Chapter 122 Wound Closure
Soft Tissue Anatomy
The first layer deep to the skin is the subcutaneous tissue. Within this layer can be found a thin membranous layer of fascia,1 recently termed the iliolumbar membrane, in the back.2 Deep to the subcutaneous tissue in the cervical spine are three layers of musculature, of which the trapezius makes up the superficial group. This muscle has descending, transverse, and ascending portions originating from the superior nuchal line of the skull, the ligamentum nuchae, the spinous processes of C7-T12, and the supraspinous ligament.3 Immediately deep to the trapezius in the midline is the ligamentum nuchae. It is the cervical extension of the supraspinous ligament, attaches to the inion,4 and has connections with the lesser posterior rectus, splenius, semispinalis capiti, and the lesser rhomboids.5 The intermediate cervical muscular layer includes the splenius capitis and cervicis; semispinalis capitis and cervicis; and longissimus capitis and cervicis.5 The splenius muscles originate from the ligamentum nuchae and the spinous processes of the lower cervical and upper thoracic vertebrae, whereas the longissimus and semispinalis muscles originate from the cervical and upper thoracic transverse processes.5 The deep cervical musculature includes the multifidus, rotatores, spinalis, and interspinales groups.3 Rostral to C2 the deep musculature is termed the suboccipital musculature and consists of the rectus and obliquus capitis groups.3,6
The superficial muscular group of the thoracolumbar region includes the trapezius, described in the preceding paragraph, and the latissimus dorsi, originating from the dorsal layer of the thoracolumbar fascia from the spinous processes of T7 to the sacrum and the iliac crest.3 The thoracolumbar fascia is multilayered and arises from the aponeurosis of the transversus abdominis.4,6 The thoracolumbar fascia attaches medially to the spinous processes of the lumbar and sacral vertebrae and caudally to the iliac crests. Laterally, it attaches to the ribs and intercostal fascia. The dorsal layer of the thoracolumbar fascia is termed the lumbar aponeurosis and serves as the origin of the latissimus dorsi muscle. The intermediate muscles of the trunk include the rhomboids and serratus posterior.4 The deep muscles of the back are divided into lateral and medial tracts. The iliocostalis, longissimus, and intertransversarii muscles make up the lateral group; the rotatores, multifidus, and interspinales make up the medial group.3 The trapezius muscle spans the entire breadth of the interscapular distance and extends from the subocciput to T12. The latissimus dorsi muscle likewise extends from T7 caudally to the sacrum. By virtue of their location and breadth, they provide a ready mechanism for the closure and repair of open spinal wounds.
Wound Closure
Dorsal Spinal Wounds
After dural closure, inspection of the deep tissues, including the resected vertebral bone edges, epidural contents, and surrounding musculature, should be performed to ensure that meticulous hemostasis has been achieved. Failure to do so may lead to postoperative hematomas, which can become symptomatic due to compression of neural structures. Epidural bleeding should be controlled with bipolar cautery or local hemostatic agents, the exposed bone edges waxed, and muscular bleeding likewise controlled with cautery. Irrigation with hydrogen peroxide often is performed in cases without dural violation, particularly in large wounds, to aid in hemostasis. Once this has been achieved, some surgeons also cover exposed dura with a local hemostatic agent.
One of the central tenets of wound approximation is the use of an orderly layered closure to optimize wound healing by eliminating dead space to reduce the risk of fluid collection and infection. After the dura has been closed and hemostasis achieved, attention should be turned to the layered closure. After extensive spinal procedures, a large potential dead space exists that is amplified in patients who have undergone laminectomy at multiple contiguous levels. The paraspinal musculature is approximated with a few large absorbable #0 sutures, with care taken not to strangulate the tissue, which can cause local muscle necrosis and severe postoperative pain. Fascial closure is performed carefully with interrupted #0 absorbable sutures. In cases of reoperation, or where radiation is anticipated, nonabsorbable braided #0 suture material is recommended. A tight closure of the fascia is recommended to reduce dead space as well as prevent possible CSF leakage. This layer also may serve as a barrier to the development of a deep infection from a superficial wound infection. Placement of a drain into the epidural and/or subfascial space should be considered in those patients with large wounds, after vascular tumor removal, after instrumented fusion procedures, and after operations for trauma. These drains diminish the occurrence of hematomas and seromas that hamper wound healing7,8 and that also can cause neurologic deficit with neural element compression. If CSF is observed accumulating in the suction canister, the drain must be removed immediately to prevent a persistent CSF leak and complications associated with CSF overdrainage.
