Chapter 14 Vascular Malformations (Arteriovenous Malformations and Dural Arteriovenous Fistulas)
• Arteriovenous malformations (AVMs) are congenital, high-flow, high-pressure lesions with the primary risk of devastating intracerebral hemorrhage.
• Important characteristics of any AVM include nidus size and location, number and locations of arterial feeders, and pattern of venous drainage.
• Only complete removal of the arteriovenous shunt has been shown to definitively reduce the risk of bleeding.
• Treatment modalities include surgical resection, radiosurgery, endovascular occlusion, or some combination of these approaches.
• Dural arteriovenous fistulas are a subset of AVMs that are thought to be acquired rather than congenital. Treatment focuses on the control of cortical venous drainage to reduce hemorrhage risk.
Classification and Definitions
First, a vascular malformation of the central nervous system is believed to be a dysplastic process, which should be distinguished from a neoplastic process (i.e., a hemangioma is defined as a neoplasm derived from the endothelial cells of a blood vessel). Some literature will, for example, refer to a cavernous malformation as a “cavernoma” or “cavernous angioma,” with the suffix “oma” implying a neoplastic process. In a similar manner, one may also encounter the name “venous angioma” used to describe a developmental venous anomaly. In the category of dysplastic vascular malformations, there have traditionally been four pathological entities:1–3 developmental venous anomalies, capillary telangiectasias, cavernous malformations, and AVMs. In addition, mixed malformations have been found, though they are thought to be rare.4 What separates an AVM from the first three is the presence of an arteriovenous shunt, which is defined as oxygenated blood passing directly into the venous system without gas exchange occurring in a capillary bed. These shunts are characterized by high flow and high pressure, which distinguish them from other vascular malformations.
AVMs may be plexiform, fistulous, or both. A fistulous malformation may be an otherwise normal artery directly joining a vein. A plexiform malformation is defined as an artery connecting to a network of poorly differentiated, immature vessels before passing into a vein. This plexiform network can be termed a nidus (Latin term for “nest”). Figure 14.1 shows a plexiform nidus on the cortical surface. AVMs with plexiform and fistulous components can be seen in any intracranial structure. Because of their developmental nature, they can span multiple structures that are otherwise anatomically distinct. Because they can occur anywhere in the brain, the term brain AVM (BAVM) is coming into wider use and becoming more accepted.5 However, one may encounter multiple adjectives that attempt to describe the malformation (true, cerebral, pial, parenchymal, cisternal, ventricular, medullary) but do not necessarily imply a separate type of pathological entity. However, there is one exception: Dural AVFs (dAVFs) are a distinct pathological type in that their development is not thought to be strictly congenital but acquired. The dAVF will be treated as a separate pathological entity and will be discussed later.
Diagnostic Radiology
Cross-Sectional Imaging
A noncontrast head computed tomography (CT) scan is useful in the acute determination of intracranial hemorrhage. Because this is usually the first study acquired in patients with AVMs, it is important to recognize key features that could arouse suspicion of an AVM. First is that 25% to 30% of AVMs have calcium deposition6 that may be apparent even in the presence of a mass-displacing hemorrhage. In addition, there may be evidence of iso- to hyperdense serpiginous vessels that might be located at some distance from the hemorrhage. Noncontrast CT has no role in ruling out an AVM because many cannot be visualized. A CT angiogram (CTA) may be helpful in an unruptured AVM to help delineate the nidus and associated vessels.
A weakness common to both CT and MR modalities is the lack of temporal sequencing of images to determine the dynamic aspect of the malformation. A good example of this for the MRA (or CTA) is that when all vessels are uniformly enhanced in any image one cannot distinguish between a nidus feeding artery or a draining vein without supplemental anatomical information which may be distorted in a malformation.
Angiography
No cerebral arteriovenous shunt can be completely understood without the aid of a selective cerebral angiogram. In fact, the history of the understanding of this pathology was dependent on the technological developments in the field of radiology. The modern equivalent is the digital subtraction angiogram (DSA), which can subtract out static components of the image (i.e., the skull) to allow the viewer a better visualization of the dynamic components. The main weakness of the angiography when compared to cross-sectional imaging is the lack of good geometric characterization and localization of the nidus. This is more evident as the size of the malformations increases. However, the primary utility of DSA is to establish the diagnosis of an arteriovenous shunt by locating early opacification of the nidus or draining veins in the routine arterial phase of the angiogram (Table 14.1).7 The characterization of this shunting helps to determine not only the pathophysiology of a particular case but also provides a common communication platform upon which various treatment modalities can be discussed between specialists.
Radiological Findings
A judicious use of diagnostic imaging studies is warranted with these complex lesions. A Joint Writing Group publication, in 2001, was a multidisciplinary effort to provide guidelines in the standardized reporting of AVMs for the purposes of clinical research.5 A summary of the important features is provided here. However, note that when AVMs are described, certain characteristics may have more clinical relevance.
Nidus
The size of an AVM usually refers to that of the nidus itself, though the presence of adjacent dilated veins may be confounding. Generally, cross-sectional imaging will provide a more accurate assessment using the same measurement techniques as those for any intracranial mass lesion. Location of the nidus is a second characteristic to note. One is particularly concerned about its proximity to eloquent brain structures.8 Both size and location, though better characterized on cross-sectional imaging, can be roughly estimated as well on angiography, as shown in Figure 14.2A. Additionally, one should characterize the shape for surgical or radiosurgical planning. Unusual geometries may be obscured in angiograms. In locating the nidus, the first step in microsurgical treatment would be consideration of the needed approach for any mass lesion in that location and the potential morbidity.
Arterial Supply
Arteries supplying the AVM should be noted for number, size, relative contribution to the nidus, and location. They can be characterized first by noting what vascular territory they arise from. Angiography from each intracranial vessel should be performed. The detailed description of the intracerebral anatomical vasculature is beyond the scope of this chapter. However, when describing the source of a feeding vessel it would be best to describe its course from the circle of Willis. For large AVMs, when there is concern for meningeal involvement, angiograms of the external carotid arteries should be done as well. Contribution from deep perforating arteries should be clearly noted as they are particularly difficult to deal with in surgery. Finally, one should note that large AVMs or even smaller ones in the temporal or occipital lobe may lie in the vascular border zone between the anterior and posterior circulations; they could, therefore, have vascular pedicles from both. In the era before digitized images, one could simply overlay the transparent films from multiple angiographic injections to get an idea of the complete nidus along with relative locations of erratic feeders. Modern, electronic PACS (picture archiving and communication system) software does not yet seem to have a simple equivalent (Fig. 14.2B).
There are three types of arterial feeders. Direct feeders are the simplest to conceptualize: they end directly and exclusively in the nidus, and they are also known as terminal feeders. Transit arteries are normal arteries that appear on angiogram to pass near or even through the nidus while going on to supply normal tissue. These normal arteries can be easily obscured during nidus opacification. At the same time, their distal territory may never get a sufficient supply of contrast to opacify. An example may be the pericallosal artery passing adjacent to a mesial frontal lobe nidus before proceeding posteriorly. The third type of feeding artery is the indirect feeder or artery en passage. This artery combines the previous two in that as it passes near the nidus it can contribute to the shunt before continuing on to supply normal brain. AVMs within the sylvian fissure usually harbor many en passage contributions from the distal middle cerebral arteries. Rotational angiography and three-dimensional reconstruction has now replaced the old-fashioned stereoangiography, which previously provided excellent tracking of the course of feeding arteries and draining veins.
One other point of importance is the supply to AVM coming from pial collaterals. An AVM in the parietal area, for example, may have most of its supply from the middle cerebral artery, but it also gets its contribution from the posterior cerebral branches coursing over the hemisphere essentially supplying the distal territory of middle cerebral artery beyond the AVM (Fig. 14.2C). Even though these vessels are larger than normal, they are not suitable for endovascular occlusion. This fact also needs to be taken into consideration during microsurgery so that the supply to the normal brain is not affected.
Venous Drainage
The draining veins may be of unusual caliber with a tortuous course, ectasias, or stenosis.9 Of particular importance to note is whether the veins drain superficially via the cortical surface or deeply to the vein of Galen. This is usually easy to identify with an angiogram as the first evidence of an arteriovenous shunt is early opacification of the veins by using anatomical recognition of the vein of Galen along with the major cerebral venous sinuses.
