Chapter 91A Vascular isolation
Overview
Bleeding and subsequent blood transfusions remain important causes of death and morbidity with hepatectomies. Evidence also shows that blood transfusion may be associated with an increased risk of recurrence of malignancy through impairment of the patient’s immune response (Asahara et al, 1999; Tung-Ping Poon et al, 2000). Vascular clamping, irrespective of its modality, minimizes the risk of bleeding during liver resection. Liver cell injury induced by ischemia and reperfusion is the major drawback of clamping. Overall, normal liver tolerates up to an hour of warm ischemia, but diseased liver with fibrosis, steatosis, inflammation, and vascular or biliary disease requires much shorter periods of ischemia. Indeed, the vast majority of liver resections are performed in such diseased livers, including resections of hepatocellular carcinoma (HCC) (see Chapter 70A, Chapter 70B, Chapter 81A ) and of liver metastases, which are more often performed after chemotherapy and can induce parenchymal changes (chemotherapy-associated steatohepatitis, sinusoidal obstruction syndrome) (see Chapters 65 and 81A).
With technical improvements in liver surgery, patients with various underlying liver conditions—such as fibrosis, steatosis, or chemotherapy-induced injury—are undergoing complex and extensive liver resections (Belghiti et al, 2000; Poon et al, 2002). In this regard, pedicular intermittent clamping, which alternates short periods of clamping with intervals of restoration of blood flow, has been shown to be the best tolerated clamping modality, especially in patients with diseased liver parenchyma (Belghiti et al, 1999; Man et al, 1997). Although a real advantage in clinical practice was not demonstrated (Gurusamy et al, 2009), ischemic preconditioning that initiates a short period of clamping and restoration of blood flow followed by continuous clamping is an attractive concept (Clavien et al, 2000). On the other hand, better understanding of vascular anatomy, particularly of the venous tributaries; better anesthesiologic management with perioperative low filling (restrictive fluid administration); and the development of modern tools of transection and coagulation, such as the ultrasonic dissector associated with bipolar coagulation, have made possible even major liver resection without vascular clamping or transfusion. Therefore if vascular clamping must be applied, balancing its efficacy to control bleeding with the potential side effects of ischemic liver injury is vital.
Anatomic Basis for Vascular Control
Three large hepatic veins lie posterosuperior to the liver just below the diaphragm and form the major drainage of the liver (Fig. 91A.1). The right hepatic vein is a short, wide trunk formed by the convergence of an anterior trunk situated in the right portal fissure, which drains mainly segments V and VI, and a posterior trunk, which drains mainly segment VII. The right hepatic vein also drains part of segment VIII. The middle hepatic vein is situated in the plane of principal portal fissure; it drains the entire central sector and receives the veins from segments V and VIII at its right border and the veins of segment IV at its left border, thus forming the major drainage vein of segment IV and part of segments V and VIII. The left hepatic vein arises from the confluence of the segment II and III veins. It often receives drainage from the posterior part of segment IV, and it terminates as a short common trunk with the middle hepatic vein in the majority. In 10% to 20% of cases, a significant right inferior hepatic vein may be found (>5 mm diameter), which mainly drains segment VI. In addition to these, there are two groups of accessory hepatic veins, the right and the left. The right accessory veins drain the posterior part of the dorsal sector. On the left, they are formed by the veins of the caudate lobe. In half of the cases, a large solitary vein terminates in the inferior vena cava (IVC); in the other half, two or three veins are present, and they end in a staged fashion in the left border of the IVC. These anatomic variations are important in the context of vascular clamping, as the presence of large drainage veins can result in the inability to achieve complete vascular control during the clamping procedure (see Chapter 1B).
Hemodynamic Response to Different Types of Clamping
Hemodynamic changes are proportional to the type and extent of vascular interruption. These changes are also related to several factors, including the level of anesthesia, quantity and rapidity of bleeding, vascular filling, and spontaneous adaptation of the circulatory system to the reduction of venous return. Pedicular clamping is nearly always well tolerated, but the addition of caval clamping is more likely to be followed by major hemodynamic consequences (Table 91A.1).
Hemodynamic Response to Pedicle Clamping
The pedicular clamping, which leads to a moderate decrease in cardiac preload with a 5% decrease in pulmonary artery pressure and a 10% decrease in cardiac index, is paradoxically associated with a 10% increase in mean arterial pressure because of a sympathetic reflex after arterial clamping that induces a significant increase in systemic vascular resistance (Delva et al, 1987). Therefore isolated pedicle clamping is usually well tolerated and does not require specific anesthetic management. During the period of declamping, blood pressure is restored to normal or even shows an increase from the baseline; however, it is a frequently observed phenomenon that, as the cycle of clamping and declamping progresses, declamping causes more significant hypotension. This phenomenon, which can be observed when the cumulative period of clamping lasts 1 hour, may be attributed to a reperfusion-like syndrome as a result of the long-term ischemia of liver parenchyma and splanchnic release of toxic vasodilators; therefore it is recommended to increase the duration of declamping during each cycle, if the cumulative duration is prolonged more than 1 hour, especially in patients with diseased liver (Imamura et al, 2003).
Hemodynamic Consequences and Limitations of Hepatic Vascular Exclusion
The hemodynamic consequences of the addition of total caval clamping to pedicular clamping is more profound (see Table 91A.1). Hepatic vascular exclusion (HVE), which leads to a severe decrease in cardiac preload with 25% decrease in pulmonary artery pressure and 40% to 50% decrease in cardiac index, is associated with a 10% decrease in mean arterial blood pressure despite a 50% increase in heart rate and a very significant increase in systemic vascular resistance. Reduced venous return produces a marked reduction in left ventricular and diastolic volume that could be precisely monitored by transesophageal echocardiography (Eyraud et al, 2002). The sequence of HVE includes initial inflow occlusion followed by infrahepatic caval clamping and then suprahepatic caval clamping. The compensatory cardiac response to maintain blood pressure requires approximately 5 minutes and is facilitated by “preloading” the patient with intravenous fluids. A fall in mean arterial pressure less than 80 mm Hg suggests intolerance to HVE and should lead to consideration of caval declamping. Ventricular dimensions could be monitored by transesophageal echocardiography (Eyraud et al, 2002).
