Chapter 11 Vascular Emergencies
ARTERIAL EMERGENCIES
Improved diagnostic methods and increasing specialization have enhanced our ability to treat vascular emergencies. The treatment of these patients has changed radically in recent years, becoming increasingly complex and involving a team approach, of which diagnostic and interventional radiologists are essential elements. In the evaluation of vascular emergencies the role of catheter angiography as a diagnostic tool is being progressively replaced by computed tomographic angiography (CTA) and sometimes magnetic resonance angiography (MRA). With technological advances these modalities, and particularly multidetector CTA (MD-CTA), have become an integral part in the initial assessment of acute vascular insults, as they are minimally invasive techniques, currently available in most emergency departments and trauma centers, and permit a prompt and accurate diagnosis within a short period of time. These are the methods of choice for the diagnosis of patients who do not have an indication for immediate surgical exploration. In addition, patients with direct signs (Box 11-1) of vascular injury on CTA can be taken to surgery without diagnostic angiography given its high sensitivity for the detection of vascular injuries. Furthermore, CT/CTA can be safely performed in patients with metallic fragments from bullets or other foreign objects, as opposed to MRI/MRA. MD-CTA has been increasingly used to diagnose arterial injuries from blunt and penetrating trauma not only to the chest and abdomen but also to the neck and extremities.
Arterial Emergencies of the Neck
Trauma of the Extracranial Carotid and Vertebral Arteries
Classically, the neck is divided into three zones for injury classification and management purposes. Zone 1 is from the clavicles to the cricoid cartilage, zone 2 is from the cricoid to the angle of the mandible, and zone 3 is from the angle of the mandible to the skull base. Zones 1 and 3 are extremely difficult to approach surgically. Injuries to zone 2 are the most common (60% to 70%), and this represents a clinical advantage since they are readily accessible for physical examination and surgery. The management of unstable patients who have suffered a penetrating neck injury is emergency surgical exploration. For the hemodynamically stable patients who present with a wound that penetrates the platysma there is still some controversy, as the local standards of care and resources may vary (Fig. 11-1). In general, surgeons opt for a conservative approach starting with CT/CTA and use additional diagnostic studies or take the patient to surgery if medically indicated. CTA has high sensitivity and specificity with reported values for the diagnosis of cervical vascular injuries ranging between 90% and 100%, respectively. The routine use of conventional angiography has been discouraged because of the high number of examinations with negative findings and the potential risk for complications due to its invasive nature. Nevertheless, the presence of any indirect sign (Box 11-2) around the carotid artery should warrant a correlation with angiography given the potential catastrophic consequences of missing a lesion in this location.
Blunt traumatic injuries of the extracranial carotid and vertebral arteries may have potential devastating consequences with an incidence that oscillates between 0.33% and 2.7% according to reports from centers performing aggressive angiographic screening. Although CTA and MRA have a great potential as screening tools in patients with blunt cerebrovascular injury, catheter angiography is still considered the gold standard. Intimal dissection and occlusion are more common with blunt trauma than with penetrating injuries (Fig. 11-2). Approximately 10% of patients have focal neurologic findings on initial presentation, and two thirds of the patients develop symptoms within 24 hours. The remainder may present with neurological findings weeks to months later.
The type of intervention performed for carotid and vertebral injuries is determined by the nature of the lesion, the symptoms and clinical condition of the patient, and the feasibility of accessing the injured segment either with open surgery or with an endovascular approach. In general, intimal flaps and nonocclusive dissections are managed with anticoagulation provided there are no contraindications. Stent placement is reserved for flow-limiting dissections, while covered stents (stent grafts) have been successfully used to treat pseudoaneurysms and arteriovenous arteriovenous (AV) fistulas with good immediate results and patency rates (see Fig. 11-1). Embolization is another alternative for cases of vascular transection where surgical or endovascular vessel wall reconstruction cannot be achieved and adequate collateral pathway is demonstrated.