Special Tests
Use of adjunctive diagnostic studies is helpful in selective cases. Functional MRI (fMRI) has helped assess the proximity of language and motor function in relation to AVM but has limitations (Fig. 14.3). Magnetic source imaging (MSI) can accurately localize sensory cortex and even visual and motor areas and the information can be overlaid on the MRI. Recently introduced, tractography should aid in assessing the relationship of deep white matter tracts to the AVM. The information derived from these studies is helpful in deciding the treatment and possibly assessing the neurological risk before intervention. Intraoperative functional mapping has limited value because one cannot do partial treatment in AVMs, in contrast to tumors.
Grading Systems
Clinicians have been attempting to classify AVMs with the goal of helping patients understand the risks by comparison to historical outcomes. An ideal classification system would have the flexibility to cover many pathological variants, the simplicity to be used in a bedside fashion, and the utility to prognosticate. The variability and rarity of AVMs, however, have made this goal difficult to achieve in practice. Several classification schemes have been proposed.10,11 Of these, the Spetzler-Martin system (Table 14.2)12 has the most widespread use and should be known by the practicing neurosurgeon. Grades I and II AVM patients generally tolerated resection without morbidity while grade IV and V AVMs had higher risks of postoperative deficits. Retrospective and prospective studies have shown its utility for surgical decision making.12,13 There are several weaknesses of this system, however. First, it lacks the ability to assess risks for interventions other than exclusive microneurosurgery. Second, the studies were carried out by a highly experienced vascular team and may not necessarily be applicable to a general neurosurgeon’s ability. Third, despite its simplicity, interobserver variability can still occur.14 Finally, there is continuing concern that this system may oversimplify many AVMs. A more recent classification scheme attempts to add deep perforator supply and nidus diffuseness parameters to the Spetzler-Martin system15 as predictors of higher surgical morbidity rates. The chief drawback is the difficultly applying the diffuseness parameter based on imaging (Fig. 14.4). These factors, however, have been recognized to make microsurgical resection more challenging.
TABLE 14.2 Spetzler-Martin Scale for Grading Arteriovenous Malformations
Lesion Characteristic | Points Assigned |
---|---|
Size | |
Small: diameter <3 cm | 1 |
Medium: diameter 3-6 cm | 2 |
Large: diameter >6 cm | 3 |
Location | |
Noneloquent site | 0 |
Eloquent site (sensorimotor, language, or visual cortex; hypothalamus or thalamus; internal capsule; brainstem; cerebellar peduncles; or cerebellar nuclei) | 1 |
Pattern of venous drainage | |
Superficial only | 0 |
Any deep | 1 |
∗Total scores range from 0 to 5; high scores are associated with high risk of permanent neurological deficit after surgery.
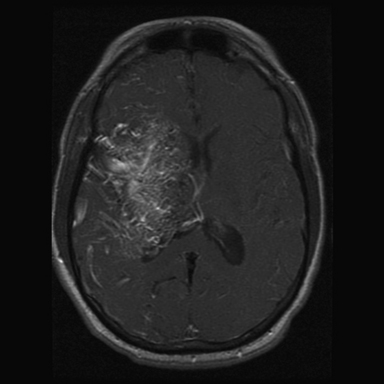
FIGURE 14.4 Axial magnetic resonance imaging (MRI) with gadolinium shows a large left frontal lobe nidus with a high level of diffuseness compared to the one seen in Figure 14.1. This characteristic, though not part of the Spetzler-Martin scale, may increase treatment morbidity.
(From Du R, Keyoung HM, Dowd CF, et al. The effects of diffuseness and deep perforating artery supply on outcomes after microsurgical resection of brain arteriovenous malformations. Neurosurgery 2007;60(4):638-646; discussion 646-648.)
In most of the grading schemes the size measurement is taken as a linear parameter, which when taken in the context of volume, has tremendous variation within the range of dimension. As an example, the spherical volume of a 5.54-cm-diameter AVM is approximately four times greater than an AVM measuring 3.5 cm, even though both of them are assigned 2 points in the Spetzler-Martin scale. Treatment with radiosurgery is volume dependent. In addition, several series have shown that volume is a better predictor of microsurgical risk and outcomes. The approximate volume can be determined by the formula: (length × width × height)/2. These measurements can be obtained from the anteroposterior (AP) and lateral views of the angiogram corrected for magnification.
Pathological Sequelae
Seizures
Seizures are the second most common presenting symptom; they are associated with supratentorial AVMs. Approximately 15% to 30% of all patients with AVMs present with a focal or generalized seizure.16–18 Angiographic characteristics of epileptogenic AVMs include cortical location of the nidus or feeding artery, feeding by the middle cerebral artery, absence of aneurysms, presence of varices in the venous drainage, and association of varix and absence of intranidal aneurysms. Other factors significantly associated with the onset of seizures include AVMs fed by the external carotid artery and a temporal or parietal cortical location.19
An AVM patient presenting with epilepsy has a similar long-term risk profile for hemorrhage when compared to other presentations. That said, some patients may not wish to consider definitive treatment for reasons of age, comorbid conditions, or personal preference. Medical control of seizures with antiepileptic drugs (AEDs) is the first-line treatment. Consultation with neurological epileptologists for multidrug therapy is also indicated. There may, however, be a subset of patients harboring AVMs with medically refractory seizures. Neurological consultation can confirm location of the epileptic focus using clinical semiology or tools such as electroencephalography (EEG), magnetoencephalography (MEG), or single-photon emission computed tomography (SPECT). Given that most AVMs do not harbor functional tissue, the location of this epileptic focus with respect to the actual AVM nidus may help decide the best course of treatment, which may include radiosurgery.20,21
Headaches
Headaches are the presenting symptom in approximately 15% of patients without evidence of rupture.16 They can be characterized as similar to migraines with lateralization to one side, but they may have a more permanent nature. This fact does not necessarily preclude migraines as an independent pathological entity, however, and patients should be informed that the goal of a successful AVM treatment is obliteration of the nidus in order to mitigate risk of debilitating hemorrhage. Occipital AVMs may be a predisposing factor.
Neurological Deficit
A relatively rare presentation for an unruptured AVM is neurological deficit. Deficits may present as transient, progressive, or permanent and the spectrum of deficits varies with the morphological nature of the malformation. Deficits can arise through a number of mechanisms. First, the AVM itself may cause mass effect on adjacent neurovascular structures. Another possibility may be mass effect caused by arterial steal. The arteriovenous shunting may be disruptive to the vascular supply and regulation of surrounding normal brain structures by redirecting flow toward the shunt at a cost to normal vascular beds. This concept, if true, suggests that large AVMs with high flow should correlate with increased physiological evidence of steal. In fact, steal is thought to be relatively rare because the surrounding tissue does manage to adapt.22,23
Development
There has been much speculation about how arteriovenous malformations are thought to develop. Traditionally it has been thought that they are congenital lesions that develop during the embryonic stage. This reasoning stems from histological examination of AVM structures that reveal characteristics that resemble the plexuses of developing vasculature in the embryo. There is also predisposition to develop AVMs in patients with genetic disorders such as Osler-Weber-Rendu and Wyburg-Mason syndromes.24
Against this theory, however, is the fact that AVMs have been known to recur after post-treatment angiographic evidence of obliteration by surgery or radiosurgery, although recurrence is very rare.25 One must keep in consideration that the imaging resolution limit of an angiogram prohibits adequate visualization of the microcirculation. With this in mind, the question becomes whether these postnatal malformations arise de novo or develop into larger structures that can eventually be seen radiographically. In either case, the evidence supports the theory that AVMs are not static lesions but biologically dynamic and that they can develop and remodel over time.
The congenital theory of AVM development suggests that there is a failure of the development of a stable vascular and capillary plexus.26 During embryological development of the nervous system, neuronal growth and migration help shape the vascular network that eventually will supply it. As an example, the population of neurons that develop in the germinal matrix near the ventricle and migrate radially outward toward the cortex can have vessels growing in tandem or, more commonly, migrating from the cortex inward in an antiparallel fashion.
Vascular development is believed to be a concerted mechanism that is a successive combination of three processes: vasculogenesis, angiogenesis, and arteriogenesis (Table 14.3).26,27 Vasculogenesis is thought to occur exclusively in the embryonic period. It is defined as a process of differentiation of vascular progenitor cells into angioblastic cells which eventually form the endothelial layer of all vessels. The vasculogenesis process creates a haphazard network of immature cells that form angiocysts, which eventually fuse to form a primitive capillary plexus. The next process, angiogenesis, involves selective apoptosis along with the migration of supporting vascular smooth muscle cells to form a stable vascular bed. The interplay of these two processes during the embryonic stage requires multiple steps for cell proliferation, migration, differentiation, and programmed destruction. Any misstep during this stage could be the initiating factor in the formation of a congenital vascular malformation.
