Ventilation-Perfusion Relationships
After reading this chapter, you will be able to:
• Explain why alveolar ventilation () and pulmonary capillary blood flow (
) determine alveolar oxygen pressure (PAO2)
• Use the oxygen–carbon dioxide diagram to characterize absolute shunt and absolute dead space
• Explain why there is an alveolar-to-arterial oxygen pressure difference (P(A-a)O2) in the normal lung
• Show why P(A-a)O2 increases as fractional concentration of inspired oxygen (FIO2) increases
• Explain why high lung regions can compensate for the hypercapnia but not the hypoxemia of low
regions
• Differentiate between general hypoventilation, shunt, and mismatch as causes of hypoxemia
• Explain why absolute shunt is not responsive to oxygen therapy
• Describe the major pathological defects involved in shunt-producing and dead space–producing diseases
• Differentiate between the effects absolute shunt and absolute dead space have on arterial blood gases
• Identify which of the following shunt indicators is the most clinically accurate and reliable: P(A-a)O2, PaO2/FIO2 ratio, or PaO2/PAO2 ratio
• Use the classic physiological shunt equation to calculate the fraction of shunted cardiac output
• Explain why changes in cardiac output affect PaO2 in a patient with abnormally high intrapulmonary shunt
Overall V ˙ A / Q ˙ C
Ratio
Normal gas exchange between air and blood can occur only if pulmonary blood flow perfuses ventilated alveoli. Thus the ratio is critical in maintaining normal gas exchange. The
ratio may refer to ventilation and blood flow in a single alveolus, all alveoli in a single lobe, or all 300 million plus alveoli in the lung. The average resting
(alveolar ventilation) for the lung is about 4 L per minute, and the resting
(pulmonary capillary blood flow) is about 5 L per minute. The overall average
is about 0.8 for the lung as a whole.1 However, the overall
ratio reveals little about the lung’s ability to function as a gas-exchange organ. For example, consider the hypothetical extreme situation in which 5 L per minute of pulmonary blood perfuses a nonventilated left lung and 4 L per minute of gas ventilates a nonperfused right lung. The overall average
would be normal (4 L/min ÷ 5 L/min = 0.8), but gas exchange would be impossible.
V ˙ A / Q ˙ C
Ratio as a Determinant Of Alveolar PO2
The model of the lung and its pulmonary blood flow in Figure 12-1 shows that ventilation continually adds atmospheric oxygen to the alveoli, whereas pulmonary blood flow continually removes oxygen from the alveoli. If blood flow ceases and ventilation continues, oxygen builds up in the alveoli until alveolar PO2 equals the inspired PO2. If ventilation ceases and blood flow continues, PAO2 decreases until it is equal to the PO2 of venous blood. Thus, the balance between the addition of oxygen () and its removal (
) determines PAO2. Decreased
relative to
decreases the
ratio and reduces PAO2 (blood removes oxygen more rapidly than it is replenished). Decreased
relative to
increases the
ratio and increases PAO2 (ventilation adds oxygen more rapidly than it is removed). In the same way, the
ratio also determines PACO2. In this instance, blood flow adds carbon dioxide to alveoli, whereas ventilation removes it.
Alveolar Oxygen–Carbon Dioxide Diagram
Figure 12-2 is a theoretical model of three alveoli with three different relationships. The inspired PO2 and PCO2 for all alveoli are 150 mm Hg and 0 mm Hg (breathing room air at sea level). The middle alveolus has a normal
ratio, maintaining a PAO2 equal to 100 mm Hg and a PACO2 equal to 40 mm Hg. Incoming mixed venous blood with a PO2 of 40 mm Hg and a PCO2 of 45 mm Hg takes on alveolar PO2 and PCO2 values as diffusion and equilibrium occur between the blood and alveolar gas pressures.
