19 Valvular Heart Disease
Replacement and Repair
In analyzing the physiologic response of the left ventricle (LV) to a variety of abnormal loading conditions, it is useful to consider the concepts of afterload mismatch and preload reserve.1 These concepts frame discussions of the pathophysiology of the individual valvular lesions. Another set of physiologic concepts essential to understanding VHD and its evolution are pressure-volume loops and the linear end-systolic pressure-volume relation (ESPVR). The former provides a graphic analysis of ventricular pressure-volume relations in a single beat, whereas the latter is a method for quantifying the intrinsic contractility of the myocardium over multiple contractions and is relatively independent of changes in loading conditions (see Chapters 5, 12, 13 and 14).
Pathophysiology

Pressure-Volume Loops
The pressure and volume changes that occur during a normal cardiac cycle are depicted in Figure 19-1. A pressure-volume loop is generated when ventricular volumes are plotted against simultaneously occurring ventricular pressures for a single contraction (Figure 19-2). Familiarity with this graphic analysis of cardiac function, the normal pressure-volume loop of the LV, provides a basis for appreciating a load-insensitive index of contractility, the ESPVR. Phase 1 in Figure 19-2 shows ventricular filling and represents the loading of the heart before contraction (i.e., preload). During early and middle diastole, filling of the ventricle is rapid, depending on the pressure gradient between the left atrium and the LV. In late diastole, the left atrium contracts (a wave), which results in the final left ventricular end-diastolic volume (LVEDV) and pressure (LVEDP). Atrial contraction in the normal heart accounts for 15% to 20% of ventricular filling. The normal ventricle accommodates large changes in ventricular volume with only a small change in ventricular diastolic pressure.
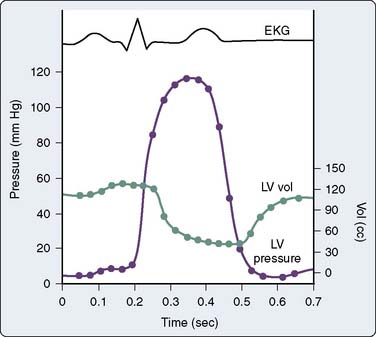
Figure 19-1 Simultaneous left ventricular (LV) volume and pressure during one cardiac cycle. EKG, electrocardiogram.
(From Barash PG, Kopriva DJ: Cardiac pump function and how to monitor it. In Thomas SJ [ed]: Manual of cardiac anesthesia. New York: Churchill Livingstone, 1984, p 1.)

Afterload Stress and Preload Reserve
Figure 19-3 shows the response of the LV to changes in afterload, with preload held constant, in the context of the pressure-volume loops from single contractions. These curves are constructed experimentally by infusing a pure α-adrenergic agonist while simultaneously measuring the corresponding end-systolic volumes (ESVs). The resultant curves describe the diastolic pressure-volume relation (ventricular compliance) and the linear ESPVR at a given level of myocardial contractility. Each counterclockwise loop represents a cardiac cycle. The stroke volume (SV) progressively decreases as the impedance to ejection increases from beats 1 to 3. This pattern continues until beat 4, when the peak ventricular systolic pressure fails to open the aortic valve and only isovolumic contraction ensues.1 This inverse relation between afterload and SV (inverse force-velocity relation) also was documented experimentally in a canine preparation in which the LVEDV was held constant (Figure 19-4).2 In the intact heart, afterload is a function of ventricular size and arterial pressure.3 Its pivotal role in cardiovascular regulation is summarized in Figure 19-5. Afterload is defined as the tension, or force per unit of cross-sectional area, in the ventricular wall during ejection.4 Laplace’s law provides a mathematical expression for the wall tension5 (in which P is the intraventricular pressure) developed in a spherical chamber of radius, R, and wall thickness, h:
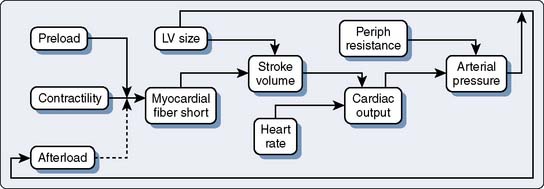
Figure 19-5 Role of afterload in cardiac regulation.
LV, left ventricular; Periph, peripheral.
(From Braunwald E: Regulation of the circulation. N Engl J Med 290:1124, 1974.)