Molecular Biology and Genetics
The study of the molecular changes with AVMs has revealed the altered expression of many factors that are known to have key roles in the developmental processes noted previously. What is more interesting is that many are shared with cerebral neoplastic processes as well. This suggests that there may be a future role for targeted medical therapies based on the understanding of the abnormal molecular pathways initiated and sustained by these active lesions. Around 900 genes have been shown to have altered expression in AVMs.26,28 Detailing the molecular factors implicated in the embryological development of cerebral vessels is beyond the scope of this chapter. However, a few of those factors that have abnormally high expression in AVMs when compared to normal brain tissue have been noted here.26
The congenital theory of development can imply that an AVM begins as a response to some environmental abnormality during development or that a genetic alteration was initially responsible. In the case of the latter, genetic alterations may be sporadic or familial. Several genetic disease syndromes have been associated with an increased predisposition to harbor AVMs such as Wyburn-Mason syndrome, Sturge-Weber disease, and ataxia-telangiectasia.24,26
Osler-Weber-Rendu disease, also known as hereditary hemorrhagic telangiectasia, is an autosomal dominant disorder with variable penetrance. Genetic analysis has isolated two sources for mutation: HHT1 is found on chromosome 9q and HHT2 is found on chromosome 12q. Incidence for AVM in HHT1 mutations is approximately 10 times higher than that for HHT2, which shows that different genetic mutations alter the risk of developing AVMs based on the function each encoded protein has during development.29 In addition to known hereditary disorders, there have been AVM cases among families, suggesting a multifactorial genetic patterning to formation.
Physiology
The hemodynamics of AVMs is a subject of continuing study. It is difficult to model an AVM using fluid dynamic models given their individual variability. Some electrical network analogies have been described in the literature in order to help conceptualize individual elements within a complex system.30,31 For the scope of this chapter, it may be simpler to reduce the concept into flow rates and pressures through three structures—the feeding artery, the nidus, and the draining vein—of an AVM with one of each. In this model, for conservation of mass, any flow increase to one structure will be the same to all. Flow increase may be achieved, however, through increased feeding arterial pressure, decreased draining pressure, or decrease in the vascular resistance of the AVM by enlargement of the nidus. A nidus can enlarge by increasing the diameter of existing vessels or generating new ones. Note that if a nidus increase can bring about decreased vascular resistance, the feeding artery’s pressure can either drop or its flow rate can increase until a new equilibrium is reached. For pressure to increase in a nidus, flow can be increased by increasing pressure in the feeding artery or flow can be decreased by increasing pressure (indirectly by increasing distal resistance) in the draining vein. This model neglects the fact that many AVMs are not so simple. Also, there is the possibility of capacitance, that is, the ability of the nidus to increase flow without necessarily increasing vascular resistance or, therefore, pressure.
With these concepts in mind, some general hemodynamic considerations in AVM therapies are as follows: (1) Decreasing flow is best effected by obstructing feeding arteries, and (2) decreasing flow by obstructing veins will increase intranidal pressures and, therefore, bleeding risk (Fig. 14.5).
First, as the vascular resistance from the arteriovenous shunt decreases (possibly as a result of nidus enlargement), arterial blood will preferentially flow toward the shunt unless the normal brain tissue can reduce its resistance as well. This situation can progress until the normal brain can no longer match the arteriovenous shunt and becomes underperfused, causing neurological dysfunction. This phenonemenon, known as vascular steal, has been difficult to definitively demonstrate.22 However, there are studies showing that patients with AVMs harbor neuropsychological dysfunction possibly as a result of this phenomenon.32–34
The second idea to develop from the arteriovenous shunting is that as adjacent tissues develop with lower perfusion pressures, they lose their ability to autoregulate their blood flow in response to normal pressure changes. Consequently, when the arteriovenous shunt is removed iatrogenically, overall systemic vascular resistance is increased, allowing for normal physiological perfusion pressures. As these capillary beds are not used to higher pressures, they tend to become hyperemic with increased risk for postresection hemorrhage. This phenomenon is known as normal perfusion pressure breakthrough (NPPB).35
Both of these phenomena have several common points. First, their existence is debated in the literature at the clinical and laboratory level. Second, their pathophysiology is related to the magnitude of arteriovenous shunting and they are therefore more likely to be encountered in larger AVMs. Finally, functional MRI (fMRI) has been used to assist in identifying eloquent brain structures for tumor surgery planning. One should be aware that fMRI utilizes cerebral blood flow changes at the microcirculatory level to determine functional brain activation. Because autoregulation is disrupted in normal brain tissue surrounding AVMs, however, this modality may produce false results (see Fig. 14.3).
Epidemiology and Natural Course
The rarity of AVMs combined with their long period of clinical silence makes it difficult to estimate the overall prevalence of this disease. Two reviews, published in 200036 and 2001,37 highlighted the flaws with previous prevalence estimates along with difficulties in obtaining good estimates. Specifically, older prevalence estimates (500-600 per 100,000 population) were based on autopsy data, a source that is inherently biased. Another estimate (140 per 100,000 population) originated from an inappropriate analysis of data from the Cooperative Study of Intracranial Aneurysms and Subarachnoid Hemorrhage. Determining the true prevalence of AVMs would likely require MRI screening of more than 1 million asymptomatic persons to achieve reasonable confidence intervals. Estimates of incidence have been made by observing the specific geographic populations over time. These separate studies were reviewed37 to find an incidence of approximately 1 per 100,000 person-years. The New York Islands AVM Study, published in 2003, found an average AVM detection rate of 1.34 per 100,000 person-years. The incidence of first ever hemorrhage was 0.51 per 100,000 person-years.38
For any patient presenting with an AVM, the risks of treatment should be weighed against the alternative of no intervention. Though extremely rare, AVMs have been known to spontaneously regress.39,40 A small nidus and single draining vein were common factors. There has been an association between smaller AVMs and increased risk of rupture. This association may be attributable to higher feeding pressures in smaller AVMs.41
There have been numerous attempts to provide better assessments of the lifetime risk for hemorrhage. The oldest and most widely reported study from 1990 utilized prospective data on 160 Finnish patients followed for a mean period of 23.7 years.42 Key characteristics of the patients in this study were that (1) they were the unoperated cohort of a total population of 262 AVM patients, and (2) they were all symptomatic (71% hemorrhage, 23% seizures, 6% other). The study reported an annual hemorrhage rate of 2% to 4% per year with a combined rate of morbidity and mortality to be 2.7% per year and mean time to hemorrhage of 7.7 years. Also, different initial presentation did not apparently change risk of hemorrhage.
In 2008 two studies were published on the Finnish AVM cohort.43,44 The first was a comparison of long-term excess mortality against the general population. It showed that excess mortality rate was highest among patients with no treatment, but also that partially treated AVMs had a comparatively lower excess mortality rate. There was no excess mortality among the completely treated AVM group after 1 year. The second study was a natural history of 238 untreated patients for a mean period of 13.5 years. It showed the annual hemorrhage risk to be 2.4%. Importantly, however, the study showed that there is a higher hemorrhage risk of 4.7% in the first 5 years after diagnosis compared to 1.6% after 5 years. A multivariate analysis showed hemorrhage risk factors to include previous rupture, infratentorial and deep location, and large size.