Nontraumatic Emergencies of the Carotid and Vertebral Arteries
Carotid and Vertebral Artery Dissection
Spontaneous dissection of the carotid or vertebral arteries in the absence of trauma is rare. It usually occurs in patients with underlying history of hypertension, fibromuscular dysplasia, or an underlying collagen disorder such as Marfan or Ehlers-Danlos syndrome. It can occur at any age, and clinical manifestations are variable, including neck pain or ipsilateral headache, Horner syndrome (ptosis, myosis, and unilateral anhydrosis), and acute or chronic focal neurologic events either in the form of transient ischemic attack or as a permanent neurological deficit. All imaging modalities including ultrasound, CT, and MRI can be used to demonstrate the intimal flap, a compressed true lumen, slow flow in the false lumen, or even thrombus formation. Catheter angiography is generally indicated to better assess flow patterns or the absence of flow (see Fig. 11-2). The majority of patients are successfully treated with anticoagulation, but stent placement in the true lumen may be necessary to maintain or restore cerebral perfusion.
Aortic Emergencies
Acute aortic syndromes encompass a spectrum of aortic emergencies that include traumatic aortic injury (TAI), aortic dissection, penetrating atherosclerotic ulcer of the aorta, intramural hematoma, and aortic aneurysm rupture. Chapter 2 covers the topics of aortic dissection, intramural hematoma, and penetrating ulcer. This section focuses on traumatic aortic injuries.
TAI is a novel term that encompasses a spectrum of injuries characterized by a variable degree of aortic wall laceration and that include intimal tear, intramural hematoma, traumatic dissection, traumatic pseudoaneurysm, and, in the most severe form, aortic transection. TAI may result from rapid decelerations, crush injuries, penetrating wounds, and surgical or angiographic instrumentation. Blunt trauma is the most frequent cause of TAI, usually as the result of shearing, hydrostatic forces, and/or torsion forces applied along the aortic arch during a motor vehicle accident and falls from heights. Less common causes include displaced clavicular and thoracic vertebral fractures with entrapment of the aorta between the anterior chest wall and the spine. The overall mortality at the scene of the accident has been reported to be as high as 80% in autopsy series, with only 10% to 20% of the victims surviving the initial trauma. Bleeding from a laceration or rupture can be controlled by the aortic adventia or the periaortic tissues, which can help the patient survive the initial injury; however, the end result is pseudoaneurysm formation, which, due to its instability, requires prompt diagnosis and treatment (Fig. 11-3). The region of the aorta most susceptible to blunt injury is the isthmus, where the relatively mobile proximal thoracic aorta and arch join the fixed distal arch at the insertion of the ligamentum arteriosum, just distal to the left subclavian artery origin (Fig. 11-4). This area is involved in as many as 90% to 95% of the cases. Other common areas of injury are the aortic root and the diaphragmatic hiatus. Injuries to the ascending aorta are uncommon (5% to 9%) and usually lethal due to the lack of surrounding connective tissue, resulting in rapid death due to exsanguination or cardiac tamponade. Clinical suspicion as well as a prompt diagnosis and treatment remain of crucial importance in TAI, given that 30% of the victims will die within 6 hours and 40% to 50% will die within 24 hours.
The initial imaging screening of trauma patients is obtained with plain radiographs due to the availability and capacity to quickly assess injuries such as pneumothorax, hemothorax, mediastinal hematoma (Fig. 11-5), and fractures. Clinical signs and symptoms found in patients with TAI include chest pain, back pain, dyspnea, cough, hoarseness, hypotension, pulse discrepancy, shock, and coma; as many as 30% to 50% of these patients may not show external signs of trauma.