This means that ventricular afterload, a function of the constantly changing intraventricular pressure and radius, varies continuously during systole. It is extremely difficult to precisely quantify afterload in the clinical setting, and commonly used approximations such as blood pressure or systemic vascular resistance (SVR) are inherently misleading because they fail to reflect instantaneous pressure-volume variations. Left ventricular size or preload is a determinant of the SV and afterload.6 Normally, the LV compensates for increases in afterload by increases in preload. This increase in end-diastolic fiber stretch or radius further increases wall tension in accordance with Laplace’s law, resulting in a reciprocal decline in myocardial fiber shortening (i.e., inverse force-velocity relation). Despite this relative decline in fiber shortening, the SV is maintained because contractile force is augmented at the higher preload (i.e., Starling or length-active tension effect). This increased contractility at higher LVEDVs may be mediated by an increased sensitivity to the inotropic effects of extracellular calcium at longer muscle lengths.7–9
Use of this “preload reserve” allows the LV to maintain its SV in the face of an afterload stress,1 as shown in Figure 19-6.1 The increase in afterload (beat 2) elicits a compensatory increase in end-diastolic volume (EDV; beat 3), preserving SV at the higher afterload. However, when the ventricle reaches the limit of its preload reserve, it overdistends, and preload behaves as if it were fixed. SV then decreases with further increases in the systolic pressure (afterload mismatch; beat 4), a clinical corollary of the inverse force-velocity relation.

Ventricular Compliance
Translating this physiologic analysis to the clinical setting is complicated by a number of practical constraints. One of the most important is the inconstant relation between LVEDV and LVEDP. The diastolic pressure-volume relation for the normal mammalian LV is a curvilinear function (Figure 19-7).3,10 The slope of this curve (i.e., ratio of change in volume to change in pressure during diastole) is the ventricular compliance (dV/dP). Although the normal ventricle is extremely compliant in the physiologic range, the instantaneous compliance decreases with increments in diastolic filling. This progressively increasing slope of the pressure-volume curve becomes evident at the extremes of ventricular volume, in which succeeding increments in volume result in exponentially greater increases in end-diastolic pressure (EDP). Patients with acute aortic regurgitation (AR) experience the hemodynamic consequences of this phenomenon. The catastrophic increases in ventricular filling pressure reflect the absolute magnitude of the volume overload and are a corollary of a precipitously declining compliance relation.
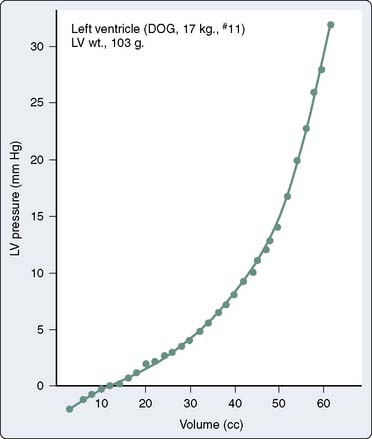
Figure 19-7 The normal diastolic pressure-volume relation.
(From Spotnitz HM, Sonnenblick EH, Spiro D: Relation of ultrastructure to function in the intact heart: Sarcomere structure relative to pressure-volume curves of the intact left ventricles of dog and cat. Circ Res 18:49, 1966.)
These hemodynamic manifestations of acute shifts up and down a single compliance curve must be distinguished from chronic, pathologic alterations in ventricular compliance, which produce actual shifts of the entire curve relating diastolic pressure and volume. For example, in animal models of chronic volume overload, the entire pressure-volume curve is shifted to the right, such that substantial increases in ventricular volume are tolerated with relatively little change in EDP (Figure 19-8).11 In this example, the slope of the new pressure-volume curve (i.e., compliance) is decreased. Similarly, time-dependent, rightward shifts of the entire pressure-volume relation have been shown to occur in patients with severe ventricular volume overload resulting from chronic AR.12 In these examples, the development of a new relation between pressure and volume may reflect the physiologic process of creep, the time-dependent change in size or the dimension of tissue maintained at a constant level of stress.4
Myocardial wall thickness is an important determinant of diastolic compliance. In clinical settings of chronic pressure overload (e.g., aortic stenosis [AS], chronic hypertension), diastolic compliance and ventricular wall thickness have been shown to be linearly and inversely related (Figure 19-9).13 This may explain why the normal, thinner-walled right ventricle (RV) is more compliant than the normal LV, even though the ventricles share similar qualities of intrinsic myocardial stiffness.3,14 This association between pathologic hypertrophy of the ventricle and deterioration in its diastolic compliance is a well-documented but poorly understood phenomenon.