In larger individuals, it may be necessary to close the subcutaneous tissue in multiple layers owing to its thickness. For this, #2-0 Vicryl suture is commonly used, and the membranous superficial fascia, most easily identified in the lumbar subcutaneous tissue,1,2 should be the target of reapproximation. The dermal tissue is reapproximated next with inverted interrupted #2-0 or #3-0 suture material, taking care to align the edges of the wound in the rostral-caudal as well as dorsal-ventral dimensions. It is important to produce wound eversion with closure of this layer to ensure the skin layer will have minimal tension after its closure, thereby improving its ability to heal. Scars from properly everted wounds tend not to widen with time, and the ridge of everted tissue always settles to normal.9 The skin is reapproximated with either monofilament suture, staples, or, in some cases, skin adhesive application.
Ventral Spinal Wounds
In the presence of wound dehiscence or infection before attempted closure, extensive debridement is necessary. In cases in which radiation therapy has been received preoperatively or is anticipated postoperatively, closure of the fascia should be accomplished with nonabsorbable #0 or #2 monofilament or braided nylon. Wound closure in general is uncomplicated in the cervical and lumbar region because of the thickness of the muscle layer and the lordotic curvature of the spine. An incision in the upper thoracic spine is subject to horizontal tensile forces caused by shoulder movement, and this area is notorious for dehiscence in as many as 30% of patients with cancer or spinal implants.10 In addition to spinous process excision, a figure-8 brace may be worn postoperatively to reduce tensile forces across the vertical incision. Drains usually are left in place for 2 days, or until the drainage is less than 50 mL per 8-hour shift. Sutures are not removed until at least 2 weeks postoperatively.
Myocutaneous Flaps
Closure of large soft tissue defects currently relies heavily on the use of myocutaneous and fasciocutaneous flaps. In the back, the most commonly used musculocutaneous flap for defects of the upper third of the thoracic spine is the trapezius muscle. Variations of flap design can cover upper-third defects over a relatively wide arc of rotation, as long as the transverse cervical artery is intact. Defects in the middle third of the back are most commonly closed using the latissimus muscle, a choice based on its thoracodorsal blood supply or on the paraspinous perforators. Defects of the lower third of the back often are closed with gluteus muscle myocutaneous flaps, most often based on the inferior gluteal artery. In the lower third, the latissimus dorsi muscle also may be used. Its use must be based on a free flap using vein grafts from the thoracodorsal trunks or by anastomosis to the superior or inferior gluteal vessels, if it is to reach the caudal-most portions of the lower third of the back.
Trapezius Muscle and Myocutaneous Flaps
Because of its length, extending from the superior nuchal line to the spinous process of T12, and its width, from one acromial process to the other, the trapezius muscle is suitable for rotation, with or without overlying flaps of skin (Fig. 122-1). The blood supply to the trapezius muscle is via the type II vascular pattern characterized by one dominant vascular pedicle with other minor contributing pedicles.11–13 The principal pedicle consists of the dorsal scapular artery, which constitutes the descending branch of the transverse cervical artery. The latter arises from the subclavian artery or thyrocervical trunk. The dorsal scapular artery courses caudally, medial to the scapula and ventral to the rhomboid muscle, supplying these muscles as well as the latissimus dorsi and trapezius muscles and eventually anastomosing with the suprascapular and subscapular arteries and some of the intercostal arteries. The minor pedicle consists of branches of the occipital artery perfusing the rostral segments of the muscle.7,14 With careful dissection, the caudal pole of the trapezius muscle can be dissected from the spinous processes and underlying latissimus and rhomboid muscles. Careful attention must be paid to preserve the integrity of its blood supply, which can be observed on the ventral aspect of the caudal and medial fibers.