Anesthetic Considerations (See Chapter 22)
Low Central Venous Pressure Anesthesia and Limited Filling
The CVP directly influences the occurrence of back bleeding during liver inflow occlusion. In a retrospective study, Smyrniotis and colleagues (2004) showed that the Pringle maneuver at a CVP of 6 mm Hg or more is associated with greater blood loss than the maneuver performed at a CVP of 5 mm Hg or less. Thus the concept of low CVP anesthesia during hepatectomy has emerged. Maintaining a low CVP during hepatic transection reduces the distension of hepatic veins and thus significantly decreases bleeding (Jones et al, 1998). It also avoids vena caval distension and thus facilitates safe dissection of the retrohepatic vena cava and major hepatic veins. As the blood loss resulting from a vascular injury is proportional to the pressure gradient across the vessel wall and to the fourth power of the radius of the injury, lowering the CVP to one fifth will decrease the blood loss by a factor greater than 5 (Melendez et al, 1998).
Low CVP during liver transection is consistently associated with reduced blood loss (Chen et al, 2000; Cunningham et al, 1994; Eid et al, 2005; Jones et al, 1998; Melendez et al, 1998; Wang et al, 2006). Moreover, in retrospective studies, low CVP tends to be associated with lower postoperative morbidity and reduction of hospital stay (Chen et al, 2000; Smyrniotis et al, 2004).
The potential drawbacks of low CVP anesthesia include perioperative occurrence of air embolism, requirement for pressor agents, and postoperative renal dysfunction. The incidence of gas embolism varies according to the approach and the surgical technique used for parenchymal transection. During a laparoscopic approach, transesophageal echocardiography detects, in nearly all cases, small volumes of air embolism (Schmandra et al, 2002, 2004). During liver resection using ultrasonic devices, air embolism was detected in all the patients who underwent liver resection with an ultrasonic surgical dissector (Koo et al, 2005). Although in the previous study, nearly half of the patients had air embolism filling more than half the right heart diameter, the incidence of clinically relevant accidents is rarely defined. Zhou and colleagues (2008) found an incidence of three cases in 110 liver resections performed with inflow occlusion, and liver surgery complicated by gas embolism accounted for 4% of the patients treated in a referral center for hyperbaric treatment (Bessereau et al, 2010).
Gas embolism usually originates in the venous system from hepatic vein injury through the parenchymal resection stump. Venous gas embolism can lead to arterial paradoxical embolism favored by a patent foramen ovale or intrapulmonary arteriovenous pulmonary shunting (Lee et al, 2002). During hepatic surgery, gas embolism is usually recognized by a sudden drop in end-tidal expiratory carbon dioxide, followed by a subsequent drop in arterial blood pressure or sudden cardiac arrest (Adachi et al, 2006). Arterial systemic embolism can cause arterial ischemia in cerebral, myocardial, spinal, or limb circulation (Lee et al, 2002). Gas embolism is considered a medical emergency, and treatment includes interruption of the embolic procedure, an attempt to extract intravascular gas, external cardiac massage, and immediate oxygenation. Despite an absence of high-level evidence, hyperbaric oxygen therapy is recommended in case of cardiac arrest or in any patient with an unexpected neurologic abnormality following a risky procedure (Blanc et al, 2002; Trytko & Bennett, 2008). But it should be kept in mind that hyperbaric therapy can be limited by the location of hyperbaric chambers, necessitating the transfer of critically ill patients; the ability of those chambers to be used to care for critically ill patients during the therapy; and the risk of hyperoxic seizures and barotrauma (Bessereau et al, 2010; Edsell & Kirk-Bayley, 2009).
The risk of postoperative renal failure linked to low-CVP anesthesia has been suggested in the setting of liver transplantation (Schroeder & Kuo, 2008). During liver resection, the incidence of postoperative renal dysfunction related to low intraoperative volemia has not been clearly established (Melendez et al, 1998). The ability of patients to tolerate fluid restriction in terms of renal function with improved recovery has led some authors to use a restrictive fluid policy in liver surgery. It has been shown in living donor resection that CVP monitoring did not reduce blood loss when a low-infusion policy was routinely applied (Niemann et al, 2007).
Maintaining Low Venous Backflow (See Chapter 22)
The anesthesiologist can maintain a low venous backflow by several methods. Fluid restriction during induction and liver transection is the most commonly used method. Intravenous fluids are given at a rate of 0.5 to 1 mL/kg/h, until the hepatic resection is complete. The patient is brought back to normovolemia at the end of surgery, and hemostasis is ensured. Other methods commonly used are the use of anesthetic gases such as isoflurane, which has systemic vasodilatory properties and results in minimal cardiac depression (Gatecel et al, 2003), and the use of certain drugs that produce vasodilatory effects. Although low tidal volume ventilation was considered to reduce the backflow bleeding, no evidence is available to support that this maneuver reduces the quantity of blood lost during the hepatic transection phase (Hasegawa et al, 2002). The surgeon can decrease the backflow bleeding by partially or totally clamping the infrahepatic IVC. This technique, which decreases venous return, has been shown to have no deleterious effect on renal function, even with a clamping time of up to an hour (Otsubo et al, 2004). The ideal tool for hemodynamic monitoring under these circumstances remains to be determined, although transesophageal echocardiography and pulmonary artery catheterization can be used (Eyraud et al, 2002). Intraoperative monitoring of fluid responsiveness could also be implemented noninvasively, using respiratory variations in arterial pulse pressure or monitoring of peripheral venous pressure (Choi et al, 2007; Solus-Biguenet et al, 2006). In experienced centers, CVP monitoring did not show any significant advantage (Niemann et al, 2007).