The cumulative probability of an annualized risk for expected years of remaining life can be determined using the formula p = [1 − (risk of no hemorrhage)n], where n is the number of years of expected life remaining.45 The cumulative probability can also be simply approximated with the linear formula of p = (105 − age)/100 (Fig. 14.6).46
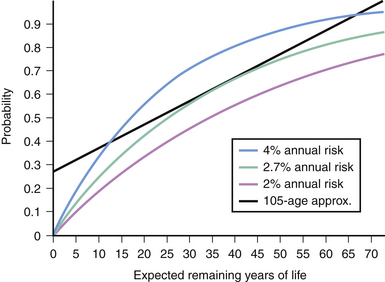
FIGURE 14.6 Applying the law of multiplicative probability, one can construct a risk curve for hemorrhage based on the expected years of life remaining using the various annual risks reported.42 If one assumes a life expectancy of 78 years, the linear formula of “105 − Age” approximates the 2.7% per year risk profile between the ages of 25 to 55 when most arteriovenous malformations present themselves.46
In discussing possible treatment options with asymptomatic patients, there is always the difficulty of performing a potentially morbid procedure for a statistical probability. A study in 2005 showed that patients with AVMs that have hemorrhaged are less likely to worsen clinically after treatment because of the injury already caused by the hemorrhage, but elective AVM surgery is associated with a greater chance of clinical decline from baseline.47 It would be ideal to have an accurate assessment of risk for an otherwise asymptomatic patient, whose AVM may have been detected by imaging modalities that were not in existence when the Finnish Database Study commenced. All studies to date have been biased toward symptomatic patients, the major symptom being hemorrhage. The ARUBA study, which began in 2008,48 was designed to test the null hypothesis that any treatment offers no difference in the risk of death or symptomatic stroke, and no better functional outcome than does conservative management at 5 years from discovery of an unruptured AVM. Although the study is ongoing, the limitation of a 5-year follow-up has been called into question by the Finnish Database Study,44 as evidence of benefits of partial treatment were seen after 5 to 7 years. The average interval between hemorrhages was noted to be 7 years.
Arteriovenous Malformations in the Pediatric Population
In the pediatric population, AVMs are the most likely pathology in spontaneous intracerebral hemorrhage.49,50 As most AVMs are likely to present in the adult years, those that present in the pediatric age group usually have relatively more morbid sequelae that can have devastating consequences. Hemorrhage is the most likely presenting symptom, followed by seizures, congestive heart failure, and neurological deficit. Congestive heart failure is a symptom seen mostly in the newborn population and is secondary to the large amount of left-to-right cardiovascular shunting that occurs from a clinically symptomatic AVM. This is most common in the distinct variety of AVM called the vein of Galen malformation. These newborns tend to have a poor prognosis, although recent advances in critical care support and neuroendovascular techniques have improved long-term outcomes.51
Surgically resected AVMs in the pediatric population have occasionally been observed to recur;52 these patients, therefore, need long-term follow-up. A possible hypothesis involves the earlier mentioned concept of microshunting that is not apparent on angiography but that enlarges over time. This idea is also consistent with the observation that many pediatric AVMs grow in volume and degree of arteriovenous shunting.
Arteriovenous Malformations and Pregnancy
As they are thought to be congenital lesions, AVMs are likely present during a woman’s reproductive years. During pregnancy total blood volume can increase by up to 40% while cardiac output can increase by up to 60% to match the increasing vascular bed demanded by the fetus.53 Arterial pressures can vary during pregnancy; however, hypertension can occur near delivery. Although these hemodynamic alterations during pregnancy have been thought to play a participating role in the occurrence of AVM hemorrhage, it is unclear as to whether there is an increased statistical risk. It is, however, believed that pregnancy does increase the risk of hemorrhage. It generally tends to occur in the second and the third trimesters and the early postpartum period.
If a pregnant patient presents with a hemorrhage from an AVM, the risk of rebleed during the same pregnancy can be up to 27%;54 this risk can be mitigated only with total resection of the AVM. Treating an AVM during pregnancy, however, entails risks to both mother and fetus. Given its risks to the fetus and its slow rate of occlusion, radiosurgery is not a viable option during pregnancy. Endovascular therapies, even as an adjunct, pose the added risks of exposing the fetus to radiation, intravenous (IV) contrast agent, and embolic solvents. Therefore, microsurgical resection may be the only tool if the patient wishes to have treatment before delivery. In general, definitive treatment of the AVM should be deferred until after the pregnancy is over. The patient needs to be followed in a high-risk pregnancy setting and delivered by cesarean section.
If a patient presents with a life-threatening intracranial hemorrhage, immediate surgical evacuation is necessary for stabilization. Otherwise, a careful discussion with the patient and the family should be done regarding the risks of hemorrhage and disability with intervention. Pregnant patients with unruptured AVMs should consider definitive treatment after parturition. These patients may present with seizures that are best controlled medically with anticonvulsants. The patient should be made aware that although these medications may pose a minimal teratogenic risk, especially in the first trimester, they provide the safest option for both mother and child as opposed to immediate surgical treatment.54
Arteriovenous Malformations and Aneurysms
AVMs presenting with aneurysms pose a special challenge because both are vascular pathological lesions with potentially devastating consequences. However, there is a definite relationship between the two.55 The increased flow associated with AVM vessels is thought to predispose them to aneurysm formation because their walls will experience higher shear stress. Generally aneurysms with AVMs can be characterized into four types:56 (1) unrelated to flow vessels (remote); (2) arising at the circle of Willis origin of a vessel supplying the AVM (proximal); (3) arising from the midcourse of a feeding pedicle (pedicular); and (4) arising from within the nidus itself (intranidal). The latter three types can be classified as “flow-related” aneurysms.
The variability in structures of AVMs has made their behavior with aneurysms difficult to predict. Nevertheless, aneurysm rupture is a possible clinical consequence that patients should be aware of. The risk of a ruptured aneurysm should always be kept in mind when evaluating a patient with intracranial hemorrhage. In this setting, a neurosurgeon may not have the luxury of a multidisciplinary team and should, therefore, be prepared. There are some general principles to note. First, a four-vessel cerebral angiogram, CTA, or MRA with adequate visualization of all major arteries should always be attempted to rule aneurysms out. Second, the risk of aneurysm rerupture is higher in the acute setting and the risk of morbidity and death from an aneurysmal subarachnoid hemorrhage is higher compared to an AVM. Third, most aneurysms can be secured using surgery or endovascular coiling, but the resectability of AVMs is not always assured. Fourth, flow-related aneurysms associated with AVMs have been known to regress spontaneously after AVM resection.57 Finally, if an AVM bleeds, there may be morphological changes to the nidus and associated vessels that preclude an accurate characterization in the acute setting. With these principles in mind the important questions to ask during an evaluation for hemorrhage are as follows:
1. Where is the aneurysm (as defined in the preceding paragraph)?
2. Which pathological entity was responsible for the hemorrhage? If there is subarachnoid blood, one may suspect the aneurysm; however, one cannot rule out a nidus on the cortical surface. If there is uncertainty, one should assume the aneurysm has ruptured.
3. Is the AVM sufficiently visualized?
4. If it is well visualized, can it be safely treated surgically?
If the AVM is surgically treatable and the aneurysm can be secured, then it is advisable to treat both in the same setting. If there is any question, however, to the resectability of the AVM, then it is advisable to treat the aneurysm by any means acutely and leave the nidus alone for later, multidisciplinary management (Fig. 14.7).
Treatment
Patients presenting with hemorrhage need treatment based on the degree of hemorrhage and the neurological status. Unlike an aneurysm, the risk of immediate rebleeding is relatively low, but early treatment without advanced understanding of the specific AVM characteristics can be dangerous. Therefore, unless there is a life-threatening hematoma requiring immediate surgical evacuation, treatment of an AVM is delayed to allow time for the hemorrhage to resolve and the AVM to stabilize its architecture for treatment planning. This allows for the brain swelling to improve, so there is better tolerance for surgical manipulation. It also allows for better assessment of the nidus whether looking at microsurgery or radiosurgery as the definitive treatment. If the patient is neurologically deteriorating, urgent intervention is necessary. Immediate treatment of hydrocephalus with a ventriculostomy may be indicated. A CTA can give a good idea about the nidus if it is large enough. If the neurological condition allows, an angiogram is desirable. Preferably one would decompress the hematoma while avoiding the AVM. Attempting to more completely remove the hematoma can result in bleeding from the nidus or premature venous rupture. In case of significant brain swelling, adding a dural patch and leaving a large bone flap out is extremely helpful. It is preferable to wait 4 to 6 weeks before undertaking the definitive treatment. The likelihood of rebleeding, though possible, is extremely rare.
When considering definitive treatment, the following options are available:
Embolization
The advancement of applications in interventional neuroradiology has led to its application in the treatment of AVMs.58,59 Although the treatment of certain AVMs may be possible with interventional techniques alone and the technologies are evolving, embolization is not yet considered an exclusive general treatment for most AVMs for two reasons. First, AVMs that are embolized have a rate of permanent morbidity between 4% and 14%.60 Second, if the goal of AVM cure is considered to be complete obliteration of the nidus with no remaining arteriovenous shunting, then current techniques are not yet at the point where any vessel of any size and location can be safely embolized without risk of perforation or cerebral infarcts. Additionally, attempting to arrest all arteriovenous shunting visualized on angiography can result in eventual reconstitution of shunting by recanalization or de novo vascular development, depending on the embolized vessel’s reaction.