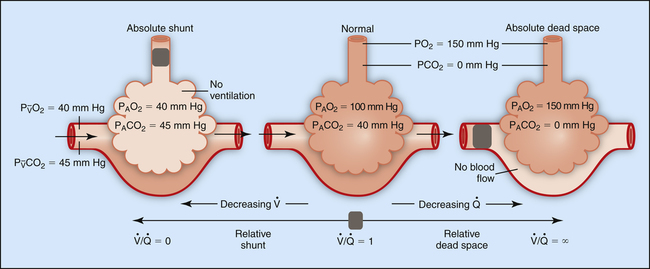
If ventilation gradually decreases with no change in blood flow, the ratio decreases until it eventually equals 0 when ventilation ceases (left alveolus, Figure 12-2). This condition is called absolute shunt. Because no ventilation exists, PAO2 and PACO2 levels eventually equalize with the levels of mixed venous blood (i.e., PAO2 becomes 40 mm Hg, and PACO2 becomes 45 mm Hg). This condition is called shunt because blood leaving unventilated alveoli is identical in composition to the mixed venous blood entering the alveoli, as though blood flow took a detour around the lung, bypassing it altogether. (In reality, a completely blocked alveolus would collapse because blood flow would continually absorb oxygen and shrink the alveolus until it collapsed.)
The alveolus on the right (see Figure 12-2) shows the other extreme. Blood flow is completely blocked, but ventilation persists unchanged. Because no carbon dioxide can enter the alveolus and no oxygen can be taken up by the blood, PACO2 and PAO2 take on values identical to room air. This alveolus has exactly the same PO2 and PCO2 as the conducting airways (i.e., the anatomical dead space). This alveolus represents absolute dead space; the equals ∞ (infinity) because blood flow is zero. The horizontal double arrow below the three alveolar units represents a
continuum of all possibilities ranging from absolute shunt to absolute dead space (from
= 0 to
= 8).
Figure 12-3 illustrates the positions of the three hypothetical alveoli in Figure 12-2 on the oxygen–carbon dioxide diagram.1 PACO2 is plotted on the vertical (y) axis, and PAO2 is plotted on the horizontal (x) axis. Gas pressures in the normal alveolus ( = 1) are represented by a point on the oxygen–carbon dioxide diagram where PAO2 and PACO2 are 100 mm Hg and 40 mm Hg. This point also indicates PO2 and PCO2 values for end-capillary blood leaving the normal alveolus because capillary blood equilibrated with alveolar gas. The absolute shunt alveolus (
= 0) takes on PAO2 values identical to values of mixed venous blood (note the mixed venous symbol,
). Gas pressures in this alveolus are plotted on the oxygen–carbon dioxide diagram showing that PO2 equals 40 mm Hg and PCO2 equals 45 mm Hg. The absolute dead space alveolus (
∞) takes on a PAO2 equal to 150 mm Hg and a PACO2 equal to 0 mm Hg, values identical to inspired atmospheric gas (note the inspired symbol, I, on the x axis). The curved line passing through these three points is the
line. It represents all possible gas compositions in a single alveolus (breathing room air) supplied by mixed venous blood with a PO2 equal to 40 mm Hg and a PCO2 equal to 45 mm Hg. Because end-capillary blood leaving the alveoli equilibrated with alveolar gas, the
line also represents all possible PO2 and PCO2 combinations of end-capillary blood from a single alveolus.
Figure 12-3 shows that a PAO2 of 50 mm Hg and PACO2 of 80 mm Hg cannot coexist in a single alveolus while the patient is breathing room air because this point does not lie on the line. However, this alveolar gas composition can exist if the mixed venous blood composition changes greatly, creating a new
line passing through different points. For example, a greatly increased metabolic rate would produce more carbon dioxide, increasing P
CO2 and decreasing P
O2, especially if cardiac output cannot increase appropriately. A new
line would be created, beginning at much higher carbon dioxide and lower oxygen values on the left end (see Figure 12-3) but curving down to the same inspired point (PCO2 = 0 mm Hg and PO2 = 150 mm Hg) on the right end. The gas composition of any single alveolus and thus of its end-capillary blood depends on the PO2 and PCO2 of its mixed venous blood and its
ratio.