Special Considerations During Hepatic Vascular Exclusion
Application of vascular isolation techniques mandates a high level of anesthetic expertise. Intraoperative management of patients undergoing vascular exclusion should include the use of pulmonary artery catheterization and occasionally of transesophageal echocardiography. When a major hepatectomy performed under HVE is planned, anesthetic management is adjusted to anticipate the reduction in venous return, sudden decrease in cardiac output, and increase in afterload. Volume expansion is usually required for patients undergoing HVE. This is achieved by rapid infusion of 500 mL of colloids before cross clamping of the vena cava. In patients who do not tolerate caval cross clamping, even after volume expansion, vasopressor agents such as noradrenaline are added (Redai et al, 2004). Persistent hypotension and/or low cardiac index, which can occur in 10% to 20% of patients, should be considered intolerance to HVE and is an indication to unclamp the vena cava or to consider the use of venovenous bypass (Redai et al, 2004).
Surgical Aspects of Vascular Clamping
Inflow Vascular Clamping
The hepatic pedicle clamping (Pringle maneuver), which interrupts the arterial and portal venous inflow to the liver, is a standard in hepatic surgery. According to the Cochrane database, in elective resection, intermittent portal triad clamping seems better than continuous clamping, especially in patients with diseased parenchyma; therefore intermittent triad clamping could be recommended as the gold standard method of clamping (Gurusamy et al, 2007).
Total Inflow Control
Inflow control implies an encircling of the hepatic pedicle (Fig. 91A.2). Adhesions to the gallbladder are freed, and the lesser omentum is opened at the level of the pars flacida, taking care to avoid injury to the right gastric pedicle. A finger or a blunt dissector may thereafter be easily passed through the foramen of Winslow, and the hepatoduodenal ligament is encircled with tape. Clamping is easily achieved by a vascular clamp or tourniquet, which should be closed until the pulse in the hepatic artery distal to the clamp is stopped; excessive closure should be avoided, as it may otherwise result in arterial or biliary injury. Care should be taken to avoid lymph nodes of the hepatoduodenal ligament, as these may be large in cirrhotic patients or in patients with a long-standing cholestasis. A search for a left hepatic artery originating from the left gastric artery is mandatory to prevent persistent bleeding during parenchymal transection. When a left hepatic artery exists, simultaneous occlusion of this vessel should be performed to complete the vascular inflow control.
Continuous Clamping
Continuous clamping implies interruption of inflow continuously during the hepatic transection phase without intermittent release to allow reperfusion. Although conceptually very efficient to control bleeding, it is used less frequently, as it is not universally effective and has several disadvantages (Table 91A.2). The efficacy is limited by its inability to control the backflow bleeding, which is increased if the CVP is high or the patient has large hepatic tumors that compress hepatic veins associated with multiple venous collaterals.
Table 91A.2 Comparison of Relative Technical Difficulty, Control of Blood Loss, and Physiologic Tolerance for Various Clamping Techniques
Disadvantages of continuous clamping include splanchnic congestion and prolonged parenchymal ischemia. The splanchnic congestion and fluid sequestration in the visceral compartment cause bowel edema, which takes a long time to subside, leading to difficulties in the closure of the abdominal cavity at the end of the operation and to prolonged postoperative ileus. A difficult closure of the abdomen can lead to abdominal compartment syndrome with subsequent effects on the intraabdominal organs (Moore et al, 2004). Development of edema will be detrimental to bowel anastomoses, especially in the context of synchronous hepatic resections for colorectal malignancies (Elias et al, 1995). Continuous clamping has been shown to induce hyperamylasemia, and it can lead to clinically significant pancreatitis in some patients (Miyagawa et al, 1994, 1996). Interruption of splenic venous return without arterial interruption poses a risk for spontaneous splenic rupture during prolonged continuous clamping (Emree et al, 1993). Continuous clamping causes prolonged ischemia to the liver. Normal liver can tolerate inflow occlusion under normothermic conditions for up to 60 minutes (Delva et al, 1989; Huguet et al, 1992), but diseased livers are much less tolerant of extended ischemia, so continuous clamping cannot be applied to diseased livers for very long. Moreover, restoration of blood flow after prolonged clamping can cause reperfusion syndrome characterized by hemodynamic disturbances as a result of the release of toxic metabolites and vasodilators from the liver and the splanchnic bed (Kim, 2003; Kim et al, 2002). Intermittent clamping has been introduced to overcome these drawbacks of continuous clamping.
Intermittent Clamping
Intermittent inflow occlusion with intervals of reperfusion reduces the consequences of splanchnic congestion and decreases the ischemic injury to the liver parenchyma. After a short period of pedicular clamping, ranging from 10 to 20 minutes, the inflow is restored for 5 to 10 minutes, according to the duration of the transection, underlying liver status, and surgeon preference (Imamura et al, 2003). Classically, each cycle comprises 15 minutes of clamping followed by 5 minutes of reperfusion (Belghiti et al, 1999; Capussotti et al, 2003; Hardy et al, 1995; Man et al, 1997; Takayama et al, 1998). Although a randomized, controlled trial (RCT) that compared the short-term outcome of hepatectomy using intermittent clamping with an ischemic interval of 15 minutes instead of 30 minutes, with 5 minutes of reperfusion, showed no significant difference (Esaki et al, 2006), most authors favor 10 to 15 minutes of clamping.