Because embolization is usually done as part of a multimodality approach, it is important that the embolization plan be discussed with the neurosurgeon and radiosurgeon prior to commencing the procedure in order to understand the goals of embolization, which can vary depending on the subsequent treatment. For example, a radiosurgeon may want overall nidus volume reduction as a primary goal in order to bring it within the limits of safe radiation dosing. The tradeoff, however, may be to complicate the overall geometric shape of the nidus beyond the ability of a radiation delivery device, such as the gamma knife. For microsurgery, preoperative embolization can offer several options. In addition to nidus reduction, one may attempt to reduce the overall flow of the arteriovenous shunt by occluding the largest feeders in order to reduce the risk of blood loss during the surgery. Finally, one may attempt to embolize the feeding arteries that may be deemed too difficult to access during surgery.61 Typically these arteries lie deep to the nidus during exposure and may hinder its manipulation. The vessels may vary, depending on which approach the surgeon is considering, so it is difficult to consider general “rules” for the best vessels to embolize. The tradeoff of embolization is that total occlusion may induce normal perfusion pressure breakthrough, especially in large AVMS. Staged embolization over multiple sessions may overcome this problem and can be considered an advantage compared to microneurosurgery because it is easier to perform these minimally invasive procedures multiple times. Another tradeoff to consider is that large amounts of embolic material in the nidus may hinder intraoperative mobilization by making it too stiff. One of the advantages of AVM resections over neoplasms is that they are generally more compressible and, if carefully manipulated, can be moved more easily than a meningioma, for example.
Embolic Agents
Several types of agents have been used in endovascular embolizations of AVMs.62 It is important for neurosurgeons to have a basic idea of their capabilities and limitations in order to effectively communicate with their interventional colleagues in deciding the best possible treatment. Embolic agents generally can be classified into three categories: occlusive devices, microparticles, and liquids.
Occlusive Devices
Occlusive devices include balloons for large vessel occlusions, braided silk threads which are highly thrombogenic, and coils. Fibered coils, in particular, are usually platinum coils with polyester fibers attached to increase thrombogenicity. Coils can be used to permanently occlude larger arterial feeders. They can also be used via a transvenous route in the case of dAVFs to more safely occlude a fistula.62
Particles
Many earlier reports of embolizations used particles, specifically, polyvinyl alcohol (PVA) (Counter PVA particles, Boston Scientific, Fremont, CA). These particles are irregularly shaped and can have slight variations in size such that a selection of 50-µm particles can range from 50 to 100 µm. Selected sizes generally range from 45 to 1180 µm.63 Appropriate particle size selection should be considered in AVMs because of the need to avoid having particles that are too small to lodge within the nidus. A fistulous connection, for example, is generally too large for any particles. In AVMs one can never know the exact sizes of the largest and smallest nidus vessels.
Liquids
Two liquid agents are currently in use in the United States for embolization: NBCA and EVOH (Onyx). The primary difference is in their mechanical properties for delivery. It is advisable to observe the delivery of these agents in the interventional suite to get an idea of their capabilities and weaknesses (Fig. 14.8).
N-Butyl cyanoacrylate (NBCA) (Trufill NBCA Liquid Embolic, Cordis Endovascular) is a monomer stabilized in a hydrophobic ethiodol solution mixed with tantalum for radiopacity; it is also used as a fast-acting skin adhesive (Indermil) and is a chemical variant of superglue. On contact with an ionic solution such as blood, NBCA polymerizes to form a solid cast that strongly adheres to surrounding structures such as the endothelium of a nidus vessel. The rate of polymerization can be manipulated by varying the amount of ethiodol, and this property can be used to plan the amount of penetration. As an example, a fast-flowing fistulous connection may require instant polymerization on exiting the delivery catheter but a large, plexiform nidus may require more time for the liquid to cast the distal portions of the vascularized pedicle. Critical events during the procedure include prevention of polymer flowing past the shunt into the venous circulation, risking pulmonary embolism, as well as rapid removal of the catheter following delivery to avoid refluxing polymer adhesion. Although older than EVOH, NBCA enjoys widespread usage from continued experience along with a microcatheter delivery system that allows for easier manipulation than EVOH in tortuous AVM feeders.63,64
EVOH (ethylene and vinyl alcohol) is also known as Onyx (Micro Therapeutics, Irvine, CA). It is a copolymer that is mixed with tantalum powder and dissolved in DMSO (dimethyl sulfoxide). On injection, the copolymer precipitates as the DMSO diffuses, in a lava-like manner. The outer portion remains nonadhesive but solidifies as the central core continues to flow. This pliable effect can be used in a slower, more controlled manner to selectively embolize the vasculature over a longer period of time. The DMSO solvent can remain in the patient’s system for several days with a distinctive odor. Patients should be reassured that it is safe in therapeutic quantities.63,65
Microsurgery
General Surgical Principles
Securing the individual vessels can be done with bipolar coagulation. However, the pathological nature of the AVM vessel wall may not allow it to be sufficiently coagulated to withstand arterial pressures. At the same time, many vessels may have walls that are too thin to close for coagulation. In many neurosurgical procedures, surgeons often place the bipolar tips in close approximation with the open vessel, usually a vein, in between the vessel wall while the electrical field generated by the tip delivers enough energy into the thin vessel walls to allow for coagulation. It is important to realize that this effect may be mitigated in AVM vessels by high blood flow transferring energy out of the vessel walls, thereby keeping them at a subcoagulation temperature. Therefore, arterial aneurysm clips should always be loaded and ready for use, especially for larger feeding arteries. For smaller vessels, Sundt vascular microclips were designed for this purpose and could be used. Another issue to consider during small vessel coagulation is that excessive bipolar usage or premature disconnection can cause a small vessel to retract into normal parenchymal tissue. Again, although in many other neurosurgical procedures, placing cotton with gentle pressure and patience may arrest this bleeding, the high flow associated with AVMs may not allow this technique to work. In the majority of instances it is feasible to occlude the vessels with bipolar coagulation by keeping the power low and occluding the vessel gradually. In our own experience it has been rarely necessary to use the clips. Occasionally, however, a larger feeder may be occluded with a temporary aneurysm clip while the actual smaller feeding vessels are isolated and occluded.
Surgical Approaches
Even though many of the small, deeper malformations are currently being treated with radiosurgery, they are also treatable microsurgically and actually successfully. For deeper AVMs surgical approaches generally fall into three categories: transcortical, transcortical-transventricular, or interhemispheric (Table 14.4).66,67 The presence of intraventricular hemorrhage from a deep AVM usually requires a transcortical-transventricular or an interhemispheric route. Each approach has advantages and complications. Cortical incisions risk injury to eloquent cortex and epileptogenesis. Interhemispheric routes offer limited, deep exposure of medial structures with risks to bridging veins and complications associated with callosotomy. Transventricular routes can cause bleeding into the CSF circulation, risking hydrocephalus and chronic shunt dependence.
TABLE 14.4 Surgical Approaches to Deep Arteriovenous Malformations (AVMs) with Intraventricular Hemorrhage
Approach | AVM Location |
---|---|
Transcortical-Transventricular Approaches | |
1. Middle frontal | Caudate head; anterolateral thalamus |
2. Parietal | Posterolateral thalamus; trigone |
3. Middle temporal | Trigone |
4. Anterior and inferior temporal | Trigone |
5. Temporoparietal | Trigone |
6. Occipital | Trigone |
Interhemispheric Approaches | |
1. Transcallosal (rostrum) | Caudate head; anteromedial thalamus; anterior third ventricle |
2. Transcallosal (splenium) | Posteromedial thalamus; posterior third ventricle |
3. Retrocallosal | Parasplenial-retrosplenial; medial occipital horn |
Transcortical Approaches to Nonhemorrhagic Deep AVMs | |
1. Middle frontal | Dorsal AVMs (caudate head) |
2. Orbitofrontal | Anterior perforated substance; deep basal ganglia |
3. Transinsular | Putamen; external capsule |
4. Combined middle frontal transsylvian | Dorsal AVMs |
Postoperative Management
Patients should be admitted to the intensive care unit (ICU) for at least 24 hours with continued arterial blood pressure control and neurological checks. Depending on the size of the AVM, its location, the surgical approach, and the patient’s neurological status, continued ICU observation may be indicated. It is generally advisable to undergo an angiogram to confirm total resection of the AVM prior to discharge. If the resection was incomplete, a reopening of the craniotomy can be done during the same admission. Postoperative complications include hemorrhage, seizures, cerebral edema, and postsurgical infarcts especially related to venous occlusions.
