydrogen ions. Increased production of [H +] may occur in conditions such as shock with poor perfusion of the gastrointestinal system or genetically determined aberrations of metabolism.27 The hydrogen ions produced must be eliminated to avoid a fall in blood pH. Derangements also occur when hydrogen ions are lost (e.g., in gastric juice) or when bicarbonate is lost (e.g., in diarrheal fluid or ileostomy drainage). 28
Authorities differ as to the best way to describe nonrespiratory derangements in acid-base status. The traditional approach relies on measurement of pH, P co2 and [
]. The alternative is description of acid-base status in terms of (1) strong ions (Na +, K +, Ca 2+, Mg 2+, Cl −), strong because they remain dissociated at body pH, and (2) weak acids (hemoglobin, albumin, inorganic phosphate), weak because they are partially dissociated at body pH. Blood pH in this conceptualization is a function of the difference between strong cations and strong anions, the strong ion difference (SID). As an example, the alkalosis associated with loss of gastric fluid would be described exclusively in terms of loss of [Cl −] with loss of [H +] making no independent contribution to the resulting alkalemia. Advocates maintain that measurement of SID leads to greater understanding of the causes of nonrespiratory and mixed acid-base derangements. 7,12,14,23 Others favor staying with the traditional bicarbonate-center model. 6,18 The present discussion focuses on the traditional bicarbonate-centered approach. Readers are referred to recent reviews for comparisons of the two methods. 14,18

A fall in blood [
] might indicate that bicarbonate, a base and therefore a hydrogen ion acceptor, has been used up by the addition of [H +]. As shown inEquation 3, a change in [
] might also reflect a change in Pco2. This difficulty is overcome in blood gas analyzers by correcting the P co2 (graphically) to 40 mm Hg, yielding a “standard bicarbonate” concentration.23The standard bicarbonate concentration and the buffering properties of hemoglobin are combined in the concept of base excess (BE). A positive value suggests a deficit of fixed (i.e., not volatile as with H 2CO 3) acid or an excess of base; a negative value indicates an excess of fixed acid or a deficit of base.23An abnormality of the standard bicarbonate concentration or base excess indicates a process of nonrespiratory (metabolic) alkalosis15or nonrespiratory (metabolic) acidosis.26


In the Henderson-Hasselbalch equation (Equation 2), the pH is equal to a constant, pK, plus the logarithm of the base/acid ratio. 1,17,23 If we substitute [
] for the base and dissolved CO 2 for the acid, 8,23 multiplying CO 2 by its solubility coefficient (0.03 mEq/L/mm Hg), the equation becomes the following:

(4)

The value of pK is 6.1; normal [
] is 24 mEq/L, and normal Pa co2 is 40 mm Hg.

Substituting, we obtain the following:
(5)
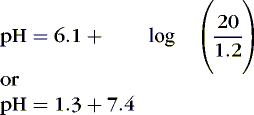
1. Hypoventilation of sufficient degree that Pa co2 is doubled from 40 to 80 (respiratory acidosis) results in a ratio of 24:2.4, or 10. The logarithm of 10 is 1, and the pH would be 6.1 + 1, or 7.1.
2. If a metabolic acidosis reduced [
] from 24 mEq/L to 12 mEq/L, the ratio would be 12:1.2 or 10:1, and the pH would be 7.1.