Intermittent pedicle clamping, the most commonly used vascular clamping modality, has gained wide acceptance, as it is technically simple, effective in control of hemorrhage, and is well tolerated, above all, by diseased liver. Intermittent clamping of a diseased liver has no significant deleterious effects, even if the cumulative clamping time is up to 1 hour (Takayama et al, 1998). Two randomized, controlled studies compared continuous versus intermittent portal triad clamping and found that, although significantly more bleeding was noted in the intermittent group during parenchymal transection, no statistical difference was reported in the total operative blood loss (Belghiti et al, 1999; Capussoti et al, 2003). The most important result, however, was the better tolerance of diseased liver to intermittent clamping (Belghiti et al, 1999); thus the application of intermittent clamping in normal livers has prolonged the total clamping duration by up to 3 hours, which allows surgeons to perform even complex hepatic resections with minimal blood loss (Sakamoto et al, 1999).
The wide safety margin of intermittent pedicle clamping has served to promote its safe use in donor hepatectomy in living donor liver transplantation (LDLT). This has not jeopardized donor safety, nor has it compromised graft function (Imamura et al, 2002, 2004). The use of intermittent clamping enables a bloodless resection, thus it adds to donor safety, suggesting that surgeons should not hesitate to use this technique during surgery for live donation (Imamura et al, 2002, 2004). The better tolerance of intermittent ischemia has stimulated investigators to further explore the possible benefits of preconditioning.
Preconditioning
A newer perspective on inflow vascular clamping has emerged from study of the biologic response to ischemia and reperfusion (Clavien et al, 2000; Hardy et al, 1996). A period of ischemia followed by reperfusion of the liver not only protects the liver from the negative effects of any subsequent period of ischemia, but it may also protect distant organs from the systemic effects of organ ischemia; recognition of this phenomenon led to a study of this technique in humans. Clavien and colleagues (2000) revealed that an initial period of ischemia (10 minutes) followed by reperfusion (10 minutes) protects the liver against subsequent prolonged ischemia, and the authors postulated that some of the benefits of intermittent clamping may actually result from the impact of the first clamp-unclamp sequence as a preconditioning treatment.
In addition, preconditioning followed by continuous clamping may have the advantage of avoidance of blood loss during the unclamped period, although no major differences have been shown in terms of blood loss between the continuous versus intermittent clamping approaches. In a prospective randomized study, the authors showed that this protective strategy against hepatic ischemia was shown to be efficient, especially in the presence of steatosis (Clavien et al, 2003); however, the beneficial effect of preconditioning was shown only for 30 minutes of continuous clamping and only for a small volume of liver resection.
Although the theoretic advantage of a hepatoprotective effect of preconditioning is definitely attractive, in practice this procedure has not been shown to have significant advantages over intermittent clamping. An RCT that compared ischemic preconditioning versus continuous clamping (Chouker et al, 2004) showed improved cardiovascular stability by lowering the need for catecholamines after liver reperfusion. In contrast, a third RCT (Azoulay et al, 2006) did not confirm a beneficial effect of ischemic preconditioning. Furthermore, the application of ischemic preconditioning in the setting of liver transplantation has not been shown to be beneficial compared with conventional liver-harvesting methods for deceased or living donors (Azoulay et al, 2005; Koneru et al, 2005). In addition, a recent Cochrane analysis found no statistically significant difference in mortality rate, liver failure, blood loss, or hemodynamic changes (Gurusamy et al, 2007).
Pharmacologic preconditioning is a promising field, as a variety of substances have been proven effective in animal experiments, although very few concepts have made the transition to being applied to humans. In one RCT (Beck-Schimmer et al, 2008), 64 patients undergoing liver surgery with inflow occlusion were randomized to 30 minutes of intraoperative preconditioning with sevoflurane or anesthesia with propofol. Thirty minutes before inflow occlusion, propofol was replaced by sevoflurane in the preconditioning group, and it was found that preconditioning with sevoflurane significantly reduced postoperative aspartate aminotransferase and alanine transaminase levels. Furthermore, the overall incidence of postoperative complications and the number of major events was reduced, and this beneficial effect was stronger in patients with hepatic steatosis.
Topical Hypothermia
As hepatic inflow occlusion carries a serious risk of ischemic injury to the remnant liver, induction of hypothermia was proposed to reduce the injury, particularly in patients with underlying liver disease (Kim et al, 1994, 2004). The adverse hemodynamic effects of systemic hypothermia has led some authors to use topical cooling by placing ice packs or ice slush on the liver surface (Imakita et al, 2000; Yamanaka et al, 1998); using such techniques, ischemic time could be prolonged for more than an hour in diseased livers, especially if the hepatic core temperature fell below 30° C (Kim et al, 2004).
Selective Inflow Control
Selective inflow control selectively interrupts the arterial and portal venous inflow to the hemiliver to be resected. The advantages of this procedure include 1) clear demarcation of the limits of resection, 2) absence of ischemic injury of the remnant liver, 3) absence of splanchnic congestion, and 4) hemodynamic disturbances. Selective inflow control was specifically devised for segmental and subsegmental resections for small hepatocellular carcinomas in diseased liver (Shimamura et al, 1986; Castaing et al, 1989). Additional clamping of the ipsilateral hepatic vein can also be performed along with this procedure for better vascular control (Makuuchi et al, 1987; Fig. 91A.3) With the wider use of intermittent clamping, selective clamping techniques have not gained wide acceptance, as this is more technically demanding and has no obvious beneficial effects compared with genuine intermittent clamping. In a prospective controlled study that included patients who underwent minor resection, both techniques were associated with similar blood loss and postoperative complications (Figueras et al, 2005); however, induction of lesser cytolysis was observed in those in the cirrhotic group who underwent selective clamping.