Catheter aortogram has long been considered the gold standard for the diagnosis of TAI with reported sensitivities and specificities approaching 100%. However, MD-CTA has become the preferred method for screening major traumatic and nontraumatic aortic emergencies, thanks to its improved spatial and contrast resolution and supplemental postprocessing techniques (thin sections, multiplanar reconstructions [MPRs], three-dimensional volume-rendered images), with a performance that rivals that of catheter angiography. MD-CTA has helped to better characterize the location and extent of TAI and other vascular injuries, allowing for faster diagnosis and treatment planning. In addition, MD-CTA can also recognize a greater number of normal variants of the vascular anatomy and subtle vascular injuries, which were less likely to be seen with conventional CT scans (Fig. 11-6). Furthermore, the need to perform an aortography to confirm the diagnosis of TAI is eliminated when direct signs of injury are present on CTA. Direct signs of TAI in CT include active extravasation of contrast, pseudoaneurysm formation, irregularity of the aortic wall, abrupt change in caliber of the aorta, aortic dissection, intimal flaps, and filling defects. Indirect signs of possible aortic injury include periaortic hematoma and mediastinal hematoma (Fig. 11-7).
Treatment
Alternative management of TAI consists of delayed operative intervention or no operation while the patient is kept under close monitoring and blood pressure (BP) control to reduce the risk of free rupture. The mainstay of treatment is to maintain systolic BP below 120 mm Hg (mean BP below 80 mm Hg) and allow patients who have suffered associated severe trauma to stabilize before surgical repair. This approach is indicated in hemodynamically stable patients with small aortic tears or those with associated injuries such as significant head, cardiac, or pulmonary trauma, large body surface burns, contaminated wounds, large retroperitoneal hematomas, or other high-risk medical comorbidities. Some of these aortic injuries might develop into a chronic pseudoaneurysm (Fig. 11-8), and others might even resolve during the period of observation. Close clinical and imaging follow-up is imperative to detect injury progression or aortic rupture.
More recently, endovascular treatment of TAI using stent grafts has been introduced as an alternative in the management of hemodynamically stable patients and patients with contraindications to cardiopulmonary bypass, such as severe coagulopathy, extensive additional injuries, and severe underlying cardiac or pulmonary disease. Mortality and morbidity appear to be lower than those of surgery, most likely because of the less invasive nature of the procedure, shorter operation time, and lack of the surgery-related comorbidities. The stent grafts are delivered via a common femoral artery cut-down access and positioned under angiographic and fluoroscopic guidance. The left subclavian artery origin often has to be covered with the endograft to provide adequate proximal support without significant clinical consequence in many patients. A significant number of patients with TAI are young, and therefore their aortic size is small; most stent grafts available today are made for the treatment of larger aortas with degenerative aortic aneurysms. Even though it is usually recommended to oversize the stent graft by 10% to 20% to ensure an adequate seal, excessive oversizing has been associated with type I endoleaks (Table 11-1) and stent graft collapse. Complications related to the endovascular repair include groin hematoma, iliofemoral dissection, endoleak, graft migration, and arterial rupture. Overall morbidity rates have been found to be around 12% with mortality rates of 4%. Clinical and imaging follow-up in both surgical and endovascular treatment modalities is required for early identification of post-treatment complications.
Endoleak type I | Inadequate seal around the proximal or distal end of the stent graft |
Endoleak type II | Retrograde flow from branch vessels (intercostal, lumbar, inferior mesenteric, gonadal arteries) resulting in persistent filling of aneurysm |
Endoleak type III | Stent graft laceration or fabric tears, dislodgment of modular graft devices |
Endoleak type IV | Porosity of the graft fabric, diffuse leakage through interstices |
Endotension | Excluded sac continues to enlarge without apparent endoleak formation |
Penetrating Aortic Injury
Penetrating injuries to the intrathoracic great vessels are uncommon, with an incidence of 1%, and a high mortality rate that ranges between 50% and 85% despite advances in trauma care and prehospital resuscitation. These types of injuries can be caused by gunshot wounds (GSWs) or stab wounds (SWs) that traverse the chest or the base of the neck (Fig. 11-9). Overall, GSWs are more common and more lethal than SWs. Penetrating injuries to the thoracic aorta are more common along the ascending aorta and the arch branches, with a documented low incidence of descending thoracic injuries. These types of lesions are commonly associated with coexisting lethal intrathoracic injuries, which worsen the patient’s prognosis. Although the aorta is protected by osseous structures, a laceration in this large-caliber vessel with high intraluminal pressure can cause rapid exsanguination. Thoracic aortic injuries have a worse prognosis than injuries to the abdominal aorta, probably due to the retroperitoneal location of the abdominal aorta, which can slow down exsanguination. They usually manifest as hemorrhage into the mediastinum or pleural cavity presenting as a hemothorax, cardiac tamponade, or mediastinal hematoma.