MIXED CONTRIBUTIONS
Thus far, these derangements (Figure 8-1) have been discussed as if they happened in isolation, but combined respiratory and nonrespiratory problems often occur, depending on pathologic processes in the body. Besides the four single acid-base derangements, there are combined acid-base derangements: (1) respiratory acidosis and metabolic acidosis, (2) respiratory acidosis and metabolic alkalosis, (3) respiratory alkalosis and metabolic acidosis, and (4) respiratory alkalosis and metabolic alkalosis. The combined acidoses or combined alkaloses have a cumulative effect on the pH, whereas an acidosis and alkalosis combination tends to negate the effects of each on the pH value. 16,17,21,31
COMPENSATION
Acid-base homeostasis maintains pH near the normal range. Thus if either the respiratory or nonrespiratory acid-base system is “deranged,” the other system will become “unbalanced” in the opposite direction to counterbalance the primary process. The body attempts to maintain equilibrium by balancing a pathologic process with a physiologic process or predictable buffering response. 3,17,28 For example, any respiratory process that leads to retention of carbon dioxide (respiratory acidosis) stimulates a nonrespiratory system, in this case the renal system, to return pH toward normal. This occurs by renal retention of bicarbonate and corresponding excretion of hydrogen ions. Given sufficient time, this may increase blood bicarbonate by as much as 3 to 4 mEq/L for each 10 mm Hg increase in carbon dioxide. Thus a neonate with a chronically increased Pa co2 and a compensatory rise in bicarbonate may attain a near-normal pH. 17
Metabolic compensations to respiratory processes can go to remarkable extremes, but respiratory compensations to metabolic processes are limited. Hyperventilation cannot lower the Pa co2 much below 10 mm Hg in compensation for a metabolic acidosis. Similarly, hypoventilation is limited in compensation for a metabolic alkalosis by the onset of hypoxemia. 28 Hypoxemia stimulates respiratory drive, overriding compensatory hypoventilation, limiting correction of alkalemia. 17
CORRECTION
Correction of an acid-base disturbance occurs when the health care provider detects the pathophysiologic process and directs therapy at the primary pathologic process, rather than counterbalancing it with a second pathologic process.
For example, if a respiratory acidosis is present, the clinician assesses the patient to discover the cause of the carbon dioxide retention and directs therapy at improving the ventilatory capacity of the lung, rather than attempting to increase the retention of bicarbonate.
Oxygenation
The remaining components of the blood gas analysis are the P o2, hemoglobin, and oxygen saturation. 21 Oxygenation is related to but also distinct from ventilation. 3The two main factors contributing to oxygenation at the tissue level are oxygen delivery and oxygen consumption. Oxygen delivery is the product of the cardiac output and the oxygen carrying capacity of blood, whereas oxygen consumption is determined by the metabolic needs of the body’s tissues. Tissue hypoxia may be caused by many different factors that derange the balance between oxygen delivery and tissue needs. Inability of the lung to oxygenate the blood would decrease oxygen delivery because of arterial hypoxemia. Another cause of tissue hypoxia is interference with blood flow, as in heart failure. The Pa o2 may be normal, but because of heart (pump) failure, oxygenated blood is not delivered in sufficient quantity. Treatment should be directed toward improving delivery by the pump (see Chapter 24). A third cause of tissue hypoxia is decreased blood oxygen–carrying capacity as with anemia. In this instance, the heart and lungs work adequately, so Pa o2 is normal but hemoglobin is insufficient to provide adequate oxygen. Finally, tissue hypoxia may result from an abnormally high affinity of oxygen for hemoglobin. Because oxyhemoglobin affinity is increased, oxygen will not dissociate from hemoglobin unless the venous, and therefore tissue, P o2 falls to an unusually low level. 5
Because Pa o2 measures only the partial pressure of oxygen in arterial blood (i.e., measures the amount of dissolved oxygen gas in the blood), it reflects lung function but not tissue oxygenation. Despite this, measurement of Pa o2 together with measurement of hemoglobin and clinical assessment of tissue perfusion is usually sufficient. 3 Two situations merit special comment. First, in a preterm infant whose retinal development is incomplete, high Pa o2 can lead to retinopathy of prematurity, especially at Pa o2 greater than 100 mm Hg (see Chapter 23). Second, in cyanotic congenital heart disease, there is a right-to-left intracardiac shunt that does not allow Pa o2 to rise despite administration of supplemental oxygen because a portion of venous return goes directly to the left side of the heart, bypassing the lungs. Low Pa o2 in these patients is not related to lung disease, although lung disease may complicate the picture.
Theoretically, in a normal lung with perfectly matched ventilation and perfusion, the alveolar (P ao 2) and the arterial oxygen tension (P ao 2) should be equal. This is not achieved. A difference (gradient) exists between the P ao 2 and the Pao 2. Minor mismatching of ventilation and perfusion leads to a functional intrapulmonary shunt. This creates an alveolar-arterial oxygen gradient (D[ a-a]O 2). 3,8 However, a D( a-a)O 2 greater than 20 mm Hg indicates pulmonary disease. 3
OXYHEMOGLOBIN SATURATION
Oxyhemoglobin saturation is the percentage of hemoglobin that is combined with oxygen. Oxygen binding with hemoglobin increases as the partial pressure of oxygen increases, but not linearly. 3,31 The oxygen dissociation curve is a measure of the affinity that hemoglobin has for oxygen (Figure 8-2).
