Chapter 1 Visual System
The Eye
Anatomic Features
The eye is a special sense organ made up of three coats, or tunics, as follows:
Within this globe are three spaces: the anterior chamber, posterior chamber, and vitreous chamber. The crystalline lens is located in the region of the posterior chamber (Figure 1-1).
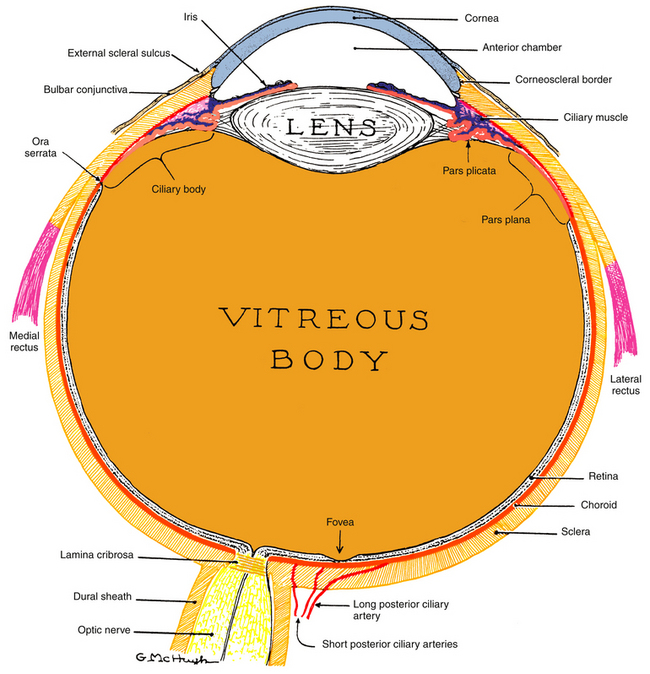
Figure 1-1 The visual system.
(From Kronfeld PC: The human eye, Rochester, NY, 1943, Bausch & Lomb Press.)
The interior of the eye is made up of three chambers. The anterior chamber is bounded in front by the cornea and posteriorly by the iris and anterior surface of the lens. The posterior chamber lies behind the iris and surrounds the equator of the lens, separating it from the ciliary body. The anterior and posterior chambers are continuous with one another through the pupil, and both contain aqueous humor that is produced by the ciliary body. The aqueous humor provides nourishment for the surrounding structures, particularly the cornea and lens. The vitreous chamber, which is the largest space, lies adjacent to the inner retinal layer and is bounded in front by the lens. This chamber contains a gel-like substance, the vitreous humor.
Anatomic Directions and Planes
Anatomy is an exacting science, and specific terminology is basic to its discussion. The following anatomic directions should be familiar (Figure 1-2):
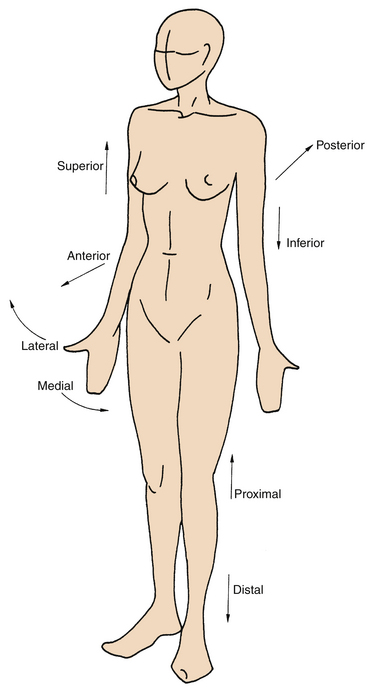
Figure 1-2 Anatomic directions.
(From Palastanga N, Field D, Soames R: Anatomy and human movement, Oxford, UK, 1989, Butterworth-Heinemann.)
The following planes are used in describing anatomic structures (Figure 1-3):
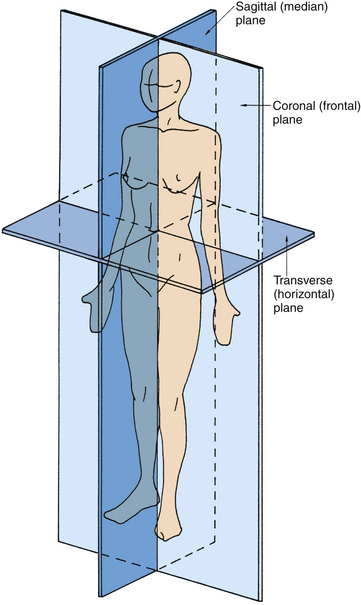
Figure 1-3 Anatomic planes.
(From Palastanga N, Field D, Soames R: Anatomy and human movement, Oxford, UK, 1989, Butterworth-Heinemann.)
Because the globe is a spherical structure, references to locations can sometimes be confusing. In references to anterior and posterior locations of the globe, the anterior pole (i.e., center of the cornea) is the reference point. For example, the pupil is anterior to the ciliary body (see Figure 1-1). When layers or structures are referred to as inner or outer, the reference is to the entire globe unless specified otherwise. The point of reference is the center of the globe, which would lie within the vitreous. For example, the retina is inner to the sclera (see Figure 1-1). In addition, the term sclerad is used to mean “toward the sclera,” and vitread is used to mean “toward the vitreous.”
Refractive Conditions
If the refractive power of the optical components of the eye, primarily the cornea and lens, correlate with the distances between the cornea, lens, and retina so that incoming parallel light rays come into focus on the retina, a clear image will be seen. This condition is called emmetropia (Figure 1-4, A). No correction is necessary for clear distance vision. In hyperopia (farsightedness), the distance from the cornea to the retina is too short for the refractive power of the cornea and lens, thereby causing images that would come into focus behind the retina (Figure 1-4, B). Hyperopia can be corrected by placing a convex lens in front of the eye to increase the convergence of the incoming light rays. In myopia (nearsightedness), because the lens and cornea are too strong or, more likely, the eyeball is too long, parallel light rays are brought into focus in front of the retina (Figure 1-4, C). Myopia can be corrected by placing a concave lens in front of the eye, causing the incoming light rays to diverge.