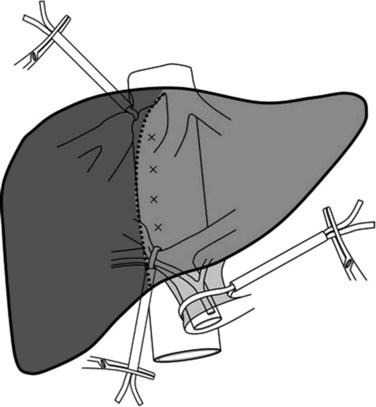
FIGURE 91A.3 Hemihepatic vascular clamping interrupts the inflow and outflow of the hemiliver to be resected.
Inflow and Outflow Vascular Control
Hepatic Vascular Exclusion
HVE combines total inflow and outflow vascular occlusion of the liver. Total isolation of the liver from the systemic circulation is intended during resection of large tumors adjacent to or involving the major hepatic veins and/or the IVC. This procedure can also be considered when significant backflow bleeding occurs as a result of persistently elevated CVP despite efficient hepatic pedicle clamping (e.g., in a patient with tricuspid insufficiency). This procedure, which requires prolonged continuous clamping, cannot be tolerated in patients with diseased liver and can cause significant hemodynamic disturbance in some patients (see Table 91A.2).
Effective HVE requires complete mobilization of the liver from its ligamentous attachments and from all surrounding adhesions. The IVC is completely freed from the retroperitoneum, which requires the ligation of the right adrenal vein. The IVC is mobilized above and below the liver and is encircled. The hepatoduodenal ligament is encircled as described above, and a careful search is made for accessory or replaced hepatic arteries (Fig. 91A.4). Clamps are applied in the following order: 1) hepatoduodenal ligament, 2) infrahepatic IVC, and 3) suprahepatic IVC. Once the surgeon and the anesthesiologist agree that clamping will be tolerated, clamps are reapplied for up to 60 minutes in patients with a normal liver. After completion of the parenchymal transection, prior to removing the clamps, the clamp on the infrahepatic vena cava can be partially released to flush air that might have been trapped and to test for caval integrity. The clamps are then removed in reverse order of placement.
Supracaval declamping provides the path of least resistance for blood from the liver to flow toward the heart rather than backward into the surgical field. Although concomitant supraceliac aortic clamping has been proposed in this situation, the technique has not been widely adopted. Three randomized studies (Belghiti et al, 1996; Chen et al, 2006; Smyrniotis et al, 2002) compared total vascular exclusion (TVE) with portal pedicle clamping or selective vascular exclusion and showed that both techniques were effective in bleeding control. No statistically significant difference was reported in the operative blood loss, number of patients transfused, or number of units transfused (Gurusamy et al, 2007), and no difference in mortality was found between the two groups; however, TVE was associated with significant decrease in mean arterial pressure and mean pulmonary arterial pressure. Postoperative serum creatinine level and length of hospital stay were significantly greater in the TVE group (Gurusamy et al, 2007). Finally, TVE requires prolonged continuous clamping that can be poorly tolerated in patients with diseased liver.
Hepatic Vascular Exclusion with Caval Flow Preservation
Better understanding of intrahepatic anatomy and advances in surgical techniques allow many liver surgeons to safely expose and control the three main hepatic veins. In the absence of tumor involvement of the hepatocaval junction, HVE with caval flow preservation can be considered. The association of inflow occlusion (hepatic pedicle clamping) and outflow occlusion by extraparenchymal clamping of the three main hepatic veins (Fig. 91A.5) enables complete vascular isolation without interruption of the IVC flow (Cherqui et al, 1999; Elias et al, 1995); however, this attractive procedure is restricted to patients without tumor involvement of the hepatocaval junction. In addition, the presence of large veins draining the caudate lobe may render this procedure ineffective. Caudate lobe veins are not isolated in this technique and can communicate intraparenchymally with other major veins, causing persistent backflow bleeding. The technical sequence of HVE with preserved caval flow includes total pedicular clamping with subsequent clamping of the three major hepatic veins at their confluence with the IVC. This clamping procedure can be applied either continuously or intermittently.
After mobilization of the right lobe, and upon complete exposure of the right and anterior side of the IVC, the liver is retracted medially and upward. The right half of the IVC is dissected caudally to cranially with ligation of short and small retrohepatic veins. When a significant right inferior hepatic vein is present, it is either encircled or ligated and then divided according to the type of resection. Before reaching the RHV level, the IVC ligament is encountered, which is dissected and divided between clamps; because it contains small veins, it should be closed with a running suture. Only after this has been completed should the RHV be encircled and taped, which is achieved easily by placement of a clamp that passes on the anterior surface of the IVC toward the space between the RHV and MHV. To facilitate the subsequent parenchymal dissection, a tape is inserted behind the RHV and is passed along the anterior surface of the IVC (Fig. 91A.6), and the right liver is released to lie in its anatomic position. The tape that elevates the precaval space, the so-called hanging maneuver, facilitates subsequent division and hemostasis of the parenchyma (Belghiti et al, 2001).
Extrahepatic control of the middle and left hepatic veins requires exposure of left border of the IVC. The left upper aspect of the IVC is exposed by division of the peritoneal reflection above the caudate lobe, and the ligamentum venosum is ligated and divided to expose the junction of left hepatic vein (LHV) and the IVC (Majno et al, 2002). When the left phrenic vein drains directly into the LHV, the former is ligated and divided. A dissector is inserted from above in the previously dissected gutter between the right and middle hepatic veins and is passed in close contact with the anterior surface of the IVC beneath the MHV. The common trunk is then encircled with a tape. When the confluence of the middle and left hepatic veins is extrahepatic, it is possible to separately encircle these two vessels; otherwise the common trunk of the middle and left hepatic veins can be encircled as a unit.