Trapezius flaps, with or without attached cutaneous pedicles, can be used to repair defects overlying the kyphotic curvature of the thoracic spine. Spinal wound closure can be a challenge in patients who are being treated for thoracic spinal metastases. The apex of the thoracic kyphosis is most prone to dehiscence in patients whose wound healing capability has been compromised by chemotherapy and radiation therapy. Radiation therapy contributes to impairment of circulation by virtue of endothelial swelling and subintimal fibrosis. This results in an obstructive endarteritis with the subcutaneous tissue being replaced by dense fibrosis.7 Shoulder movement further contributes traction on the skin edges, increasing their predilection for separation and dehiscence. This is further expedited in cases of wound infection or diabetes or in elderly patients. When such wound dehiscence fails to heal by secondary intention, alternative approaches must be sought. Failure of healing with superimposed infection can result in dural erosion and CSF leaks. Spinal drainage is insufficient to provide for dural closure in the depths of an open infected wound. Under those circumstances, extensive debridement of the wound in the operating room is necessary. Excision of all infected bone is undertaken until bleeding cancellous bone is encountered. Dural repairs are undertaken with free or pedicle fascial grafts attached to a trapezius flap. The trapezius flap can obliterate the dead space in the depths of the spinal wound, providing for an optimal watertight closure. The wound is further approximated in layers, usually over drains. The approximation usually is undertaken with heavy, nonabsorbable sutures such as #0 or #2 braided nylon, and the skin is closed with heavy #0 Prolene monofilament. It is important to maintain optimum nutritional status of the patient to enhance healing. In the presence of anemia (hemoglobin <8 g/dL), blood transfusions also will contribute to increased activity and, potentially, to wound healing. The patient is maintained in a figure-8 brace for 4 to 8 weeks.
Latissimus Flaps
The latissimus dorsi muscle, because of its location and blood supply, provides muscle or myocutaneous flaps for the closure of spinal defects overlying the lower thoracic and lumbar spine (see Fig. 122-1). Its blood supply is type V, consisting of one dominant vascular pedicle, which in this case is the thoracodorsal artery arising from the subclavian artery and secondary midline and paramedian pedicles. The thoracodorsal artery consists of the dorsal cutaneous branches of the intercostal and lumbar arteries.7,11 The intercostal arteries that contribute most to the secondary pedicles are the 9th, 10th, and 11th intercostal arteries, as confirmed on cadaveric dissections.15 These perforators usually enter the latissimus muscle approximately 5 cm from the midline. Latissimus dorsi flaps, with and without skin, are based on the thoracodorsal artery and are dissected away from the spinous process attachments. Such flaps generally are suited for repair of large thoracic wall or lateral iliac decubiti.
Reverse latissimus flaps, on the other hand, are dissected laterally or rostrally and thus maintain the blood supply of the flap from the midline paraspinal secondary vascular pedicles. The integrity of the penetrating vessels on the ventral surface of the muscle is preserved because the supply from the thoracodorsal artery is sacrificed for distal mobilization. Such latissimus dorsi flaps are referred to as reverse flaps and are suited for the repair of spinal defects of the lower thoracic and lumbar spines (Fig. 122-2). The muscle flap is based medially on the 9th, 10th, and 11th intercostal feeders.
The length of the pedicle is geared to the size of the spinal defect to be repaired. The portion of the latissimus dorsi muscle mobilized and its width are customized to the case at hand (Fig. 122-3). The latissimus dorsi muscle provides a large muscle mass capable of filling far larger spinal defects than is possible with trapezius muscle flaps. The reverse latissimus flap, with or without its cutaneous pedicle, when necessary, is tunneled subcutaneously to the midline incision. The site of the cutaneous pedicle is then repaired with a split-thickness skin graft. Reverse latissimus flaps are usually adequate when used unilaterally.
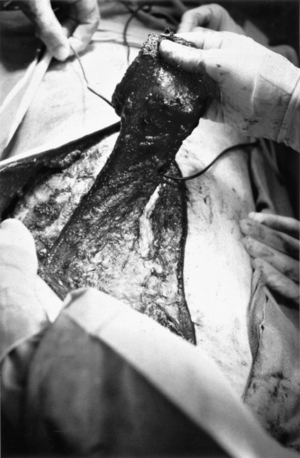
FIGURE 122-3 Surgical exploration was performed in the patient shown in Figure 122-2, and no frank cerebrospinal fluid leak was identified. This intraoperative photograph was taken while standing at the foot of the table looking toward the head. A reverse latissimus dorsi flap was harvested from the left side and used for obliteration of the dead space. The midline incision was extended rostrally, and the latissimus muscle sectioned below its insertion. The muscle head is held up and reflected into the lumbosacral incision while safeguarding its vascular supply. On follow-up, she was asymptomatic, and her lower back was flat without reaccumulation of the meningocele.