The “30-60-90 rule” is useful in remembering percent saturation and reconstructing the adult hemoglobin dissociation curve if necessary (seeFigure 8-2). At a Pa o2 of 30 mm Hg, the oxygen saturation is 60%; at a Pa o2 of 60 mm Hg, saturation is 90%; and at 90 mm Hg Pa o2, the hemoglobin is 95% saturated. At the normal venous oxygen tension of 40 mm Hg, the oxygen saturation is 75%. Factors that affect this affinity include temperature, pH, and hemoglobin structure. Hypothermia, alkalemia, hypocapnia, and fetal hemoglobin increase the affinity of hemoglobin for oxygen (shift the curve to the left), whereas fever, acidemia, and hypercapnia decrease the affinity of hemoglobin for oxygen (shift the curve to the right).
At a given tissue P o2, an increased affinity for oxygen releases less oxygen at the tissue level, whereas a decreased affinity releases more oxygen to the tissue. Alternately, the P o2 at which the oxygen-binding sites of hemoglobin are 50% saturated (the P 50) is low when the hemoglobin affinity is great and higher when the hemoglobin affinity is low. 3The affinity of fetal hemoglobin for oxygen is higher than adult hemoglobin (seeFigure 7-1). The P 50 of fetal hemoglobin is 19 mm Hg compared with a P 50 of 27 mm Hg for adult hemoglobin. Approximately 70% of hemoglobin in term infants, and more in preterm infants, consists of fetal hemoglobin.5As a result, hemoglobin in a term infant with a Pa o2 of 35 mm Hg will be 80% saturated, and a “pink” newborn infant may have a very low Pa o2.
OXYGEN CONTENT
Oxygen content is calculated from the hemoglobin saturation and hemoglobin concentration. One gram of hemoglobin binds 1.39 mL of oxygen. The oxygen content in milliliters per deciliter is the product of the saturation percentage and the hemoglobin in grams per deciliter plus the amount of dissolved oxygen. For clinical purposes, we can neglect the amount of dissolved oxygen in plasma, because it is only 0.003 mL/dL/mm Hg.
Oxygen content becomes critical in anemia, which can cause a significant derangement in tissue oxygenation unless organ blood flow and cardiac output increase to maintain the delivery of oxygen.3,31 The blood of an infant with a hemoglobin of 8 g/dL will have half the oxygen content of that of an infant with a hemoglobin of 16 g/dL at an equivalent percentage saturation. In Figure 8-2, an infant with 16 g hemoglobin that is 95% saturated (Pa o2 = 90 mm Hg) carries 21.1 mL/dL oxygen, whereas the infant with 8 g hemoglobin carries 10.6 mL/dL oxygen. Tissues require approximately 4 to 5 mL/dL oxygen for metabolism. With normal cardiac output, venous blood contains 4 to 5 mL/dL oxygen less than the arterial blood. The venous oxygen content in an infant with 16 g hemoglobin would be between 16 and 17 mL/dL, which corresponds to approximately 75% saturation, or a Pv o2 of 40 mm Hg. However, unless cardiac output increases, the venous oxygen content in an infant with 8 g hemoglobin would be 6.1 mL/dL oxygen. The saturation is 55%, which corresponds to a P o2 of less than 30.
BLOOD FLOW AND SHUNTS
The product of oxygen content and blood flow returning from the lungs determines the total amount of oxygen in arterial blood. Total pulmonary blood flow can be divided into the amount of blood in pulmonary capillaries and the amount that is shunted through or around (i.e., through an intracardiac shunt) the lungs.
A right-to-left shunt occurs when blood passes from the systemic venous to the systemic arterial circulation without receiving oxygen. This can occur because of anatomic defects in the heart (e.g., cyanotic congenital heart disease) or because of blood perfusing alveoli that are not ventilated (e.g., intrapulmonary shunts). Shunts lower the final arterial oxygen saturation. The usual degree of shunt in a newborn is 15% to 20% of the cardiac output.