Hepatic Vascular Exclusion with Hypothermia
Initially described by Fortner and colleagues (1974), this technique has been reevaluated by Azoulay and others, especially when HVE was longer than 60 minutes in duration (Azoulay et al, 2005). Compared with standard HVE, this group showed that hypothermic perfusion of the liver was associated with better postoperative liver and renal functions and less morbidity, especially in patients who required more than 60 minutes of HVE, although the technique requires systematic venovenous bypass for all patients. In the study, cooling was performed using 4 to 8 L of University of Wisconsin solution chilled to 4° C, perfused through the PV, and let out through an incision above the inferior caval clamp. To expedite the process, surface cooling using cold packs, ice, or cold water may be used. Intraparenchymal temperature should ideally be monitored using a fine needle, and the objective is to range between 14° C and 18° C. This renewed attractive procedure is applicable in difficult and complex liver resection in patients with diseased liver, and it should be added to the armamentarium of modern surgery.
Isolated Caval Clamping
Infrahepatic Caval Clamping
The major impact of caval pressure on blood loss during liver resection has led some surgeons to propose isolated IVC clamping to decrease the CVP (Fig. 91A.7; Abdalla et al, 2004; Otsubo et al, 2004). This technique is simple and is usually hemodynamically well tolerated, and it can decrease the CVP by approximately 4 cm of H2O and can be used alone, when the liver resection is performed without pedicular clamping or along with pedicular clamping. When it is used in fluid-loaded patients, the hemodynamic impact on arterial pressure and postoperative renal function is minimal (Otsubo et al, 2004); therefore surgeons should consider this method, especially when the anesthesiologist cannot maintain a low CVP.
Isolated Total Caval Clamping
Clamping of the infrahepatic vena cava before liver transection can be considered in patients with a persistently high CVP (Otsubo et al, 2004). When a suprahepatic venous trunk is not involved by a tumor that otherwise involves the vena cava, total caval clamping below the hepatic veins associated with clamping of the vena cava but above renal veins maintains hemodynamic stability (Varma et al, 2007). This technique of caval clamping is indicated in patients with tumors in contact with the vena cava or near the cavohepatic junction, which necessitates en bloc resection of the IVC or cavohepatic junction. In this maneuver, the vena cava alone is clamped, leaving the inflow and outflow of the remnant liver intact; this procedure requires the control of the infrahepatic vena cava and of the uninvolved venous trunk.
The initial phase of the procedure consists of parenchymal transection by an anterior approach, usually under intermittent clamping after ligation of the hepatic pedicle to the tumor-bearing hemiliver. When the parenchymal transection reaches the anterior surface of the vena cava, the tape that encircled the uninvolved venous trunk is passed behind the suprahepatic IVC. The IVC is clamped at the site of this caval tape after clamping the infrahepatic vena cava. Thus the vena cava is isolated while hepatic flow is maintained through the remnant liver (Fig. 91A.8). The maintenance of the flow to the suprahepatic vein through the “liver shunt” avoids splanchnic congestion, hemodynamic disturbances, and liver ischemia. Total caval clamping without liver ischemia allows comfortable caval resection and reconstruction. This procedure, which is especially indicated, is efficient when the caudate lobe is involved and resected, which further reduces the indication of HVE to exceptional patients.
Nonclamping Technique
Several conditions should be satisfied to perform a hepatectomy without clamping: 1) fluid must be restricted, 2) completely preserved remnant liver venous drainage should be assessed preoperatively with CT scan and intraoperatively by Doppler US, and 3) the surgeon should possess modern tools of parenchymal transection. Bleeding from the transection surface is minimized when the remnant liver has a patent venous drainage; therefore a nonclamping technique should be considered essentially in major anatomic resections that preserve complete venous drainage of the remnant liver. Nonclamping increases liver transection time, which can be reduced by the use of several technical refinements that include use of an ultrasonic dissector, bipolar scissors, or water-irrigated bipolar forceps. As minimizing blood loss remains the major objective, the pedicle should be controlled and clamped if the blood loss approaches 20 mL/kg (Scatton et al, 2004). A recent randomized trial has shown that major liver resection can be performed without clamping (Capussotti et al, 2006).
Abdalla EK, et al. Hepatic vascular occlusion: which technique? Surg Clin North Am. 2004;84:563-585.
Adachi YU, et al. Cardiac arrest by venous air embolism during hepatic resection using the Cavitron ultrasonic surgical aspirator. Anesth Analg. 2006;103:493-494.
Asahara T, et al. Perioperative blood transfusion as a prognostic indicator in patients with hepatocellular carcinoma. World J Surg. 1999;23:676-680.
Azoulay D, et al. Effects of 10 minutes of ischemic preconditioning of the cadaveric liver on the graft’s preservation and function: the yin and the yang. Ann Surg. 2005;242(1):133-139.
Azoulay D, et al. In situ hypothermic perfusion of the liver versus standard total vascular exclusion for complex liver resection. Ann Surg. 2005;24:277-285.
Azoulay D, et al. Ischemic preconditioning for major liver resection under vascular exclusion of the liver preserving the caval flow: a randomized prospective study. J Am Coll Surg. 2006;202:203-211.
Beck-Schimmer B, et al. A randomized controlled trial on pharmacological preconditioning in liver surgery using a volatile anesthetic. Ann Surg. 2008;248:909-918.
Belghiti J, et al. Portal triad clamping or hepatic vascular exclusion for major liver resection: a controlled study. Ann Surg. 1996;224:155-161.
Belghiti J, et al. Continuous versus intermittent portal triad clamping for liver resection: a controlled study. Ann Surg. 1999;229:369-375.