Bilateral large musculocutaneous latissimus flaps may be advanced medially to cover large midline spinal defects. In these circumstances, the flaps are based on the thoracodorsal artery with partial preservation of the secondary intercostal blood supply. Skin grafting may be necessary to cover the relaxing incisions made on either side of the torso to facilitate advancement.10
It is possible to create a large muscle or skin and muscle flap using the latissimus dorsi muscle based on a vein graft extension of the thoracodorsal artery.12 This approach requires a microsurgical team and the harvest of saphenous or other suitable vein to interpose on both the arterial and venous circulation. The vein interposition can be performed below the subscapularis branch, and a large muscle advancement can be carried out. This overcomes the problems that occasionally occur when using the most medial portions of the flap with complete ligation of the paraspinous perforators. This medial-most portion of the flap is primarily fascial and of marginal circulation. It depends entirely on the thoracodorsal vessel for inflow. Use of the vein graft and transection of the insertion of the muscle permits safe movement of more reliable muscle and skin medially. A “V-to-Y” technique allows movement of relatively large skin-muscle flaps, when such flaps are needed. This technique also can be used to advance only the latissimus dorsi muscle, and the epithelial coverage is provided by application of a split-thickness graft to the exposed portion of the latissimus muscle.
Gluteus Maximus Flaps for the Sacral Region
The gluteus maximus is a large buttock muscle with a quadrilateral shape. It extends from the ilium, sacrum, coccyx, and sacrospinal aponeurosis to the greater trochanter of the femur and the iliotibial band of the fascia lata.10 This large muscle has a type III vasculature, with two dominant arteries, the superior and inferior gluteal arteries, arising from the internal iliac artery.11,16 Because of its location, the gluteus maximus muscle, with or without an attached skin flap, is suited for repair of sacral, ischial, trochanteric, and ilial open wounds. The muscle mass and overlying skin have sufficient size and redundancy that a unilateral myocutaneous flap often is sufficient to provide coverage for a midline defect. For the coverage of a sacral decubitus, the skin incision extends along the caudal margin of the iliac crest laterally from the edges of the wound that had been debrided. The incision is carried through the subcutaneous tissue, and a plane is created between the gluteus maximus and medius muscles. It is important to maintain the integrity of the superior gluteal artery as it enters the deep surface of the gluteus maximus muscle. However, if the superior gluteal artery interferes with the mobility of this muscle flap, it can be sacrificed for increased mobility. In instances in which this has been done, the blood supply provided by the inferior gluteal artery has proven sufficient.16 The muscle and skin edges are then sutured to the contralateral debrided edge of the exposed wound after incision of all infected and devascularized bone. Depending on the size of the defect to be covered, the lateral caudal margin of the myocutaneous flap can extend inferiorly to the greater trochanter. The gluteus medius muscle provides adequate soft tissue padding of the iliac crest. The donor site of the skin is covered with a split-thickness skin graft. Vacuum drains usually are sufficient to avoid the accumulation of hematomas and seromas that can complicate and impede healing. The muscle flap usually is sutured in place with heavy-gauge absorbable suture such as #0 and #2 Vicryl, and the skin is sutured with monofilament nylon or Prolene. Heavy dressings are applied to prevent pressure on the flap that may impair adequate perfusion. The patient is to be kept on the side or in the prone position for at least 2 weeks subsequent to flap rotation.
The rotational advancement of gluteus-based flaps for sacral wounds has been largely replaced by V-to-Y techniques. The sacral lesion is debrided, and depending on the transverse diameter of the wound, either a single or a bilateral V incision is designed. The origins of the gluteus maximus are freed up as needed, and the muscle is dissected free at the lateral margin, dissecting medially as needed to free the flap. The superior or inferior gluteal artery must be kept intact. This myocutaneous flap is then advanced medially and sutured to the wound edge or to its mirror image flap from the opposite side, if the size of the wound dictates a bilateral approach. As the lateral portion of the flap is moved by this advancement, a linear closure of the defect results in the Y-shaped final appearance as observed in Figures 122-4 and 122-5.