Acid-Base and Oxygenation Disorders
Ventilation is defined as the amount of gas leaving the mouth per unit of time (e.g., minute ventilation). Minute ventilation is equal to the product of the tidal volume and respiratory frequency in breaths per minute. Tidal volume can be divided into (1) gas in the airway plus the gas in nonperfused alveoli (this is called physiologic dead space) and (2) gas in the alveolar space involved in gas exchange. 3,8Alveolar ventilation is defined as the ratio of CO 2 production by the body to the Pa co2. Pa co2 indicates the magnitude of alveolar ventilation and is inversely related to it such that if Pa co2 doubles, alveolar ventilation is one half of the original value. If the Pa co2 triples, alveolar ventilation in one third of the original value, and so forth.1
RESPIRATORY ACIDOSIS
When the lungs become less effective at removing carbon dioxide, Pa co2 increases and respiratory acidemia ensues. Causes of respiratory acidosis can be separated into pulmonary and nonpulmonary causes.8 The most common pulmonary cause of respiratory acidosis is obstructive lung disease, such as meconium aspiration30 and transient tachypnea20 of the newborn. Obstructive lung disease is also found in the recovery phase of uncomplicated respiratory distress syndrome (RDS) and in bronchopulmonary dysplasia. 24 Also, included in the pulmonary causes of hypoventilation are conditions that interfere with expansion of the lungs, such as diaphragmatic hernia, phrenic nerve paralysis, or pneumothorax. These limit tidal volume. 8
A nonpulmonary cause of carbon dioxide retention is poor respiratory effort. Decreased respiratory drive may be secondary to narcosis because of maternal anesthesia before delivery, depressed respiratory drive because of sepsis, intracranial hemorrhage, including intraventricular hemorrhage, and metabolic disturbances, such as hypoglycemia, affecting the respiratory center. 8
RESPIRATORY ALKALOSIS
In respiratory alkalosis, carbon dioxide clearance is increased and thus Pa co2 is below normal.11 Respiratory alkalosis occurs as a result of hyperventilation, which may be caused by (1) overly vigorous ventilator therapy; (2) central nervous system (CNS) stimulation of the respiratory drive (e.g., hyperammonemia from a genetic abnormality of the urea cycle) 4; and (3) hypoxemia, which stimulates respiratory centers through chemoreceptors. 11
NONRESPIRATORY (METABOLIC) ACIDOSIS
In nonrespiratory (metabolic) acidosis, the metabolic component results from either adding nonvolatile acid (an acid other than carbonic acid) or losing base (bicarbonate).19,25,28 Nonvolatile acids include lactic acid in circulatory shock and hypoxia, organic acids in inborn errors of metabolism, and ketoacids in diabetic acidosis. Loss of base occurs in renal tubular acidosis (a defect in the ability of the renal tubules to reabsorb bicarbonate), with diarrhea with loss of bicarbonate in the feces, or through urinary excretion from the effects of the diuretic acetazolamide (Diamox). 26,27,29
Measurement of the anion gap helps identify the mechanism of metabolic acidosis.6,9,14,29 The anion gap is variably calculated as the serum sodium concentration minus the serum chloride concentration minus the serum bicarbonate concentration, 17,29 or, alternately, sodium plus potassium minus chloride minus bicarbonate. * The upper limit of the normal anion gap with the first method is given as 14 mEq/L29 and with the second method as 15 mEq/L. 13 Addition of nonvolatile acids is associated with an increased anion gap. Loss of base or excess chloride [Cl −] is the likely mechanism of acidosis with a normal anion gap. 23,29 An advantage of measuring the anion gap in understanding the effect of excessive chloride administration is clear. Given that there must be balance between blood cations and anions to preserve electroneutrality, [Cl −] in excess simply displaces [
], resulting in metabolic acidosis. 29 In normal anion gap acidosis, low serum potassium indicates loss of base (e.g., diarrhea) and high serum potassium points to a renal defect (e.g., renal tubular acidosis). 17

Albumin is a major component of the anion gap. Hypoalbuminemia, common in critically ill neonates and children, may mask the presence of the anions of lactic and organic or other nonvolatile acids. 6,7,9,13,17 A “normal” anion gap in combination with low serum albumin indicates that a nonvolatile acid anion is making up the difference for “absent” anions that albumin would ordinarily provide. Thus hypoalbuminemia may hide a metabolic acidosis. Correcting the anion gap for hypoalbuminemia is accomplished by adding 2.5 mEq/L to the anion gap for every g/dL that the concentration of serum albumin is reduced below the normal value of approximately 3.5 g/dL. 6,27