Radiosurgery
There are drawbacks to radiosurgery for AVMs, however. First, as the radiation induces a biological effect that is dependent on cellular mitosis, there is a considerable delay of 2 to 5 years between treatment and nidus obliteration. During this time, there is no reduction in the risk of hemorrhage. Applying the 2.7% annual rate42 leaves a 2- to 5-year actuarial risk of 5.3% to 12.7%; note that this risk would be even higher if the AVM has recently ruptured. In a similar manner, the potential morbidities from radiosurgery may develop over time. Complications not related to the AVM include the effects of radiation delivery to surrounding functional tissue. These effects have been studied more in relation to treatment for neoplastic diseases and include postradiation edema, cyst formation, and radionecrosis.
High-dose radiation is believed to induce an inflammatory response in the vascular walls of the nidus with loss of endothelium and proliferation of smooth muscle cells.29 Because the AVM is a biologically dynamic structure, it has the ability to compensate if the radiation dose is insufficient. Radiosurgeons typically prescribe an isodose shell, defined as a set of all points within a plan that receive the same radiation dose. The objective is to match the minimum isodose shell to the periphery of a nidus. As an example, a nidus border that is set to 18 Gy to the 70% isodose line means that the periphery of the nidus will receive 18 Gy while points interior will receive up to 18/0.7 = 25.7 Gy. The dose delivered to the periphery is highly correlated to the rate of obliteration. Typically, an AVM with a small, compact nidus will be easily covered by an isodose conformation. As the volume becomes higher the radiosurgeon must either decrease the radiation delivered to the periphery of the nidus by reducing the isodose shell (risking nonobliteration) or increase the overall amount of radiation (risking increased complications). A nidus volume that is less than 3 mL or a nidus that is smaller than 2 cm in its largest dimension, therefore, is a good predictor of successful AVM obliteration.68–70 Other factors include sharp delineation of the nidus border on imaging, younger age, fewer draining veins, and hemispheric location.71
A conservative definition of radiosurgery treatment failure would be persistent arteriovenous shunting demonstrated on angiography 5 years after treatment. Options for therapy include revisiting multimodality treatment.72,73
Dural Arteriovenous Fistulas
As mentioned earlier, dAVFs (also called dAVMs) are not thought to be congenital, but acquired. The dura is a vascularized structure that has a blood supply that is distinct from the rest of the brain. Most dAVFs occur adjacent to the venous sinuses.74 The dural walls form several sinuses functioning as conduits for cerebral venous drainage. There is the opportunity for arteriovenous shunting between the arterial vessels supplying the dura and the sinuses themselves. A simple conceptualization of the formation of a dural arteriovenous shunt may be the rupture of a small artery due to a trauma that, in other compartmentalized tissues, may tamponade itself. The relatively low pressure of the adjacent venous drainage, however, leads to a high-flow fistula that is unable to spontaneously thrombose. Infection may pose a similar effect. Most fistulas, however, are idiopathic. Adjacent venous sinus thrombosis has been known to be related to dAVFs and has been theorized to be the triggering factor.75 Thrombosis may precipitate dissolution of microvessels within the sinus secondary to venous hypertension or the release of pro-angiogenesis factors. Like brain AVMs, these fistulas are biologically active and thus are able to recruit additional arterial supply (including intracranial arteries) over time. Despite their acquired nature, however, they are rarer than AVMs, usually composing 10% to 15% of all high-flow arteriovenous shunts. Most are seen in the region of the transverse and sigmoid sinuses.76 Other locations include the cavernous, straight, petrosal, and superior sagittal sinuses. Carotid cavernous fistulas (CCFs) have been classified into four types based on the feeding arteries involved.77 The type A variant, involving direct communication between the internal carotid artery and cavernous sinus, can usually be brought about by trauma or a cavernous carotid aneurym. However, types B, C, and D can be considered dAVFs based on the supply from meningeal internal carotid artery branches (B), meningeal external carotid artery branches (C), or both (D).
Like the AVM, the main morbidity risk of dAVF is intracranial hemorrhage from rupture. Unlike an AVM, however, dAVFs do not usually have a well-defined nidus. This difference may make their pathological behavior similar to that of a fistulous brain AVM. Another difference with brain AVMs is that the anatomical presentation influences the risk of rupture. The mechanical strength of the vessel wall of the dural sinuses is much larger than that of an intracranial vein, allowing the sinus to withstand arterial pressures with minimal risk of rupture. The risk of hemorrhage and neurological deficit is increased, however, with cortical vein involvement.74 This has led to classification schemes based more on patterns of venous drainage than size, shape, or location. The Borden and Cognard classification systems (Table 14.5) are commonly used.74,78 Although the Borden scheme is simpler, the advantage of the Cognard scheme is that it uses the dynamics of angiography to incorporate the direction of venous flow in the sinus which may cause venous congestion to cortical veins that are not exhibiting reflux.74,78 Proper classification is important in order to assess the necessity of treatment.
TABLE 14.5 Classification Systems for Dural Arteriovenous Fistulas with Recommended Treatment Strategy
Type | Venous Drainage Pattern | Recommendation |
---|---|---|
Borden Classification | ||
I | Into venous sinus or meningeal vein | Observation |
II | Into venous sinus with cortical venous reflux | Treatment |
III | Into cortical veins only | Treatment |
Cognard Classification | ||
I | Into venous sinus with antegrade flow | Observation |
IIa | Into venous sinus with retrograde flow | Treatment if symptomatic |
IIb | Into venous sinus with antegrade flow and cortical venous reflux | Treatment |
IIa + IIb | Into venous sinus with retrograde flow and cortical venous reflux | Treatment |
III | Into cortical veins only | Treatment |
IV | Into cortical veins only with venous ectasia | Treatment |
V | Into spinal perimedullary vein | Treatment |
It is critical to make the distinction between dAVFs with direct sinus drainage versus associated or direct cortical venous drainage (Fig. 14.9). Treatment decisions, being dependent on making this distinction, require absolutely detailed selective and superselective angiography including the external carotids as well as vertebral arteries. This also allows for the assessment in planning the treatment, if felt necessary.
Treatment concepts center on whether the fistula can be successfully occluded or not. Here, both endovascular and microsurgical techniques can be used to occlude the arterial feeders and the venous drainage. It is tempting to initially plan transarterial obliterations using endovascular and surgical approaches. However, several caveats apply: First, arterial feeders may be too small and numerous to successfully catheterize. Second, not all arterial feeders may be seen on angiogram. In addition, the dAVF is a biologically dynamic pathological process that can recruit new arterial feeders to the fistula and they may not be as accessible, especially if only partial occlusion or simply proximal occlusion of large feeders is accomplished. The liquid embolic agents are providing better means of transarterial embolization. The risk to the cranial nerves needs to be kept in mind because these nerves derive their blood supply from the external carotid branches such as the ascending pharyngeal arteries. Similarly, unusual anastomoses between the external and internal carotid branches (e.g., middle meningeal) require identification to avoid inadvertent passage of embolic material into the internal carotid circulation, risking neurological deficits.
The dAVFs involving the skull base and the tentorium are often extremely complex, in the case of both arterial supply and venous drainage. The arterial supply to the tentorial lesions is typically dual (i.e., from external carotid branches as well as internal carotid). There can also be feeders from branches of the vertebrobasilar circulation. The internal carotid branches usually come from the meningohypophyseal trunk or the meningeal branches of the ophthalmic artery. These branches are usually not amenable to transarterial occlusion and require microsurgical occlusion. The surgical approaches need to be individualized and one can take advantage of different skull base approaches.79 The fistulas affecting the skull base itself have their own special features. The ones involving the bone lateral to the jugular and hypoglossal foramina can be easily eliminated by intra-arterial embolization followed by removal of the affected bone.80–82 On the other hand, when the clivus is involved there is arterial supply from both sides and often very small branches, making complete obliteration literally impossible.
Cognard C., Gobin Y.P., Pierot L., et al. Cerebral dural arteriovenous fistulas: clinical and angiographic correlation with a revised classification of venous drainage. Radiology. 1995;194(3):671-680.