Nonhealing Spinal Wounds
Impediments to wound healing are numerous and can be challenging to overcome when faced with a nonhealing spinal wound. Nonhealing wounds are costly not only in terms of dollars to the health care system but also, more importantly, in terms of the morbidity and potential for mortality for the affected patient.17 Risk factors for poor healing include but are not limited to nutritional status, corticosteroid use, diabetes mellitus, history of radiation treatment, a variety of collagen disorders, smoking, immunosuppressant therapy, infection, neurologic deficit resulting in inability for the patient to change positions, and tissue hypoxia.18–20 It is advantageous for the surgeon and the patient to address as many factors preoperatively as possible.
Strategies for Nonhealing Wounds
Strategies for addressing large tissue defects with flaps and grafts have been previously described. Additional strategies for nonhealing wounds include the use of vacuum-assisted closure (VAC) devices and hyperbaric oxygen therapy. Wound VAC therapy, also referred to as negative pressure wound therapy, involves placing a nonbioabsorbable sponge in the bed of a wound covered with an occlusive dressing connected to a suction device with a fluid collection chamber. Wound healing is enhanced by removal of wound fluid, increased angiogenesis, and up-regulation of several tissue factors.21,22 Wound VACs have been used not only in chronic nonhealing wounds but also in cases of trauma, fasciotomy, and infection.23–27 A variety of surgical specialties use these devices with seemingly good results,23,27–29 and some studies have shown cost savings.30,31 Despite their increased use, however, there are very few randomized controlled trials evaluating their efficacy. A recent Cochrane Review found insufficient evidence that wound VAC use improves healing of chronic wounds.32
Hyperbaric oxygen therapy (HBOT) is another useful adjunct in treating nonhealing wounds. HBOT involves placing a patient in a hyperbaric chamber where he or she breathes 100% oxygen for brief periods while at an increased barometric pressure. Tissue oxygen tension is more than doubled during these treatments,33 augmenting wound healing, inducing angiogenesis, and aiding neutrophil function.34–37 The plasma becomes supersaturated with oxygen to the point that red blood cells are not required to supply oxygen for cellular respiration.38 HBOT is currently indicated in the treatment of the following: decompression sickness, air embolism, necrotizing fasciitis, clostridial necrosis, carbon monoxide poisoning, crush injuries, problem wounds including diabetic ulcers, refractory osteomyelitis, compromised skin grafts and flaps, thermal burns, delayed radiation injury, exceptional blood loss, and intracranial abscesses.39 The number and length of treatments are dictated by the disease process being treated, the response of the wound to treatment, and patient tolerance. Currently, more than 600 hyperbaric chambers are in use across the United States.39
The usefulness of HBOT is predicated on its ability to supply oxygen to tissues at much higher concentrations than at normobaria. Before therapy is initiated, transcutaneous partial pressure of oxygen (TcPo2) measurements often are made to confirm wound hypoxia and assess whether a wound may benefit from a course of HBOT.40–43 TcPo2 values near the wound are obtained with the patient inspiring air at sea level (21% oxygen), inspiring 100% oxygen at sea level, and during HBOT. If the TcPo2 is in the normal range (≥40 mm Hg) while inspiring air, this confirms that the wound is not hypoxic, and alternative causes of wound nonhealing should be aggressively sought and corrected. When inspiring 100% oxygen at sea level, it has been shown that increases of less than 10 mm Hg in the TcPo2 reliably predict wounds that likely will not respond to HBOT.40 TcPo2 values less than 400 mm Hg during HBOT also have been shown to predict which wounds will not respond to HBOT.41–43
Summary
Understanding spinal fascial and muscular anatomy is crucial when performing spinal wound closure, whether secondary to a microdiscectomy or a complicated reconstruction procedure. Following the basic tenets of wound closure, including debridement of dead tissue, elimination of dead space via an orderly layered closure, maintenance of meticulous hemostasis, and ensuring adequate vascularization of the wound bed, is crucial for optimal wound healing and closure. Large tissue defects may be treated with a variety of myocutaneous flaps, depending on the size, location, and depth of the wound. Adjunctive treatment strategies for nonhealing wounds are increasing, with current emphasis on vacuum-assisted closure devices and hyperbaric oxygen therapy in select cases.
Hunt T.K., Hopf H., Hussain Z. Physiology of wound healing. Adv Skin Wound Care. 2000;13(Suppl 2):6.