NONRESPIRATORY (METABOLIC) ALKALOSIS
Nonrespiratory (metabolic) alkalosis is caused by either a loss of acid or addition of base, principally bicarbonate.15 Bicarbonate addition is probably iatrogenic secondary to administration of sodium bicarbonate. Alkalosis also occurs when excessive amounts of acetate, citrate, or lactate are given; metabolism of these anions in the liver generates bicarbonate. Loss of acid occurs with nasogastric suctioning or prolonged vomiting with pyloric stenosis. Acid loss by renal mechanisms can occur through the influence of diuretics, digitalis, and corticosteroids. 11 Urine electrolytes, especially chloride, are useful in the differential diagnosis of metabolic alkaloses. Low urine Cl − (<20 mEq/L) is associated with chloride (saline)-responsive metabolic alkalosis from acid loss (e.g., vomiting, nasogastric suction), whereas high urine Cl − is associated with chloride (saline)-unresponsive metabolic alkalosis from renal acid loss (e.g., diuretics). 9,17
OXYGENATION
Although delivery system failure (heart failure), anemia, abnormal hemoglobin affinity for oxygen, and hypoxemia (decreased Pa o2) may cause tissue hypoxia, hypoxemia results only from lung disease or cyanotic congenital heart disease. The most common lung abnormality is mismatched ventilation and perfusion.3 Perfect matching of ventilation and perfusion would occur if all alveoli were perfectly oxygenated and ventilated and supplied with an appropriate amount of pulmonary capillary blood. This ideal situation rarely applies. There is always some degree of ventilation and perfusion mismatch. Two extreme examples are (1) ventilated and oxygenated alveoli without perfusion (e.g., pulmonary emboli) and (2) perfused but nonventilated alveoli (atelectasis). The former is an example of wasted ventilation, and the latter represents an intrapulmonary shunt. Either extreme is incompatible with life. Clinically relevant degrees of ventilation-perfusion mismatch lie somewhere between those extremes. 3
Hypoxemia resulting from ventilation-perfusion mismatch can be overcome with supplemental inspired oxygen. An increased inspired oxygen concentration will eventually displace nitrogen from even the most poorly ventilated alveoli, and alveolar and then arterial oxygen tension will increase. However, an extrapulmonary shunt bypasses the lungs. Pa o2 cannot increase. This is why placing a neonate in 100% oxygen helps separate lung disease from cyanotic congenital heart disease as a cause of hypoxemia.
To perform the “shunt test,” the caretaker should place the hypoxemic neonate in 100% oxygen; if Pa o2 rises to more than 150 mm Hg, cyanotic congenital heart disease is very unlikely.
Central hypoventilation from narcosis may cause hypoxemia. As alveolar carbon dioxide rises, P ao2 falls and Pa o2 decreases. This condition should be clinically evident and should not be confused with lung or congenital heart disease. Other causes of hypoxemia are sufficiently rare in the infant that we need only mention them: decreased inspired oxygen tension, as with increasing altitude, and oxygen diffusion limitation. Diffusion limitation is not clinically important in neonatology.
PREVENTION
Prevention of acid-base and oxygenation disturbances and maintenance of acid-base homeostasis require attention to detail. Prevention of premature births or transport of pregnant women who may deliver a high-risk infant to tertiary care centers for treatment can minimize perinatal asphyxia and its consequences.
With respiratory disturbances, immediate assessment and prompt therapy, including supplemental inspired oxygen and assisted ventilation, help avoid oxygenation and respiratory component of acid-base disturbances (see Chapter 23). Careful monitoring of fluid and electrolyte intake and output, minimizing blood loss, and observing for sepsis help the clinician prevent development of nonrespiratory acid-base disturbances (see Chapter 14).
DATA COLLECTION
Monitoring inspired oxygen concentrations and arterial oxygen tension and supplying appropriate concentrations of additional inspired oxygen will prevent hypoxemia (see Chapter 23). Monitoring may be accomplished intermittently through indwelling arterial catheters or continuously by transcutaneous oxygen monitors and pulse oxygen saturation devices (see Chapter 7). Monitoring hemoglobin concentrations and blood loss, with appropriate replacement, helps ensure adequate blood oxygen content.
Reviewing the patient’s history, performing a physical examination, and evaluating laboratory data augment each other in the assessment of disturbances in acid-base homeostasis and oxygenation (Box 8-2).