Nontraumatic Aneurysms of the Thoracic and Abdominal Aorta
Thoracic Aortic Aneurysms
Thoracic aortic aneurysms (TAAs) are often the result of atherosclerotic disease or cystic medial degeneration with subsequent weakening and dilatation of the aortic wall. It is usually a silent process, less commonly seen than abdominal aortic aneurysm, and generally occurs in older men in their sixth and seventh decades. Based on their morphology, aneurysms are classified as either fusiform aneurysms, which involve the full circumference of the vessel wall, or saccular aneurysms involving only a focal portion. Thoracic aneurysms occur in the ascending aorta in 40% to 60% of the cases, descending aorta in 30% to 40% of the cases, and aortic arch and thoracoabdominal aorta in 10% of the cases. The incidence and prevalence of thoracic aortic disease including TAA are steadily increasing due to the aging population and improved diagnostic techniques. The reported incidence of TAA ranges from 6 to 10 cases per 100,000 patients per year. The natural history of untreated TAA is progressive expansion of the aneurysm and ultimately rupture (Fig. 11-10).
Given that most patients are asymptomatic, TAAs are commonly diagnosed as an incidental finding on imaging studies. However, they can also present with signs and symptoms related to compression of adjacent structures. Ascending aortic aneurysms can compress the coronary arteries or the origin of the great vessels causing myocardial or cerebral ischemia; together with arch aneurysms, they can erode into the mediastinum and compress the left recurrent laryngeal nerve causing hoarseness, or the phrenic nerve and lead to hemidiaphragmatic paralysis. They can also press on the tracheobronchial tree causing wheezing, dyspnea, cough, hemoptysis, and pneumonitis; on the esophagus and cause dysphagia; and on the superior vena cava (SVC) causing SVC syndrome. Mural thrombus from the aneurysms can be the source of emboli and cause strokes, as well as renal, mesenteric, or limb ischemia. Heart failure can result from aortic root aneurysms leading to aortic regurgitation, or from the rupture of a sinus of Valsalva aneurysm into the right side of the heart. Chest and back pain are rare symptoms associated with compression of intrathoracic structures or erosion into adjacent bones (Fig. 11-11).
The most feared complications of TAA are aneurysm dissection and rupture. Dissection can lead to arterial occlusion and end-organ ischemia, while rupture can cause massive hemorrhage that usually cannot be contained by adjacent structures and therefore is considered a surgical emergency. Patients present with hypotension and acute onset of chest pain, abdominal pain, back pain, or neck pain. Rupture occurs more commonly into the left pleural space, but it can also occur into the pericardium causing pericardial tamponade (Fig. 11-12), and into the esophagus resulting in aortoesophageal fistula and dramatic upper gastrointestinal bleeding.
Multiple etiologies have been associated with thoracic aortic aneurysms, and they differ depending on their location within the thoracic aorta. Aneurysms of the ascending aorta and aortic arch are more commonly seen in elderly patients secondary to atherosclerosis or aortic valve stenosis leading to poststenotic dilatation. The younger patient population presenting with thoracic aneurysms in the ascending aorta and aortic arch (Tables 11-2 and 11-3) usually have a connective tissue disorder such as Marfan syndrome (mutation of fibrillin-1 gene), Ehlers-Danlos syndrome (defect in type III collagen), Loeys-Dietz syndrome, or Turner syndrome (associated to bicuspid aortic valves). Other infrequent etiologies include mycotic and syphilitic aneurysms. Aneurysms of the sinus of Valsalva can be congenital, infectious, or postsurgical in origin, and they are seen as dilatations in connection with the aortic root. Vasculitis can affect all portions of the thoracic aorta. Takayasu arteritis is one of the most striking entities, characterized by a chronic inflammatory process of unknown etiology that affects the aorta, its major branches, and the pulmonary arteries. It affects the vessel wall causing obliterative luminal changes, occlusion, or dilatation. Aneurysm formation can be a fatal complication of this disease since it may lead to heart failure secondary to aortic valve regurgitation or aortic rupture.