Reciprocal Relationship between Alveolar Carbon Dioxide Pressure and Alveolar Oxygen Pressure Breathing Room Air
The line in Figure 12-3 is drawn as though the mixed venous blood gas composition does not change as
changes. Although this statement is essentially true if the
of only one alveolus changes, it is not true if the collective
of all alveoli uniformly change. Chapter 4 explains that
and PACO2 are reciprocally related. For example, if
decreases to half normal, PACO2 increases from 40 mm Hg to 80 mm Hg. The corresponding PAO2 while breathing room air can be calculated with the alveolar air equation (see Chapter 7), yielding the following result:
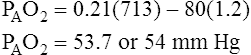
This reciprocal relationship is not shown on the oxygen–carbon dioxide diagram in Figure 12-3 because this diagram represents a hypothetical change in the of one alveolus out of millions of alveoli; a
change in one alveolus would not affect mixed venous blood composition. (The PAO2 of 54 mm Hg and PACO2 of 80 mm Hg in the example do not define a point on the
curve.) In reality, mixed venous blood gases do change when the entire lung hypoventilates. For example, if all 300 million alveoli uniformly hypoventilate, the average PACO2 increases, producing an equal increase in the average end-capillary PCO2. As a result, arterial PCO2 increases. When this arterial blood with an increased PCO2 passes through tissue capillaries, tissue PCO2 builds up until a diffusion gradient from tissues to blood is created; this increases mixed venous PCO2. By increasing PACO2, overall hypoventilation ultimately also increases the mixed venous PCO2. The end result is a new PACO2-PAO2 combination, identifying a point on a new
line in the oxygen–carbon dioxide diagram. The important point illustrated by Figure 12-3 is that the gas composition of any alveolus is determined by its incoming venous gas composition and
ratio.
V ˙ A / Q ˙ C
RATIO Distribution in Normal Lung
Chapter 6 describes why the ratio increases from the lung base to the lung apex (see Figure 6-11). Similar to blood flow, ventilation is greatest in the lung base and least in the apex. However, from the base to apex, blood flow decreases at a more rapid rate than ventilation, which means that apical regions are relatively overventilated with respect to blood flow (high
), whereas basal regions are relatively underventilated with respect to blood flow (low
). Figure 12-4 illustrates these regional differences in
with respect to the oxygen–carbon dioxide diagram. (In this figure, PAO2 decreases by 40 mm Hg from the lung apex to the lung base, whereas PACO2 increases only about 15 mm Hg from apex to base.2)
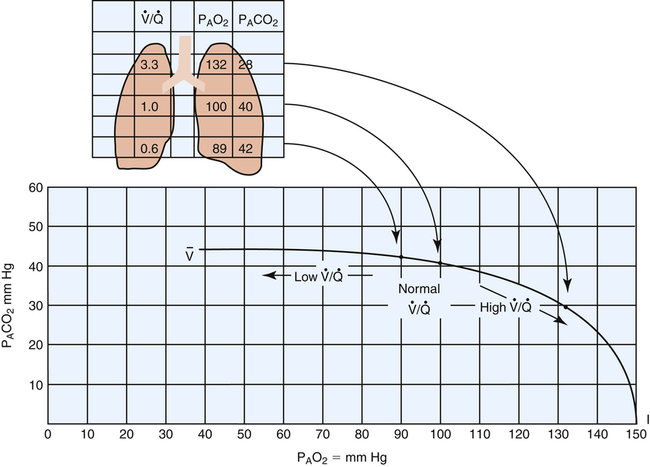

Effect Of V ˙ A / Q ˙ C
Imbalances on Gas Exchange
Normal Gas Exchange
Figure 12-4 shows that the normal lung is not a perfect gas-exchange organ. End-capillary blood from low basal regions has a decreased PO2 and submaximal oxygen content. End-capillary blood from normal
regions has near-maximal oxygen content (PO2 = 100 mm Hg; hemoglobin saturation = 98.5%). Blood from high
apical regions carries only slightly more oxygen per 100 mL than blood from normal units because blood from normal
regions already has near-maximal oxygen content.