Belghiti J, et al. Seven hundred forty-seven hepatectomies in the 1990s: an update to evaluate the actual risk of liver resection. J Am Coll Surg. 2000;191:38-46.
Belghiti J, et al. Liver hanging maneuver: a safe approach to right hepatectomy without liver mobilization. J Am Coll Surg. 2001;193:109-111.
Bessereau J, et al. Long-term outcome of iatrogenic gas embolism. Intensive Care Med. 2010;36:1180-1187.
Blanc P, et al. Iatrogenic cerebral air embolism: importance of an early hyperbaric oxygenation. Intensive Care Med. 2002;28:559-563.
Capussotti L, et al. Continuous versus intermittent portal triad clamping during hepatectomy in cirrhosis: results of a prospective, randomized clinical trial. Hepatogastroenterology. 2003;50:1073-1077.
Capussotti L, et al. Randomized clinical trial of liver resection with or without hepatic pedicle clamping. Br J Surg. 2006;93:685-689.
Castaing D, et al. Segmental liver resection using ultrasound-guided selective portal venous occlusion. Ann Surg. 1989;210:20-23.
Chen H, et al. Hepatic resection using intermittent vascular inflow occlusion and low central venous pressure anesthesia improves morbidity and mortality. J Gastrointest Surg. 2000;4:162-167.
Chen XP, et al. Modified technique of hepatic vascular exclusion: effect on blood loss during complex mesohepatectomy in hepatocellular carcinoma patients with cirrhosis. Langenbecks Arch Surg. 2006;391:209-215.
Cherqui D, et al. Hepatic vascular exclusion with preservation of the caval flow for liver resections. Ann Surg. 1999;230:24-30.
Choi SJ, et al. Can peripheral venous pressure be an alternative to central venous pressure during right hepatectomy in living donors? Liver Transpl. 2007;13:1414-1421.
Chouker A, et al. Effects of Pringle manoeuvre and ischaemic preconditioning on haemodynamic stability in patients undergoing elective hepatectomy: a randomized trial. Br J Anaesth. 2004;93:204-211.
Clavien PA, et al. Protective effects of ischemic preconditioning for liver resection performed under inflow occlusion in humans. Ann Surg. 2000;232:155-162.
Clavien PA, et al. A prospective randomized study in 100 consecutive patients undergoing major liver resection with versus without ischemic preconditioning. Ann Surg. 2003;238:843-850. discussion 851-852
Cunningham JD, et al. One hundred consecutive hepatic resections: blood loss, transfusion, and operative technique. Arch Surg. 1994;129:1050-1056.
Delva E, et al. Hemodynamic effects of portal triad clamping in humans. Anesth Analg. 1987;66:864-868.
Delva E, et al. Vascular occlusions for liver resections: operative management and tolerance to hepatic ischemia: 142 cases. Ann Surg. 1989;209:211-218.
Edsell ME, Kirk-Bayley J. Hyperbaric oxygen therapy for arterial gas embolism. Br J Anaesth. 2009;103:306.
Eid EA, et al. Low central venous pressure anesthesia in major hepatic resection. Middle East J Anesthesiol. 2005;18:367-377.
Elias D, et al. Intermittent vascular exclusion of the liver (without vena cava clamping) during major hepatectomy. Br J Surg. 1995;82:1535-1539.
Elias D, et al. Is simultaneous hepatectomy and intestinal anastomosis safe? Am J Surg. 1995;169:254-260.
Emree S, et al. Liver resection under total vascular isolation: variations on a theme. Ann Surg. 1993;217:15-19.
Esaki M, et al. Randomized clinical trial of hepatectomy using intermittent pedicle occlusion with ischaemic intervals of 15 versus 30 minutes. Br J Surg. 2006;93:944-951.
Eyraud D, et al. Hemodynamic and hormonal responses to the sudden interruption of caval flow: insights from a prospective study of hepatic vascular exclusion during major liver resections. Anesth Analg. 2002;95:1173-1178.
Figueras J, et al. Complete versus selective portal triad clamping for minor liver resection: a prospective randomized trial. Ann Surg. 2005;241:582-590.
Fortner JG, et al. Major hepatic resection using vascular isolation and hypothermic perfusion. Ann Surg. 1974;180:644-652.
Gatecel C, et al. The postoperative effects of halothane versus isoflurane on hepatic artery and portal vein blood flow in humans. Anesth Analg. 2003;96:740-745.
Gurusamy KS, et al. Methods of vascular occlusion for elective liver resections. Cochrane Database Syst Rev. Oct 17(4), 2007. CD006409
Gurusamy KS, et al. Ischaemic pre-conditioning for elective liver resections performed under vascular occlusion. Cochrane Database Syst Rev. Jan 21(1), 2009. CD007629
Hardy KJ, et al. Comparison of continuous versus intermittent ischaemia-reperfusion during liver resection in an experimental model. Br J Surg. 1995;82:833-836.
Hardy KJ, et al. Ischaemic preconditioning of the liver: a preliminary study. Aust N Z J Surg. 1996;66:707-710.
Hasegawa K, et al. Effect of hypoventilation on bleeding during hepatic resection: a randomized controlled trial. Arch Surg. 2002;137:311-315.
Huguet C, et al. Liver ischemia for hepatic resection: where is the limit? Surgery. 1992;111:251-259.
Huguet C, et al. Technique of hepatic vascular exclusion for extensive liver resection. Am J Surg. 1992;163:602-605.
Imakita M, et al. Does topical cooling alleviate ischemia/reperfusion injury during inflow occlusion in hepatectomy? Results of an experimental and clinical study. Surg Today. 2000;30:795-804.
Imamura H, et al. One thousand fifty-six hepatectomies without mortality in 8 years. Arch Surg. 2003;138:1198-1206. discussion 1206
Imamura H, et al. Pringle’s manoeuvre in living donors. Lancet. 2002;360:2049-2050.
