Chapter 51E Vascular Diseases of the Nervous System
Spinal Cord Vascular Disease
Vascular Anatomy of the Spinal Cord
The embryonic arterial supply to the spinal cord derives from intradural vessels that enter at each spinal level and divide to follow the dorsal and ventral roots. The ventral radicular branches join along the midline to form the anterior spinal artery. Irregular anastomoses among the dorsal roots, as they enter the cord on each side, form paired posterior spinal arteries. The anterior and posterior spinal arteries constitute longitudinal arterial plexuses. Circumflex vessels (arteria vasocorona) connect the anterior and posterior arterial systems in a pial plexus around the lateral margins of the cord (Fig. 51E.1).
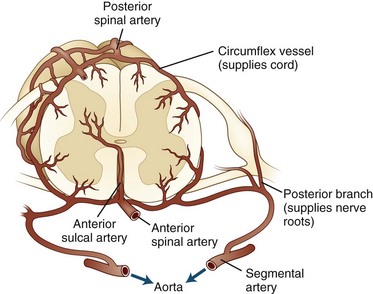
Fig. 51E.1 Arterial supply to the spinal cord and nerve roots at the level of a medullary radicular artery.
(Adapted from Henson, R.A., Parsons, M., 1967. Ischaemic lesions of the spinal cord: an illustrated review. Q J Med 36, 205-222.)
During development, a few radicular arteries become dominant and provide most of the flow to the spinal cord through the anterior spinal artery. These are variably known as medullary or radiculomedullary vessels. Unfortunately, the terms radicular, medullary, and radiculomedullary are often used interchangeably in the literature. Five to eight of these vessels typically persist in adults. The largest and most frequently identified of the anterior vessels is the arteria radicularis magna or great artery of Adamkiewicz, which courses along one of the lower thoracic or upper lumbar anterior roots to join the anterior spinal artery. It provides a major portion of the blood flow to the lower thoracic cord and the lumbar enlargement. The sacral cord, conus medullaris, and cauda equina are supplied by small lower segmental radicular arteries. In general, a larger number of smaller vessels serve the posterior spinal artery. The cervical and upper thoracic spinal cord is richly vascularized by a plexus arising from branches of the ascending cervical and vertebral arteries (Fig. 51E.2).
The main blood supply to spinal gray matter, as well as to anterior and lateral funiculi, is derived from anterior sulcal arteries. These arise from the anterior spinal artery in the midline and course into the ventral median fissure. Each anterior sulcal artery distributes blood to only the left or right half of the spinal cord. The greatest distance between sulcal arteries is in the thoracic segments; the vascularity is proportional to the numbers of neurons located throughout the cord at that level. The dorsal columns and extreme dorsal horns (approximately one-third of the cord cross-section) are supplied by penetrating branches from the posterior spinal arteries. The superficial white matter also receives blood flow via the circumflex anastomotic vessels. This arrangement leads to a clinically relevant border zone between the territories of the sulcal and superficial arterial distributions. As a result, many spinal cord infarctions do not follow the conventional boundaries between anterior and posterior arterial distributions (Ishizawa et al., 2005).
Spinal Cord Ischemia
Paraplegia complicating aortic surgery was recognized as early as 1825, though it was not attributed to spinal cord ischemia until the 1880s. By the early 20th century, cardiac embolism, atheromatous disease, and decompression sickness were also described as causes of paraplegia attributable to spinal cord ischemia. The actual prevalence of spinal cord infarction is unknown but is generally cited as representing 1% to 2% of all central neurovascular events and 5% to 8% of all acute myelopathies. The clinical presentation of spinal cord syndromes is presented in more detail in Chapter 24.
Presentation and Initial Course
Weakness (100%), sensory loss (89%), back pain at onset (82%), and urinary complaints requiring catheterization (75%) were the most common symptoms of cord ischemia at the time of presentation in a prospectively collected series (Masson et al., 2004). In retrospective series, the same major symptoms are observed, though with minor differences in observed frequency (Kumral et al., 2010; Nedeltchev et al., 2004; Novy et al., 2006). The most common location to be affected is the mid- to low thoracic spine. Lower cervical lesions are less common, and upper thoracic spinal infarcts are rare. Quadriparesis is present in only 20% to 25% of all cases of spinal infarction.
Pain and sensory changes occur first in most cases, followed by weakness within minutes or hours. Over 80% of the back pain with spinal infarction follows a radicular pattern (Novy et al., 2006), but in cases of acute aortic disease, pain may have a more visceral character. Maximum motor disability is observed within 12 hours of onset in a majority of patients, with a trend toward longer intervals when dysfunction was less severe. Urinary retention is typical in the acute phase, but involuntary voiding or defecation may be associated with the onset of the ischemic insult.
Investigations
Magnetic resonance imaging (MRI) is the diagnostic procedure of choice for detecting spinal cord ischemia, although the results can be normal in up to one-third of patients (Novy et al., 2006). In the acute phase of spinal cord infarction, high diffusion-weighted imaging signal is noted and matches with low apparent diffusion coefficient signal (Thurnher and Bammer, 2006). Pencil-shaped hyperintense signal on T2-weighted images initially appear within 2 hours to several days, and may be accompanied by gadolinium enhancement (Weidauer et al., 2002). Abnormal signal and contrast enhancement may demonstrate a double-dot (“owl’s eyes”) pattern in the region of the anterior horns, an H-shaped pattern involving the central gray matter, or a more diffuse pattern involving both gray and white matter (Fig. 51E.3). The diffuse pattern may be difficult to distinguish from venous infarction. When cord infarction results from compromise of a segmental artery, branches supplying the ipsilateral half of the vertebral body may also be affected. Vertebral body infarct is best detected on sagittal T2-weighted images, usually appearing as a triangular area of increased signal near the end-plate and/or deep medullary portion of the vertebral body.
Other laboratory and radiographic studies are not diagnostic in noncompressive spinal cord ischemia. Myelography is usually normal. Cerebrospinal fluid (CSF) protein was elevated in 44% of one series, but pleocytosis was not observed (Novy et al., 2006).
The exact cause of spinal ischemia often remains occult and could not be discerned in up to half of prospective and retrospective series (Masson et al., 2004; Novy et al., 2006).
Course
The course of spinal ischemic syndromes is variable. Transient ischemic attacks have been reported to precede up to 10% of cord infarcts (Kumral et al., 2010; Novy et al., 2006). Pain is often persistent and is a major contributor to long-term disability after spinal cord infarction. A slowly progressive myelopathy attributed to chronic constriction of radiculomedullary arteries in the neck has been suggested but not established.
Prognosis
Rates of recovery vary widely among case series, which were collected by different methods. In a prospectively collected series, approximately half of patients had a favorable outcome, defined as the ability to walk with one assistive device (or none) and no need for urinary catheterization at the time of hospital discharge (Masson et al., 2004). Recent retrospective series also suggest that ambulation can be restored in about 50% of patients (Kumral et al., 2010; Novy et al., 2006). Likelihood of recovery is higher when the deficits are less severe at presentation. Over a mean follow-up period of 4 years, more than 90% of patients with the mildest severity of deficit during the acute phase were able to walk independently or with assistive devices; in contrast, nearly one-third of more severely affected patients required a wheelchair (Nedeltchev et al., 2004