Normal Alveolar-Arterial Oxygen Pressure Difference
To understand how the lung’s normal imbalances contribute to the normal P(A-a)O2, one must understand what determines alveolar and arterial oxygen compositions. (Recall from Chapter 1 that another source of the normal P(A-a)O2 is anatomical shunting, in which bronchial venous blood mixes with freshly oxygenated pulmonary venous blood.) Arterial blood is a mixture of the end-capillary blood from all
regions in the lungs. Alveolar gas, collected at the end of a tidal exhalation, is a mixture of alveolar gases from these same differing
regions. Although alveolar gas is in equilibrium with end-capillary blood, the average PAO2 is not the same as the average end-capillary blood PO2. The oxygen-hemoglobin equilibrium curve illustrates this point (Figure 12-5). For the sake of simplicity, this illustration assumes that equal volumes of end-capillary blood from apical and basal lung regions mix to form arterial blood. (This is not actually true because the lung’s bases receive more blood flow than the apices.) PaO2 is not determined by an average of the mixed end-capillary PO2 values; instead, PaO2 is determined by an average of the mixed end-capillary oxygen contents.
For example, let us assume in Figure 12-5 that 100 mL of end-capillary blood from the lung apex is mixed with 100 mL of end-capillary blood from the lung base. Blood from the apex has a PO2 equal to 130 mm Hg and an oxygen saturation (SaO2) of 100%. Blood from the base has a PO2 of 85 mm Hg and an SaO2 of about 94%. With a hemoglobin concentration of 15 g/dL, the oxygen content in 100 mL of apical blood is as follows:

The oxygen content in 100 mL of basal blood is as follows:

Mixing these two samples produces 200 mL of blood containing 39.6 mL of oxygen, or 19.8 mL of oxygen per 100 mL of blood (19.8 mL/dL), corresponding to about 97% saturation on the oxyhemoglobin equilibrium curve (see Figure 12-5). This mixture produces a PaO2 of about 96 to 97 mm Hg, which is obviously not the average of apical and basal PO2 values. High apical units cannot negate the desaturating effect that low
basal units have on mixed end-capillary blood. The desaturating effects of basal alveolar units are even more pronounced than illustrated in this example because basal regions actually receive more blood flow and contribute proportionally more to the arterial blood.
In contrast, mixed expired alveolar gas PO2 is simply the average of the PO2 values from all of the differing regions in the lungs because no hemoglobin is involved. In the example just discussed, the average PAO2 is (130 + 85)/2 = 108 mm Hg, producing a P(A-a)O2 of 12 mm Hg (PAO2 of 108 mm Hg − PaO2 of 96 mm Hg). The differing
ratios in the lung create a normal P(A-a)O2 of approximately 7 to 14 mm Hg, if room air is breathed (FIO2 = 0.21).3 Severely diseased lungs have many abnormally low
regions; in such instances, the P(A-a)O2 is even higher.
Why Alveolar-Arterial Oxygen Pressure Difference Increases When Fractional Concentration of Oxygen in Inspired Gas Increases
P(A-a)O2 increases from about 10 mm Hg when room air is breathed to greater than 50 mm Hg when 100% oxygen is breathed. The shape of the oxygen-hemoglobin equilibrium curve helps explain this phenomenon. Figure 12-6 shows the oxygen-hemoglobin curve extended to a PO2 of 663 mm Hg, which compresses the sigmoid shape of the curve into the extreme left side of the diagram. At PO2 values between 100 mm Hg and 663 mm Hg, the curve is practically flat because the hemoglobin is essentially saturated to capacity between these two points; the small increase in oxygen content is due to increased dissolved oxygen. The diagram of the lung indicates a small, normal amount of shunt—about 2% to 5% of the cardiac output does not perfuse ventilated alveoli. This shunted blood is venous in composition (PO2 = 40 mm Hg; saturation = 75%; oxygen content = 15 mL/dL). Figure 12-6