Imamura H, et al. Pringle’s maneuver and selective inflow occlusion in living donor liver hepatectomy. Liver Transpl. 2004;10:771-778.
Jones RM, et al. Central venous pressure and its effect on blood loss during liver resection. Br J Surg. 1998;85:1058-1060.
Kim YI. Ischemia-reperfusion injury of the human liver during hepatic resection. J Hepatobiliary Pancreat Surg. 2003;10:195-199.
Kim YI, et al. In situ and surface liver cooling with prolonged inflow occlusion during hepatectomy in patients with chronic liver disease. Arch Surg. 1994;129:620-624.
Kim YI, et al. Enhanced inflammatory cytokine production at ischemia/reperfusion in human liver resection. Hepatogastroenterology. 2002;49:1077-1082.
Kim YI, et al. 101 hepatectomies under continuous inflow occlusion following simple in-situ liver cooling in patients with chronic liver diseases. Hepatogastroenterology. 2004;51:1093-1098.
Koneru B, et al. Ischemic preconditioning in deceased donor liver transplantation: a prospective randomized clinical trial of safety and efficacy. Liver Transpl. 2005;11:196-202.
Koo BN, et al. Hepatic resection by the Cavitron Ultrasonic Surgical Aspirator increases the incidence and severity of venous air embolism. Anesth Analg. 2005;101:966-970.
Lee SY, et al. Paradoxical air embolism during hepatic resection. Br J Anaesth. 2002;88:136-138.
Majno PE, et al. Arantius’ ligament approach to the left hepatic vein and to the common trunk. J Am Coll Surg. 2002;195:737-739.
Makuuchi M, et al. Safety of hemihepatic vascular occlusion during resection of the liver. Surg Gynecol Obstet. 1987;164:155-158.
Man K, et al. Prospective evaluation of Pringle maneuver in hepatectomy for liver tumors by a randomized study. Ann Surg. 1997;226:704-713.
Melendez JA, et al. Perioperative outcomes of major hepatic resections under low central venous pressure anesthesia: blood loss, blood transfusion and the risk of postoperative renal dysfunction. J Am Coll Surg. 1998;187:620-625.
Miyagawa S, et al. Changes in serum amylase level following hepatic resection in chronic liver disease. Arch Surg. 1994;129:634-638.
Miyagawa S, et al. Serum amylase elevation following hepatic resection in patients with chronic liver disease. Am J Surg. 1996;171:235-238.
Moore AF, et al. Intra-abdominal hypertension and the abdominal compartment syndrome. Br J Surg. 2004;91:1102-1110.
Niemann CU, et al. Central venous pressure monitoring during right donor hepatectomy. Liver Transpl. 2007;13:266-271.
Otsubo T, et al. Bleeding during hepatectomy can be reduced by clamping the inferior vena cava below the liver. Surgery. 2004;135:67-73.
Poon RT, et al. Extended hepatic resection for hepatocellular carcinoma in patients with cirrhosis: is it justified? Ann Surg. 2002;236:602-611.
Redai I, et al. Anesthetic considerations during liver surgery. Surg Clin North Am. 2004;84:401-411.
Sakamoto Y, et al. Pringle’s maneuver lasting 322 min. Hepatogastroenterology. 1999;46:457-458.
Scatton O, et al. Major liver resection without clamping: a prospective reappraisal in the era of modern surgical tools. J Am Coll Surg. 2004;199:702-708.
Schmandra TC, et al. Transoesophageal echocardiography shows high risk of gas embolism during laparoscopic hepatic resection under carbon dioxide pneumoperitoneum. Br J Surg. 2002;89:870-876.
Schmandra TC, et al. Risk of gas embolism in hand-assisted versus total laparoscopic hepatic resection. Surg Technol Int. 2004;12:137-143.
Schroeder RA, Kuo PC. Pro: low central venous pressure during liver transplantation—not too low. Cardiothorac Vasc Anesth. 2008;22:311-314.
Shimamura Y, et al. Selective portal branch occlusion by balloon catheter during liver resection. Surgery. 1986;100:938-941.
Smyrniotis V, et al. Total versus selective hepatic vascular exclusion in major liver resections. Am J Surg. 2002;183:173-178.
Smyrniotis V, et al. The role of central venous pressure and type of vascular control in blood loss during major liver resections. Am J Surg. 2004;187:398-402.
Solus-Biguenet H, et al. Non-invasive prediction of fluid responsiveness during major hepatic surgery. Br J Anaesth. 2006;97:808-816.
Takayama T, et al. Selective and unselective clamping in cirrhotic liver. Hepatogastroenterology. 1998;45:376-380.
Trytko BE, Bennett MH. Arterial gas embolism: a review of cases at Prince of Wales Hospital, Sydney, 1996 to 2006. Anaesth Intensive Care. 2008;36:60-64.
Tung-Ping Poon R, Fan ST, Wong J. Risk factors, prevention, and management of postoperative recurrence after resection of hepatocellular carcinoma. Ann Surg. 2000;232:10-24.
Varma D, Ogata S, Belghiti J. Isolated total caval clamping with “preserved remnant liver perfusion” for combined hepatic and venacaval resection in tumors involving vena cava. Surgery. 2007;141:112-116.
Wang WD, et al. Low central venous pressure reduces blood loss in hepatectomy. World J Gastroenterol. 2006;12:935-939.
Yamanaka N, et al. Historical evolution of hypothermic liver surgery. World J Surg. 1998;22:1104-1107.
Zhou W, et al. Selective hepatic vascular exclusion and Pringle maneuver: a comparative study in liver resection. Eur J Surg Oncol. 2008;34:49-54.