Joint Writing Group. Reporting terminology for brain arteriovenous malformation clinical and radiographic features for use in clinical trials. Stroke. 2001;32(6):1430-1442.
Ondra S.L., Troupp H., George E.D., Schwab K. The natural history of symptomatic arteriovenous malformations of the brain: a 24-year follow-up assessment. J Neurosurg. 1990;73(3):387-391.
Pollock B.E., Flickinger J.C., Lunsford L.D., et al. Factors associated with successful arteriovenous malformation radiosurgery. Neurosurgery. 1998;42(6):1239-1244. discussion 1244-1247
Spetzler R.F., Martin N.A. A proposed grading system for arteriovenous malformations. J Neurosurg. 1986;65(4):476-483.
Please go to expertconsult.com to view the complete list of references.
1. McCormick W.F. The pathology of vascular (“arteriovenous”) malformations. J Neurosurg. 1966;24(4):807-816.
2. McCormick W.F., Nofzinger J.D. “Cryptic” vascular malformations of the central nervous system. J Neurosurg. 1966;24(5):865-875.
3. McCormick W.F., Hardman J.M., Boulter T.R. Vascular malformations (“angiomas”) of the brain, with special reference to those occurring in the posterior fossa. J Neurosurg. 1968;28(3):241-251.
4. Hamm K.D., Klisch J., Surber G., et al. Special aspects of diagnostic imaging for radiosurgery of arteriovenous malformations. Neurosurgery. 2008;62(5):A44.
5. Joint Writing Group. Reporting terminology for brain arteriovenous malformation clinical and radiographic features for use in clinical trials. Stroke. 2001;32(6):1430-1442.
6. Osborn A. Diagnostic Imaging. Salt Lake City: Amirsys; 2004.
7. Yasargil M. Microneurosurgery. Stuttgart: Georg Thieme Verlag; 1984.
8. Ganju A., Malik G. Eloquent cortex localization in arteriovenous malformations. Adv Clin Neurosci. 2000;10:93-106.
9. Patel S., Burke T., Chundi V., et al. Venous restrictive disease in cerebral arteriovenous malformations. Proceedings of the XV Symposium Neuro-radiologicum. Wien, Austria: Springer-Verlag; 1994:. 118-120
10. Luessenhop A.J., Gennarelli T.A. Anatomical grading of supratentorial arteriovenous malformations for determining operability. Neurosurgery. 1977;1(1):30-35.
11. Shi Y.Q., Chen X.C. A proposed scheme for grading intracranial arteriovenous malformations. J Neurosurg. 1986;65(4):484-489.
12. Spetzler R.F., Martin N.A. A proposed grading system for arteriovenous malformations. J Neurosurg. 1986;65(4):476-483.
13. Hamilton M.G., Spetzler R.F. The prospective application of a grading system for arteriovenous malformations. Neurosurgery. 1994;34(1):2.
14. Du R., Dowd C.F., Johnston S.C., et al. Interobserver variability in grading of brain arteriovenous malformations using the Spetzler-Martin system. Neurosurgery. 2005;57(4):668-675. discussion 668-675
15. Du R., Keyoung H.M., Dowd C.F., et al. The effects of diffuseness and deep perforating artery supply on outcomes after microsurgical resection of brain arteriovenous malformations. Neurosurgery. 2007;60(4):638-646. discussion 646-648
16. Brown R.D., Wiebers D.O., Torner J.C., O’Fallon W.M. Frequency of intracranial hemorrhage as a presenting symptom and subtype analysis: a population-based study of intracranial vascular malformations in Olmsted Country, Minnesota. J Neurosurg. 1996;85(1):29-32.
17. Crawford P.M., West C.R., Chadwick D.W., Shaw M.D. Arteriovenous malformations of the brain: natural history in unoperated patients. J Neurol Neurosurg Psychiatr. 1986;49(1):1-10.
18. Mast H., Young W.L., Koennecke H.C., et al. Risk of spontaneous haemorrhage after diagnosis of cerebral arteriovenous malformation. Lancet. 1997;350(9084):1065-1068.
19. Turjman F., Massoud T., Sayre J., et al. Epilepsy associated with cerebral arteriovenous malformations: a multivariate analysis of angioarchitectural characteristics. AJNR Am J Neuroradiol. 1995;16(2):345-350.
20. Kurita H., Kawamoto S., Suzuki I., et al. Control of epilepsy associated with cerebral arteriovenous malformations after radiosurgery. J Neurol Neurosurg Psychiatr. 1998;65(5):648-655.
21. Lim Y.J., Lee C.Y., Koh J.S., et al. Seizure control of gamma knife radiosurgery for non-hemorrhagic arteriovenous malformations. Acta Neurochir Suppl. 2006;99:97-101.
22. Mast H., Mohr J.P., Osipov A., et al. “Steal” is an unestablished mechanism for the clinical presentation of cerebral arteriovenous malformations. Stroke. 1995;26(7):1215-1220.
23. Fink G.R. Effects of cerebral angiomas on perifocal and remote tissue: a multivariate positron emission tomography study. Stroke. 1992;23(8):1099-1105.
24. Stehbens W. Telangiectasias, hemangiomas, arteriovenous malformations, and allied disorders. In: Stehbens W., editor. Pathology of the Cerebral Blood Vessels. St. Louis: Mosby; 1972:471-558.
25. Hashimoto N., Nozaki K. Do cerebral arteriovenous malformations recur after angiographically confirmed total extirpation? Crit Rev Neurosurg. 1999;9(3):141-146.
26. Moftakhar P., Hauptman J.S., Malkasian D., Martin N.A. Cerebral arteriovenous malformations. Part 1: cellular and molecular biology. Neurosurg Focus. 2009;26(5):E10.
27. Heil M., Eitenmuller I., Schmitz-Rixen T., Schaper W. Arteriogenesis versus angiogenesis: similarities and differences. J Cell Mol Med. 2006;10(1):45.
28. Lim M., Cheshier S., Steinberg G.K. New vessel formation in the central nervous system during tumor growth, vascular malformations, and moyamoya. Curr Neurovasc Res. 2006;3(3):237-245.
29. Achrol A.S., Guzman R., Varga M., et al. Pathogenesis and radiobiology of brain arteriovenous malformations: implications for risk stratification in natural history and posttreatment course. Neurosurg Focus. 2009;26(5):E9.
30. Hademenos G.J., Massoud T.F., Viñuela F. A biomathematical model of intracranial arteriovenous malformations based on electrical network analysis: theory and hemodynamics. Neurosurgery. 1996;38(5):1005-1014. discussion 1014-1015
31. Kailasnath P., Chaloupka J.C. Mathematical modeling of AVM physiology using compartmental network analysis: theoretical considerations and preliminary in vivo validation using a previously developed animal model. Neurol Res. 1996;18(4):361-366.
32. Mahalick D.M., Ruff R.M., U HS. Neuropsychological sequelae of arteriovenous malformations. Neurosurgery. 1991;29(3):351-357.
33. Mahalick D.M., Ruff R.M., Heary R.F., U HS. Preoperative versus postoperative neuropsychological sequelae of arteriovenous malformations. Neurosurgery. 1993;33(4):563-570. discussion 570-571
34. Marks M.P., Lane B., Steinberg G., Chang P. Vascular characteristics of intracerebral arteriovenous malformations in patients with clinical steal. AJNR Am J Neuroradiol. 1991;12(3):489-496.
35. Spetzler R.F., Wilson C.B., Weinstein P., et al. Normal perfusion pressure breakthrough theory. Clin Neurosurg. 1978;25:651-672.
36. Berman M.F., Sciacca R.R., Pile-Spellman J., et al. The epidemiology of brain arteriovenous malformations. Neurosurgery. 2000;47(2):389-396. discussion 397
37. Al-Shahi R., Warlow C. A systematic review of the frequency and prognosis of arteriovenous malformations of the brain in adults. Brain. 2001;124(10):1900.
38. Stapf C., Mast H., Sciacca R.R., et al. The New York Islands AVM study: design, study progress, and initial results. Stroke. 2003;34(5):e29.
39. Enam S.A., Malik G.M. Association of cerebral arteriovenous malformations and spontaneous occlusion of major feeding arteries: clinical and therapeutic implications. Neurosurgery. 1999;45(5):1105-1111. discussion 1111-1112
40. Abdulrauf S.I., Malik G.M., Awad I.A. Spontaneous angiographic obliteration of cerebral arteriovenous malformations. Neurosurgery. 1999;44(2):280-287. discussion 287-288
41. Spetzler R.F., Hargraves R.W., McCormick P.W., et al. Relationship of perfusion pressure and size to risk of hemorrhage from arteriovenous malformations. J Neurosurg. 1992;76(6):918-923.
