CHAPTER 42 Ventricular Septal Defect
Ventricular septal defects (VSDs) are among the most common congenital cardiac disorders seen at birth, but are less frequently seen as isolated lesions in adulthood. The reason for this is that a VSD in an infant is usually either large and nonrestrictive, permitting equalization of pressures between the ventricles and leading to heart failure necessitating early surgical repair, or small and restrictive, closing spontaneously without intervention. A VSD can occur as an isolated lesion or in combination with other congenital cardiac anomalies. Blood flow across the defect is typically left to right, and depends on the size of the defect and the pulmonary vascular resistance.1
DEFINITION
Type IV defects also account for 10% of all VSDs, and are called muscular defects. They may be single, but are commonly multiple. Most commonly, multiple defects occur in the apical trabecular septum.1–5
PREVALENCE AND EPIDEMIOLOGY
In the United States, VSD accounts for approximately 20% to 40% of all congenital cardiac malformations—approximately 1 to 2 cases per 1000 live births. Studies have shown that the prevalence of VSD has increased in the United States during the past 30 years. A twofold increase in the prevalence of VSD was reported by the U.S. Centers for Disease Control and Prevention from 1968 to 1980. This increase is primarily attributed to more sensitive detection through echocardiography.6,7
ETIOLOGY AND PATHOPHYSIOLOGY
Alcohol and illicit drug use have been identified as possible risk factors for VSD. There is a twofold increase in the risk of VSD associated with maternal cocaine use during pregnancy. Abnormal blood flow to the heart owing to the vasoconstricting effects of cocaine is a postulated reason for these increases.5,6
VSDs result from a lack of growth or a failure of alignment or fusion of the portions of the ventricular septum. Between weeks 4 and 8 of gestation, the single ventricular chamber is effectively divided into two. This division is accomplished with the fusion of the membranous portion of the ventricular septum, the endocardial cushions, and the bulbous cordis. The muscular portion of the ventricular septum grows cephalad as each ventricular chamber enlarges, eventually meeting with the right and left ridges of the bulbous cordis. The ridges fuse with the tricuspid and mitral valves and the endocardial cushions. The tricuspid valve is separated from the pulmonary valve by the infundibulum, but the mitral valve remains in fibrous continuity with the aortic valve. The endocardial cushions develop concomitantly and finally fuse with the bulbar ridges and the muscular portion of the septum. The fibrous tissue of the membranous portion of the interventricular septum makes the final closure and separates the two ventricles.1–5
The VSD permits a left-to-right shunt to occur at the ventricular level, causing increased pulmonary blood flow, right ventricular volume overload, and compromise of systemic cardiac output. These effects depend on the magnitude of the shunt, which is a function of the size of the VSD and the status of the pulmonary vascular bed. A small VSD with high resistance to flow permits only a small left-to-right shunt. A large interventricular communication allows a large left-to-right shunt. Measuring the ratio of pulmonary-to-systemic circulation (Qp/Qs ratio) is useful for determining the magnitude of the shunt. Over time, as pulmonary vascular resistance increases, right ventricular function is compromised. Eventually, irreversible damage may occur within the pulmonary vascular bed, leading to a reversal of the shunt and the development of increasing cyanosis (Eisenmenger syndrome).1–5
MANIFESTATIONS OF DISEASE
Clinical Presentation
The clinical significance of VSD depends on its size and location, and the level of pulmonary and left ventricular outflow resistance. A restrictive VSD produces a small shunt and does not cause significant hemodynamic derangement. In contrast, a large VSD may progressively lead to higher pulmonary resistance and, finally, to irreversible pulmonary vascular changes, producing Eisenmenger physiology with reversal of the shunt to a right-to-left shunt.1,2
A VSD typically produces a harsh grade IV-VI holosystolic murmur. The murmur is best heard along the left sternal border, is usually louder at the third and fourth intercostal spaces, and is widely transmitted over the precordium with the production of a palpable thrill. The murmur of VSD does not radiate to the left axilla, as with mitral regurgitation, and does not increase in intensity with inspiration, as with tricuspid regurgitation. The smaller and more restrictive the VSD, the more turbulent the flow through the defect, and the louder the murmur. Large defects, with appreciable left-to-right shunts, have wide splitting of S2, which varies with respiration. If pulmonary hypertension develops, the holosystolic murmur diminishes, and the thrill disappears. Cyanosis may become evident, and polycythemia follows. A pulmonary ejection sound may also be noted.1–4
Of patients with congenital VSD, 20% have additional cardiac abnormalities. Most abnormalities are detected at the initial assessment stage; however, aortic valve leaflet prolapse and pulmonary stenosis may also develop subsequently. Aortic regurgitation may result from the high-velocity flow beneath a poorly supported right aortic cusp near the VSD.1
Imaging Technique and Findings
A small VSD usually manifests with no radiographic abnormality. With larger defects, chamber enlargement and pulmonary vascular changes are present, depending on the defect size and volume of the shunt. The typical plain film appearance of a patient with VSD is shunt vascularity associated with right and left ventricular and left atrial enlargement (Fig. 42-1). In young children, and especially in children with larger shunts, the plain film appearance is less specific. There is cardiac enlargement, but interstitial edema is superimposed on shunt vascularity, often giving the appearance of heart failure (Fig. 42-2). With increasing pulmonary resistance, right ventricular and pulmonary artery pressure increase, changing the appearance of the plain film to that of pulmonary hypertension (Fig. 42-3).1
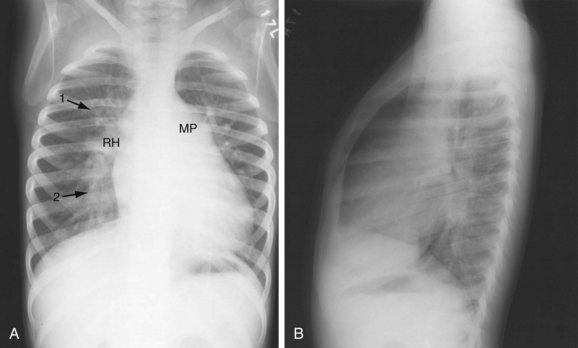
FIGURE 42-2 Chest examination in a 6-year-old boy with a membranous VSD. A, Posteroanterior examination showing typical signs of shunt vascularity and cardiac enlargement. The main pulmonary artery (MP) and right hilar (RH) and upper lobe (1 arrow) and lower lobe (2 arrow) pulmonary arteries are enlarged. The left pulmonary artery lies posterior to the leftward rotated heart. The pulmonary arterial segments, although dilated, are unsharp (compare with the vessels in Fig. 42-1), typical of shunt vessels in young children. Right heart enlargement is indicated by the leftward displacement of the heart from the midline. B, Lateral examination shows filling of the retrosternal clear space (indicating right heart and pulmonary artery enlargement), and filling of the superior and inferior retrocardiac space, indicating left atrial and left ventricular enlargement.
Ultrasonography
Echocardiographic evaluation of VSD is a noninvasive modality that accurately delineates the morphology and associated defects (Figs. 42-4 through 42-6). Hemodynamic evaluation of the defect and the presence of elevated pulmonary artery pressure, insufficiency of the aortic valve, and distortion of the valve apparatus all are accurately evaluated by echocardiography. When limitations in image quality of TTE prevent adequate evaluation, TEE can be performed.8–10