Jones G.A., Butler J., Lieberman I., Schlenk R. Negative-pressure wound therapy in the treatment of complex postoperative spinal wound infections: complications and lessons learned using vacuum-assisted closure. J Neurosurg Spine. 2007;6:407.
Mehboud A.A., Ogilvie J.W., Pinto M.R., et al. Postoperative deep wound infections in adults after spinal fusion: management with vacuum-assisted wound closure. J Spinal Disord Tech. 2005;18:14.
Putz R., Pabst R., editors. Sobotta atlas of human anatomy; Volume 2: Trunk, viscera, lower limb. Philadelphia: Lippincott Williams & Wilkins, 2001.
Tibbles P.M., Edelsberg J.S. Hyperbaric oxygen therapy. N Engl J Med. 1996;334:1642.
1. Abu-Hijleh M.F., Roshier A.L., Al-Shboul Q., et al. The membranous layer of superficial fascia: evidence for its widespread distribution in the body. Surg Radiol Anat. 2006;28:606.
2. Loukas M., Louis R.G.Jr., Van der Wall B., et al. Iliolumbar membrane, a newly recognized structure in the back. Folia Morphol. 2006;65:15.
3. Putz R., Pabst R., editors. Sobotta atlas of human anatomy; Volume 2: Trunk, viscera, lower limb. Philadelphia: Lippincott Williams & Wilkins, 2001.
4. Agur A.M.R., Lee M.J., editors. Grant’s atlas of anatomy, ed 10, Philadelphia: Lippincott Williams & Wilkins, 1999.
5. Lang J. Clinical anatomy of the cervical spine. New York: Thieme Medical Publishers; 1993.
6. Drake R.L., Vogl A.W., Mitchell A.W.M., editors. Gray’s anatomy for students, ed 2, Philadelphia: Churchill Livingstone Elsevier, 2010.
7. El-Tamer M.D., Chaglassian T. Wound management in spinal surgery. In: Sundaresan N., Schmidek H.H., Schiller A.L., Rosenthal D.I., editors. Tumors of the spine: diagnosis and clinical management. Philadelphia: WB Saunders, 1990.
8. Zide B.M., Wisoff J.H., Epstein F.J. Closure of extensive and complicated laminectomy wounds: operative technique. J Neurosurg. 1987;67:59.
9. Thorne C.H. Techniques and principles in plastic surgery. In Thorne C.H., editor: Grabb and Smith’s plastic surgery, ed 6, Philadelphia: Wolters Kluwer/Lippincott Williams & Wilkins, 2007.
10. Clemante C.D. Muscles and fascia. In Clemente CD, editor: Gray’s anatomy, ed 13. Philadelphia: Lea & Febiger, 1985.
11. Mathes S.J., Nahai F. Classification of the vascular anatomy of muscles—experimental and clinical correlation. Plast Reconstr Surg. 1981;67:177.
12. Nahai F., Hagerty R. One-stage microvascular transfer of latissimus flap to the sacrum using vein grafts. Plast Reconstr Surg. 1986;77:312.
13. Seyfer A.E., Joseph A.S. Use of trapezius muscle for closure of complicated upper spinal defects. Neurosurgery. 1984;14:341.
14. Clemante C.D. The arteries. In In Clemente CD, editor: Gray’s anatomy, ed 13, Philadelphia: Lea & Febiger; 1985:648.
15. Sheffield P.J. Measuring tissue oxygen tension: a review. Undersea Hyper Med. 1998;25:179.
16. Minami R.T., Mills R., Pardoe R. Gluteus maximus myocutaneous flaps for repair of pressure sores. Plast Reconstr Surg. 1977;60:242.
17. Branski L.K., Gauglitz G.G., Herndon D.N., et al. A review of gene and stem cell therapy in cutaneous wound healing. Burns. 2009;35:171.
18. Stadelmann W.K., Digenis A.G., Tobin G.R. Impediments to wound healing. Am J Surg. 1998;176(Suppl 2A):39S.
19. Hunt T.K., Hopf H., Hussain Z. Physiology of wound healing. Adv Skin Wound Care. 2000;13(Suppl 2):6.
20. de la Torre J.I., Chambers J.A. Wound healing, chronic wounds. Emedicine. October 9, 2008.