BOX 8-2
1. History
a. Obstetric and perinatal
b. Neonatal
c. Family
2. Physical examination
a. Vital signs
b. General appearance
c. Respiratory effort
d. Pulmonary examination
e. Cardiac examination
f. Abdominal examination
g. Neurologic examination
3. Laboratory
a. Chest x-ray film
b. Arterial blood gases
c. Urinalysis
d. In selected cases: sepsis evaluation, serum electrolytes, serum albumin, urine electrolytes, and urine osmolality
History
An adequate obstetric and perinatal history may warn of potential acid-base and oxygenation disturbances:
• Premature delivery predisposes the infant to shock and respiratory distress.
• Meconium staining may portend respiratory difficulties.
• Prolonged rupture of membranes, maternal diabetes, or abnormal maternal bleeding may be associated with either metabolic or respiratory acid-base disturbances and hypoxemia.
• A neonatal history of vomiting, diarrhea, or other gastrointestinal disturbances can cause acid-base disturbances.
• The infant’s general appearance, feeding habits, and activity level may indicate sepsis or CNS injury, both of which promote acid-base disturbances and hypoxemia.
• Nosocomial infections and pneumonia may significantly influence acid-base and oxygenation disturbances.
• A family history of inherited renal problems such as tubular acidosis may suggest an acid-base disturbance.
• A family history of salt-losing endocrinopathies may produce an acid-base disturbance.
Physical Examination
SIGNS AND SYMPTOMS
Signs of acid-base disturbance vary widely and often go undetected. Hypothermia and low blood pressure should alert caretakers to the possibility of metabolic acidosis. Altered respiratory rate and pattern, grunting respirations, nasal flaring, and chest wall retractions raise the possibility of respiratory acidosis or respiratory compensation for metabolic acidosis or may indicate abnormal oxygenation. Abnormalities on auscultation of the heart may point to congenital heart disease and resulting acid-base and oxygenation abnormalities. Lethargy, seizures, and abnormal neurologic signs increase concern for acid-base disturbances or hypoxemia.
Laboratory Data
Chest Radiograph: A chest x-ray examination may identify a respiratory cause for acid-base disturbance and hypoxemia.
Urinalysis: The routine urinalysis records urine specific gravity and demonstrates that urine is being produced. Urine electrolytes are helpful in differentiating among the pathophysiologic mechanisms of metabolic derangements.
Arterial Blood Gases: Interpretation of the arterial blood gases will point to the primary acid-base derangement and may reveal a secondary compensation and define the degree of hypoxemia. 3,16,21,31 Presently, methods for monitoring the components of acid-base analysis comprise both invasive and noninvasive techniques. Intermittent arterial punctures or indwelling catheters in various vessels (often the umbilical artery or vein) supply data. However, we can continuously measure transcutaneous P o2 or O 2 saturation. Monitors can continuously measure expired end-tidal CO 2, which corresponds to the alveolar CO 2. (Alveolar and arterial CO 2 are equivalent unless respirations are excessively rapid.) In addition, skin electrodes are available that measure Pa o2 and Pa co2 (see Chapter 7).
Although the pathophysiologic condition of the acid-base disturbance is determined through the analysis of arterial blood gases, further assessment of the infant is necessary, as follows:
• Respiratory alkalosis or acidosis should be suggested by physical examination, arterial blood gas analysis, and chest x-ray examination.
• Metabolic acidosis often accompanies shock and septicemia. The anion gap and urine electrolytes may provide additional information to delineate causes. Blood pressure measurement, a complete blood cell count, serum and urine electrolytes, serum albumin and glucose determinations, and assessment of intake and output of fluids are often needed to identify the source of a metabolic acidosis.
• Oxygenation disturbances may be analyzed from the preceding laboratory tests, and when indicated, electrocardiogram and arterial blood gas response to increased inspired oxygen concentrations are used to evaluate the possibility of congenital heart disease.
Another calculation, the oxygenation index (OI), is used to assess critically ill neonates receiving ventilator therapy. The OI is (Fio 2 × 100 × mean airway pressure) ÷ Pa o2. An OI of 25 or greater has been considered an indication for extraordinary ventilatory support, such as inhaled nitric oxide or extracorporeal membrane oxygenation (ECMO).