Degenerative | Associated with atherosclerosis |
Inheritable/connective tissue | Marfan syndrome |
Ehlers-Danlos syndrome | |
Loeys-Dietz syndrome | |
Turner syndrome | |
Osteogenesis imperfecta | |
Rheumatoid arthritis | |
Bicuspid aortic valve | |
Aneurysm of sinus of Valsalva | |
Arteritis | Giant cell arteritis |
Takayasu arteritis | |
Behcet’s disease | |
Relapsing polychondritis | |
Infectious | Syphilis |
Mycotic aneurysms |
Table 11-3 Aortic Arch Aneurysms
Degenerative | Secondary to atherosclerosis |
Arteritis | Giant cell |
Takayasu | |
Behcet’s disease | |
Infectious | Syphilis |
Thoracic aneurysms of the descending aorta are also most commonly degenerative in origin caused by atherosclerosis and usually associated with hypertension, hypercholesterolemia, and smoking. These aneurysms are generally fusiform with significant intimal calcifications and demonstrate associated tortuous aorta and mural thrombus. Other causes include chronic aortic dissection (Stanford type A or B), which can dilate over time as the wall of the false lumen weakens, creating a dissecting aneurysm with a high risk of rupture. Injuries to the descending thoracic aorta associated with blunt trauma can result in pseudoaneurysm formation, characteristically located near the aortic isthmus distal to the subclavian artery, and with a high risk of rupture (these are discussed in the TAI section). As with ascending thoracic aneurysms, younger patients with descending thoracic aortic aneurysms usually have an underlying connective tissue disorder (Table 11-4) or vasculitis. Mycotic aneurysms can also affect this segment.
Table 11-4 Descending Thoracic Aortic Aneurysm
Degenerative | Secondary to atherosclerosis |
Focal pseudoaneurysm secondary to penetrating aortic ulcer | |
Arteritis | Giant cell |
Takayasu | |
Behcet’s disease | |
Inherited/connective tissue | Marfan syndrome |
Ductus aneurysm | |
Loeys-Dietz syndrome | |
Infectious | Mycotic aneurysms |
Traumatic | Post-traumatic pseudoaneurysms |
Chronic aortic transection |
Aneurysms located in the thoracoabdominal aorta are less frequent than abdominal or thoracic aneurysms, which is fortunate because these are difficult-to-treat lesions due to the numerous visceral branches that arise along this segment. They are divided into four types according to the Crawford classification (Table 11-5). Types II and III are the more complex ones, with type II having the highest risk of treatment-induced spinal cord infarction and renal failure.
Table 11-5 Crawford Classification of Thoracoabdominal Aneurysms
I | Descending thoracic aorta to suprarenal aorta |
II | Proximal descending thoracic aorta to infrarenal aorta (below the diaphragm) |
III | Mid-descending thoracic aorta to infrarenal aorta |
IV | Supravisceral aorta to infrarenal aorta |
Diagnosis
CTA provides sufficient information to diagnose and follow up progression of TAA; angiographic sequences allow for precise delineation of the extension of the aneurysm, involvement of the great vessels, and other associated thoracic pathology (see Fig. 11-11). Unenhanced CT is used to identify areas of aortic calcification, mural thrombus, acute wall hematoma (circumferential or crescentic), and recent hemorrhage. Contrast administration helps to differentiate the patent lumen from mural thrombus and to demonstrate an intimal flap and a false lumen in areas of aortic dissection. CT also shows the relationship of the aneurysm to the adjacent structures and helps correlate associated patient symptomatology with imaging findings. Mycotic aneurysms can affect any portion of the aorta and are seen as saccular dilatations with multilobulated contour (Fig. 11-13). Cross-sectional CT imaging features include a perianeurysmal soft tissue mass, fluid collection, and occasionally gas-forming inflammation. Syphilitic aortic aneurysms are rarely seen nowadays, but if encountered they usually demonstrate extensive aortic calcification or linear calcifications with longitudinal wrinkling of the wall causing a “shaggy tree-bark” pattern; approximately 75% of the cases exhibit a saccular morphology.