21. Moues C.M., van Toorenenbergen A.W., Heule F., et al. The role of topical negative pressure in wound repair: expression of biochemical markers in wound fluid during wound healing. Wound Repair Regen. 2008;16:488.
22. Labler L., Rancan M., Mica L., et al. Vacuum-assisted closure therapy increases local interleukin-8 and vascular endothelial growth factor levels in traumatic wounds. J Trauma. 2009;66:749.
23. Herscovici D Jr, Sanders RW, Scaduto JM, et al. Vacuum-assisted wound closure (VAC therapy) for the management of patients with high-energy soft tissue injuries. J Orthop Trauma 17:683, 2003.
24. Mehboud A.A., Ogilvie J.W., Pinto M.R., et al. Postoperative deep wound infections in adults after spinal fusion: management with vacuum-assisted wound closure. J Spinal Disord Tech. 2005;18:14.
25. Jones G.A., Butler J., Lieberman I., Schlenk R. Negative-pressure wound therapy in the treatment of complex postoperative spinal wound infections: complications and lessons learned using vacuum-assisted closure. J Neurosurg Spine. 2007;6:407.
26. Bollero D., Carnino R., Risso D., et al. Acute complex traumas of the lower limbs: a modern reconstructive approach with negative pressure therapy. Wound Repair Regen. 2007;15:589.
27. Zannis J., Angobaldo J., Marks M., et al. Comparison of fasciotomy wound closures using traditional dressing changes and the vacuum-assisted closure device. Ann Plast Surg. 2009;62:407.
28. Dhir K., Reino A.J., Lipana J. Vacuum-assisted closure therapy in the management of head and neck wounds. Laryngoscope. 2009;119:54.
29. Ennker I.C., Malkoc A., Pietrowski D., et al. The concept of negative pressure wound therapy (NPWT) after poststernotomy mediastinitis—a single center experience with 54 patients. J Cardiothoracic Surg. 2009;4:5.
30. Flack S., Apelgvist J., Keith M., et al. An economic evaluation of VAC therapy compared with wound dressings in the treatment of diabetic foot ulcers. J Wound Care. 2008;17:71.
31. Gabriel A., Shores J., Heinrich C., et al. Negative pressure wound therapy with instillation: a pilot study describing a new method for treating infected wounds. Int Wound J. 2008;5:399.
32. Ubbink D.T., Westerbos S.J., Evans, et al. Topical negative pressure for treating chronic wounds. Cochrane Database Syst Rev. 2007. 4: CD001898,
33. Tibbles P.M., Edelsberg J.S. Hyperbaric oxygen therapy. N Engl J Med. 1996;334:1642.
34. Knighton D.R., Silver I.A., Hunt T.K. Regulation of wound-healing angiogenesis—effect of oxygen gradients and inspired oxygen concentration. Surgery. 1981;90:262.
35. Knighton D.R., Halliday B., Hunt T.K. Oxygen as an antibiotic: the effect of inspired oxygen on infection. Arch Surg. 1984;119:199.
36. Hunt T.K. The physiology of wound healing. Ann Emerg Med. 1988;17:1265.
37. Roth R.N., Weiss L.D. Hyperbaric oxygen and wound healing. Clin Dermatol. 1994;12:141.
38. Leach R.M., Rees P.J., Wilmhurst P. Hyperbaric oxygen therapy. Br Med J. 1998;317:1140.
39. Undersea and Hyperbaric Medical Society (website):. www.uhms.org. Accessed October 2, 2009
40. Grolman R.E., Wilkerson D.K., Taylor J., et al. Transcutaneous oxygen measurements predict a beneficial response to hyperbaric oxygen therapy in patients with nonhealing wounds and critical limb ischemia. Am Surg. 2001;67:1072.
41. Fife C.E., Buyukcakir C., Otto G.H., et al. The predictive value of transcutaneous oxygen tension measurement in diabetic lower extremity ulcers treated with hyperbaric oxygen therapy: a retrospective analysis of 1,144 patients. Wound Repair Regen. 2002;10:198.
42. Niinikoski J. Hyperbaric oxygen therapy of diabetic foot ulcers, transcutaneous oxymetry in clinical decision making. Wound Repair Regen. 2003;11:458.
43. Niinikoski J.H. Clinical hyperbaric oxygen therapy, wound perfusion, and transcutaneous oximetry. World J Surg. 2004;28:307.