TREATMENT
In respiratory acidosis, the pathophysiologic mechanism is decreased alveolar ventilation. Treatment is directed at the underlying cause. 8Hypoxemia caused by ventilation-perfusion mismatch is treated with increased inspired oxygen concentration. Techniques that may be of benefit include continuous positive airway pressure (CPAP), standard ventilation, high-frequency ventilation, ECMO, inhaled nitric oxide, and others (see Chapter 23). Treatment of respiratory alkalosis usually consists of reducing ventilator settings. One of the few causes of central hyperventilation is hyperammonemia caused by an inborn error of urea cycle metabolism4 (Chapter 27). Prompt recognition is crucial to early diagnosis and treatment.
Asphyxia often leads to combined respiratory and metabolic acidosis. Ventilation will resolve the respiratory acidosis. Improved oxygenation usually allows lactic acidosis to resolve without bicarbonate therapy. In narcosis, temporary ventilator support may be necessary. The narcosis may be reversed with administration of naloxone (Narcan) at a dose of 0.1 mg/kg if the possibility of maternal opiate drug abuse has been ruled out. (Repeated doses may be necessary; see Chapter 4.)
With any acidosis and alkalosis, a careful search must be instituted for causes. If the cause of metabolic acidosis is septicemia, intestinal necrosis, or poor cardiac output severe enough to result in metabolic acidosis, successful treatment of the cause is of far more importance than buffer therapy for acidosis. *Sodium bicarbonate administration was once thought to be vitally important to management of metabolic acidosis. Use in neonatal intensive care has decreased dramatically as shortcomings have emerged, especially the possibility that “push” administration may cause intraventricular hemorrhage.19,22It should not be used if severe lung disease restricts carbon dioxide elimination (seeEquation 3).
COMPLICATIONS
The outcome of unrecognized and untreated acid-base or oxygenation disturbances may be an increased mortality or an increased morbidity in the survivors. Complications of the correction of the acid-base and oxygenation disturbance vary according to the disturbance and treatment provided.
One effect of acidosis is CNS depression. In metabolic acidosis, the rate and depth of respiration are increased, whereas in respiratory acidosis, respiration may be labored or depressed. An effect of alkalosis is increased excitability of the CNS and tetany (often of the respiratory muscles). 8,9,15,26 Complications associated with sodium bicarbonate therapy for metabolic acidosis are discussed above.
Treatment of respiratory acidosis by assisted ventilation can produce all of the complications of assisted ventilation, including infection, trauma, oxygen toxicity, sepsis, air leak, and subglottic stenosis (see Chapter 23).
Complications of oxygen therapy include hypoxemia and hyperoxemia. Severe hypoxemia may cause pulmonary vasoconstriction, a change from aerobic to anaerobic metabolism (with eventual metabolic acidosis), cyanosis, bradycardia, hypotonia, and decreased CNS and cardiac function. Prolonged high inspired oxygen concentrations can result in pulmonary oxygen toxicity and contribute to retinopathy of prematurity. If ventilatory support is necessary to achieve adequate oxygenation, one must deal with the additional complications of ventilator therapy (see Chapter 23).
PARENT TEACHING
Obtaining blood for blood gas analysis by invasive techniques (arterial punctures and heel sticks) is stressful for parents and their infant. Explaining the rationale for the test, eliciting parental assistance (if they are present), and encouraging them to comfort their crying baby involve parents as primary caregivers. Explaining the results of the analysis and needed changes in therapy keeps parents apprised of their baby’s progress. Many parents become quite adept at blood gas interpretation and are able to anticipate therapeutic alterations: “Did you change the F io2? The ventilator rate?” This helps parents master a difficult situation. Beyond sharing technical information, the care provider should also personalize the infant to his or her parents (see Chapter 29).
REFERENCES
1. Adrogué, H.E.; Adrogué, H.J., Acid-base physiology, Respir Care 46 (2001) 328.
2. Boyle, M.; Lawrence, J., An easy method of mentally estimating the metabolic component of acid/base balance using the Fencl-Stewart approach, Anaesth Intensive Care 31 (2003) 538.
3. Breen, P.H., Arterial blood gas and pH analysis: clinical approach and interpretation, Anesthesiol Clin North Am 19 (2001) 885.
4. Brusilow, S.W., Hyperammonemic encephalopathy, Medicine 81 (2002) 240.
5. Delivoria-Papadopoulos, M.; Roncevic, N.P.; Oski, F.A., Postnatal changes in oxygen transport of term, premature, and sick infants: the role of red cell 2,3-diphosphoglycerate and adult hemoglobin, Pediatr Res 5 (1971) 235.