Treatment
TAA repair depends on its location. Aneurysms located in the ascending aorta are usually treated surgically via a median sternotomy with aneurysm resection and prosthetic tube graft placement. Surgical treatment is recommended for patients who are symptomatic, present with large aneurysms, have a high aneurysmal growth rate, or have associated complications. The best time to treat patients with TAA is still uncertain given the limited understanding of its natural history. Nonetheless, for most ascending thoracic aneurysms, repair is considered in patients with aneurysms that are 5.5 cm in diameter or larger. The decision to intervene is made depending on the patient’s operative risks versus the risk of developing an aneurysm-related complication such as dissection or rupture (e.g., patients with Marfan syndrome have their aneurysms repaired earlier due to the high risk of dissection and valvular insufficiency). Mortality of elective surgical repair in ascending aortic aneurysms ranges from 3% to 5%, and it carries a high risk of postsurgical morbidity including paraplegia, stroke, and bleeding; however, successful repair practically eliminates the risk of rupture. Lesions that are not treated by a surgical approach can have a mortality rate as high as 74%. Repair of arch aneurysms is even more challenging due to the high risk of stroke during replacement of the abnormal arch and reimplantation of the brachiocephalic vessels. Elective surgical repair for descending thoracic and thoracoabdominal aortic aneurysms has an associated mortality rate of 5% to 14% and a significant risk of paraplegia due to occlusion of the spinal cord blood supply; the risk has decreased with the implementation of protective techniques such as regional epidural hypothermic protection and cerebrospinal fluid drainage. Indications for surgical repair of descending aortic aneurysms include diameter equal to or larger than 6.5 cm, a patent primary entry site, expanding false lumen of either a dissection or aneurysm, symptomatic patients, or signs of impending rupture (Box 11-3).
Endovascular treatment of aortic arch aneurysms requires surgical transposition of supra-aortic vessels and is currently used in selected cases to help reduce the risk inherent in surgical repair. Patients with descending thoracic aortic aneurysms can also be offered endograft repair as an alternative to surgical treatment, in particular those patients with high operative risk. Endografts successfully exclude the aneurysm sac in most patients, with an apparent lower rate of persistent neurologic deficits in the range of 2% to 3% (versus 5% to 6% for surgically treated patients). Nevertheless, stent grafts are also associated with complications such as stroke, paraplegia, and device-related complications such as endoleaks and graft migration. Long-term studies are on their way to assess the durability of endograft aneurysm repair (see Fig. 11-10).
Abdominal Aortic Aneurysms
Diagnosis
MD-CTA has emerged as the new gold standard for the diagnosis of aortic abdominal aneurysms, replacing catheter arteriography. MD-CTA offers high-resolution imaging and shorter scan times, allowing the detection and characterization of aneurysms and related complications including impending rupture and contained or complete rupture. Several signs of impending rupture have been identified (see Box 11-3) on CT including increased aneurysm size, a low thrombus-to-lumen ratio, and mural thrombus hemorrhage that is usually identified as high-attenuation crescents in the wall of the aortic aneurysm in unenhanced CT images (Fig. 11-14). There are various signs that suggest a contained AAA rupture, including loss of definition of the posterior aortic wall; presence of an organized hematoma with contour abnormalities of the vessel wall; interruption of a continuous ring of aortic wall calcification; and a posterior wall of the aorta following the contour of the vertebra with or without associated vertebral erosion (sign known as a “draped” aorta). A ruptured AAA is determined by the presence of hemorrhage contiguous to the aorta, almost always involving the retroperitoneal space and rarely the iliopsoas compartment. Periaortic blood might be seen in the pararenal space, perirenal space, or both; a hematocrit sign (cellular-fluid level) and rectus sheath bleeds are more rare but helpful if present, and tend to be associated with coagulopathic hemorrhage (Fig. 11-15).