42. Ondra S.L., Troupp H., George E.D., Schwab K. The natural history of symptomatic arteriovenous malformations of the brain: a 24-year follow-up assessment. J Neurosurg. 1990;73(3):387-391.
43. Hernesniemi J.A., Dashti R., Juvela S., et al. Natural history of brain arteriovenous malformations: a long-term follow-up study of risk of hemorrhage in 238 patients. Neurosurgery. 2008;63(5):823-829. discussion 829-831
44. Laakso A., Dashti R., Seppänen J., et al. Long-term excess mortality in 623 patients with brain arteriovenous malformations. Neurosurgery. 2008;63(2):244-253. discussion 253-255
45. Kondziolka D., McLaughlin M.R., Kestle J.R. Simple risk predictions for arteriovenous malformation hemorrhage. Neurosurgery. 1995;37(5):851-855.
46. Fleming K., Brown R.D. Natural history of intracranial vascular malformations. In: Winn H.R., editor. Youmans Neurological Surgery. 5th ed. St. Louis: Elsevier; 2004:2160.
47. Lawton M.T., Du R., Tran M.N., et al. Effect of presenting hemorrhage on outcome after microsurgical resection of brain arteriovenous malformations. Neurosurgery. 2005;56(3):485-493. discussion 485-493
48. Mohr J.P. A randomized trial of unruptured brain arteriovenous malformations (ARUBA). Acta Neurochir Suppl. 2008;103:3-4.
49. Schoenberg B.S., Mellinger J.F., Schoenberg D.G. Cerebrovascular disease in infants and children: a study of incidence, clinical features, and survival. Neurology. 1978;28(8):763-768.
50. Malik G.M., Sadasivan B., Knighton R.S., Ausman J.I. The management of arteriovenous malformations in children. Childs Nerv Syst. 1991;7(1):43-47.
51. Lasjaunias P., Hui F., Zerah M., et al. Cerebral arteriovenous malformations in children. Management of 179 consecutive cases and review of the literature. Childs Nerv Syst. 1995;11(2):66-79. discussion 79
52. Hladky J.P., Lejeune J.P., Blond S., et al. Cerebral arteriovenous malformations in children: report on 62 cases. Childs Nerv Syst. 1994;10(5):328-333.
53. Pedersen H., Finster M. Anesthetic risk in the pregnant surgical patient. Anesthesiology. 1979;51(5):439-451.
54. Sadasivan B., Malik G.M., Lee C., Ausman J.I. Vascular malformations and pregnancy. Surg Neurol. 1990;33(5):305-313.
55. Mckissock W., Paterson J.H. A clinical survey of intracranial angiomas with special reference to their mode of progression and surgical treatment: a report of 110 cases. Brain. 1956;79(2):233-266.
56. Perata H.J., Tomsick T.A., Tew J.M.Jr. Feeding artery pedicle aneurysms: association with parenchymal hemorrhage and arteriovenous malformation in the brain. J Neurosurg. 1994;80(4):631-634.
57. Meisel H.J., Mansmann U., Alvarez H., et al. Cerebral arteriovenous malformations and associated aneurysms: analysis of 305 cases from a series of 662 patients. Neurosurgery. 2000;46(4):793-800. discussion 800-802
58. Wolpert S.M., Stein B.M. Catheter embolization of intracranial arteriovenous malformations as an aid to surgical excision. Neuroradiology. 1975;10(2):73-85.
59. Luessenhop A.J., Rosa L. Cerebral arteriovenous malformations. Indications for and results of surgery, and the role of intravascular techniques. J Neurosurg. 1984;60(1):14-22.
60. Haw C.S., ter Brugge K., Willinsky R., Tomlinson G. Complications of embolization of arteriovenous malformations of the brain. J Neurosurg. 2006;104(2):226-232.
61. Martin N.A., Khanna R., Doberstein C., Bentson J. Therapeutic embolization of arteriovenous malformations: the case for and against. Clin Neurosurg. 2000;46:295-318.
62. Alexander M.J., Tolbert M.E. Targeting cerebral arteriovenous malformations for minimally invasive therapy. Neurosurgery. 2006;59(5):S3.
63. Hurst R. Interventional Neuroradiology. New York: Informa Healthcare; 2008.
64. N-BCA Trial Investigators. N-butyl cyanoacrylate embolization of cerebral arteriovenous malformations: results of a prospective, randomized, multi-center trial. AJNR Am J Neuroradiol. 2002;23(5):748-755.
65. Weber W., Kis B., Siekmann R., et al. Preoperative embolization of intracranial arteriovenous malformations with Onyx. Neurosurgery. 2007;61(2):244-252. discussion 252-254
66. Malik G., Charbel F. Arteriovenous malformations of the basal ganglia, thalamus, and adjacent ventricles. Rengachary S.S., Wilkins R.H., editors. Neurosurgical Operative Atlas. Vol. 4. AANS: Rolling Meadows; 1995:43-58.
67. Malik G.M., Umansky F., Patel S., Ausman J.I. Microsurgical removal of arteriovenous malformations of the basal ganglia. Neurosurgery. 1988;23(2):209-217.
68. Flickinger J.C., Pollock B.E., Kondziolka D., Lunsford L.D. A dose-response analysis of arteriovenous malformation obliteration after radiosurgery. Int J Radiat Oncol Biol Phys. 1996;36(4):873-879.
69. Flickinger J.C., Kondziolka D., Maitz A.H., Dade Lunsford L. An analysis of the dose-response for arteriovenous malformation radiosurgery and other factors affecting obliteration. Radiother Oncol. 2002;63(3):347-354.
70. Friedman W.A., Bova F.J., Mendenhall W.M. Linear accelerator radiosurgery for arteriovenous malformations: the relationship of size to outcome. J Neurosurg. 1995;82(2):180-189.
71. Pollock B.E., Flickinger J.C., Lunsford L.D., et al. Factors associated with successful arteriovenous malformation radiosurgery. Neurosurgery. 1998;42(6):1239-1244. discussion 1244-1247
72. Marks M.P., Lane B., Steinberg G.K., et al. Endovascular treatment of cerebral arteriovenous malformations following radiosurgery. AJNR Am J Neuroradiol. 1993;14(2):297-303. discussion 304-305
73. Steinberg G.K., Chang S.D., Levy R.P., et al. Surgical resection of large incompletely treated intracranial arteriovenous malformations following stereotactic radiosurgery. J Neurosurg. 1996;84(6):920-928.
74. Borden J.A., Wu J.K., Shucart W.A. A proposed classification for spinal and cranial dural arteriovenous fistulous malformations and implications for treatment. J Neurosurg. 1995;82(2):166-179.
75. Mullan S., Johnson D.L. Combined sagittal and lateral sinus dural fistulae occlusion. J Neurosurg. 1995;82(2):159-165.
76. Awad I.A., Little J.R., Akarawi W.P., Ahl J. Intracranial dural arteriovenous malformations: factors predisposing to an aggressive neurological course. J Neurosurg. 1990;72(6):839-850.
77. Barrow D.L., Spector R.H., Braun I.F., et al. Classification and treatment of spontaneous carotid-cavernous sinus fistulas. J Neurosurg. 1985;62(2):248-256.
78. Cognard C., Gobin Y.P., Pierot L., et al. Cerebral dural arteriovenous fistulas: clinical and angiographic correlation with a revised classification of venous drainage. Radiology. 1995;194(3):671-680.
79. Mahmood A., Malik G.M. Dural arteriovenous malformations of the skull base. Neurol Res. 2003;25(8):860-864.
80. Liu J.K., Mahaney K., Barnwell S.L., et al. Dural arteriovenous fistula of the anterior condylar confluence and hypoglossal canal mimicking a jugular foramen tumor. J Neurosurg. 2008;109(2):335-340.
81. Tirakotai W., Benes L., Kappus C., et al. Surgical management of dural arteriovenous fistulas with transosseous arterial feeders involving the jugular bulb. Neurosurg Rev. 2007;30(1):40-49.
82. Malik G.M., Mahmood A., Mehta B.A. Dural arteriovenous malformation of the skull base with intraosseous vascular nidus. Report of two cases. J Neurosurg. 1994;81(4):620-623.