6. Dubin, A.; Menises, M.M.; Masevicius, F.D.; et al., Comparison of three different methods of evaluation of acid base disorder, Crit Care Med 35 (2007) 1264.
7. Durward, A.; Mayer, A.; Skellett, S.; et al., Hypoalbuminaemia in critically ill children: incidence, prognosis, and influence on the anion gap, Arch Dis Child 88 (2003) 419.
8. Epstein, S.K.; Singh, N., Respiratory acidosis, Respir Care 46 (2001) 366.
9. Fencl, V.; Jabor, A.; Kazda, A.; et al., Diagnosis of metabolic acid-base disturbances in critically ill patients, Am J Respir Crit Care Med 162 (2000) 2246.
10. Finer, N.N.; Barrington, K.J., Nitric oxide for respiratory failure in infants born at or near term, Cochrane Database Syst Rev 4 (2006); CD000399.
11. Foster, G.T.; Vaziri, N.D.; Sassoon, C.S., Respiratory alkalosis, Respir Care 46 (2001) 384.
12. Gunnerson, K.J.; Kellum, J.A., Acid-base and electrolyte analysis in critically ill patients: are we ready for the new millennium?Curr Opin Crit Care 9 (2003) 468.
13. Hatherill, M.; Waggie, Z.; Purves, L.; et al., Correction of the anion gap for albumin in order to detect occult tissue anions in shock, Arch Dis Child 87 (2002) 526.
14. Kellum, J.A., Clinical review: reunification of acid-base disorders, Crit Care 9 (2005) 500.
15. Khanna, A.; Kurtzman, N.A., Metabolic alkalosis, Respir Care 46 (2001) 354.
16. Kirksey, K.M.; Holt-Ashley, M.; Goodroad, B.K., An easy method for interpreting the results of arterial blood gas analysis, Crit Care Nurs 21 (2001) 49.
17. Kraut, J.A.; Madias, N.E., Approach to patients with acid-base disorders, Respir Care 46 (2001) 392.
18. Kurtz, I.; Kraut, J.; Ornekian, V.; et al., Acid-base analysis: a critique of the Stewart and bicarbonate-centered approaches, Am J Physiol Renal Physiol 294 (2008) F1009.
19. Levraut, J.; Grimaud, D., Treatment of metabolic acidosis, Curr Opin Crit Care 9 (2003) 260.
20. Sandberg, K.; Sjöqvist, B.A.; Hjalmarson, O.; et al., Lung function in newborn infants with tachypnea of unknown cause, Pediatr Res 22 (1987) 581.
21. Shoulders-Odom, B., Using an algorithm to interpret arterial blood gases, Dimens Crit Care Nurs 19 (2000) 36.
22. Simmons, M.A.; Adcock, E.W.; Bard, H.; et al., Hypernatremia and intracranial hemorrhage in neonates, N Engl J Med 291 (1974) 6.
23. Sirker, A.A.; Rhodes, A.; Grounds, R.M.; et al., Acid-base physiology: the ‘traditional’ and the ‘modern’ approaches, Anaesthesia 57 (2002) 348.
24. Sivieri, E.M.; Bhutani, V.K., Pulmonary mechanics, In: (Editors: Sinha, S.K.; Donn, S.M.) Manual of neonatal respiratory care ( 2000)Mosby, Armonk, NY.
25. Story, D.A.; Morimatsu, H.; Bellomo, R., Strong ions, weak acids and base excess: a simplified Fencl-Stewart approach to clinical acid-base disorders, Br J Anaesthesiol 92 (2004) 54.
26. Swenson, E.R., Metabolic acidosis, Respir Care 46 (2001) 342.
27. Van Gosen, L., Organic acidemias: a methylmalonic and propionic focus, J Pediatr Nurs 23 (2008) 225.
28. Whittier, W.L.; Rutecki, G.W., Primer on clinical acid-base problem solving, Dis Mon 50 (2004) 122.
29. Wilson, W.C., Clinical approach to acid-base analysis: importance of the anion gap, Anesthesiol Clin North Am 19 (2001) 907.
30. Wiswell, T.E.; Srinivasan, P.; Roberton, N.RC., Aspiration syndromes, In: (Editors: Greenough, A.; Milner, A.D.) Neonatal respiratory disorders ( 2003)Arnold, London.
31. Woodrow, P., Arterial blood gas analysis, Nurs Stand 18 (2004) 45.