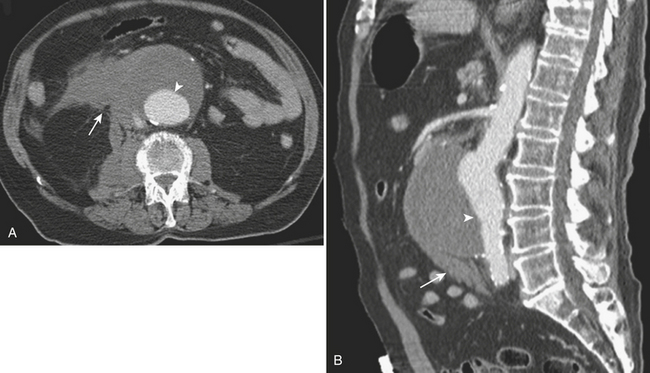
Figure 11-15 A and B, CTA images demonstrating a large infrarenal aortic aneurysm (arrowheads) with rupture (arrows).
(Courtesy of Dr. Daniel J.A. Margolis, UCLA, David Geffen School of Medicine.)
CT is also useful in differentiating between different types of aortic aneurysms. Mycotic aneurysms are usually seen as saccular-shaped collections with a lobulated contour; other features may include a periaortic soft tissue mass with stranding, retroperitoneal para-aortic fluid collection, vertebral erosion, gas-forming inflammation around the aneurysm, intra-aortic air pockets, and thrombus formation within a false lumen after aneurysmal rupture (see Fig. 11-13). In inflammatory aneurysms, CT and MRI can detect the cuff of soft tissue inflammation surrounding the aneurysm, thickening of the aneurysm wall, perianeurysmal and retroperitoneal fibrosis, and adherence of the anterior aneurysm wall to adjacent structures (Fig. 11-16). On precontrast CT images the thickened aortic wall has soft tissue attenuation that enhances after intravenous contrast administration. The arterial wall may become undistinguishable from periaortic fibrosis. Periaortic fibrotic tissue can adhere to the ureters, small bowel, duodenum, and inferior vena cava causing entrapment of these structures and further complicating surgical repair. Preoperative imaging is of great importance in the planning of surgical and endovascular treatment of patients with AAA. Important preoperative features and measurements done on CTA include the maximum transverse aneurysm diameter, relation of the aneurysm to the renal arteries, presence of a proximal neck (renals to aneurysm distance), presence of a distal neck (aneurysm to aortic bifurcation distance), extension of the aneurysm into the iliac arteries, identification of concomitant aneurysms, and appearance of the access pathway including femoral and iliac arteries. Some conditions that limit the delivery of an endograft include very large AAA, markedly tortuous aorta, pelvic arteries with abrupt angles, and extensive calcification causing luminal narrowing. Any of those factors can represent an exclusion criterion depending on the specific anatomy, and the degrees of angulation and stenosis. They determine the suitability or unsuitability for endovascular treatment, and cautious analysis of the images in conjunction with the surgical/interventional team is crucial to decrease the risk of endoleaks, attachment failures, graft migration, and conversion to open repairs. MRA has shown similar properties as CTA for the diagnosis of and for preoperative planning for AAA, providing precise information about the location and extent of the aneurysm. Conventional arteriography is currently used as an adjunct imaging modality for evaluating patients with complex vascular anatomy, for aneurysms that could not be fully characterized with CTA and MRA, and for intraprocedural graft positioning.