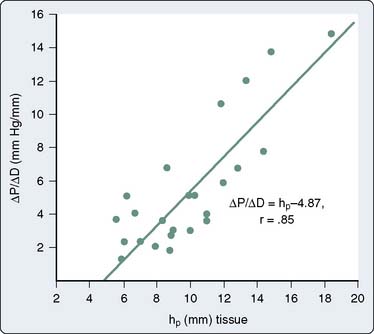
Figure 19-9 Relation between chamber stiffness (ΔP/ΔD, the inverse of compliance) and ventricular wall thickness.
(From Grossman W, McLaurin P, Moos SP, et al: Wall thickness and diastolic properties of the left ventricle. Circulation 49:129, 1974.)
It has become customary to characterize diastolic function in certain disease states as normal or abnormal, such as diastolic dysfunction or diastolic failure.15,16 The latter condition is a distinct pathophysiologic entity that results from an increased resistance to ventricular filling and leads to an inappropriate upward shift of the diastolic pressure-volume relation.17 For example, diastolic dysfunction is seen in ischemic cardiomyopathy, particularly when combined with pressure overload hypertrophy.18
In certain diseases, these primary derangements of diastolic function may predominate over abnormalities of diastolic function associated with ventricular hypertrophy. For example, in hypertrophic cardiomyopathy (HCM), the impaired ventricular relaxation inherent in the myopathic process appears to play the greater role in the observed abnormalities of diastolic filling because the diastolic dysfunction is often disproportionate to the degree of ventricular hypertrophy.19,20 The effects of pathologic hypertrophy on diastolic compliance and ventricular relaxation are complex and are considered in more detail later in the Aortic Stenosis and Hypertrophic Cardiomyopathy sections.
Filling of one ventricle or changes in its configuration or compliance properties can significantly alter the diastolic pressure-volume characteristics of the other ventricle.21,22 Progressive increases in right ventricular filling shift the left ventricular compliance curve up and to the left. This effect is greatest at high right ventricular filling pressures and is accentuated by the presence of the pericardium.23 With severe right ventricular distention, the interventricular septum encroaches on the LV. This encroachment reduces the size of the LV and alters its geometric configuration such that its compliance declines.21 As a result, left ventricular filling pressures may fail to reflect even directional changes in left ventricular size.24

Contractility
Myocardial contractility can be defined as the ability of the heart to generate force at a given preload.25 Although most clinicians and researchers seem to be comfortable with their intuitive notions of the cardiac inotropic state, the search for a consensus on a quantitative yardstick of ventricular inotropy has proved to be elusive. Its accurate and reproducible measurement is of more than theoretic interest because the contractile function of the heart is a key determinant of prognosis for most cardiac diseases, and it is especially important in critical decisions regarding the timing of surgical correction in patients with VHD.
Historically, methods of assessing myocardial contractility have been divided into two groups, based on analysis of the isovolumetric or the ejection phase of cardiac contraction.4 Details of their clinical determination and relative reproducibility are beyond the scope of this discussion, but more information can be found in several excellent reviews,4,26,27 as well as in Chapters 5 and 14. The isovolumetric indices include measurements such as maximal velocity of myocardial fiber shortening (Vmax), peak pressure development (dP/dt), and peak dP/dt measured at an instantaneous pressure (dP/dt/P). Although relatively insensitive to loading conditions, these tests poorly reflect basal levels of contractility and are unreliable for comparing contractility among patients or assessing directional changes in contractility in an individual patient over time.26
Ejection phase indices, such as the ejection fraction (EF), are determined, in part, by the intrinsic inotropic state and can be used to define basal levels of contractility.26 Such measurements are extremely useful in evaluating ventricular function in patients with CAD or other conditions that do not significantly alter ventricular loading conditions.28 Ejection phase indices are understandably popular because they are readily available, and they are the most widely used clinical measures of left ventricular function. However, these indices are directly proportional to preload, vary inversely with ventricular afterload, and are accordingly unreliable for assessing contractile performance in patients with most forms of VHD.
The use of the pressure-volume diagram and analysis of the ESPVR allow a more precise appreciation of left ventricular contractility, which is independent of preload.29 The extent of myocardial shortening, and therefore of end-systolic fiber length, is a direct function of afterload (i.e., inverse force-velocity relation), and myocardial contractility can be evaluated by making use of this fundamental property. In most instances, end-systolic pressure (ESP) can be substituted for afterload. Only with pathologic degrees of ventricular hypertrophy is there a major divergence between ESP and afterload. This means that for any level of contractility, the ESV to which a ventricle contracts is a linearly increasing function of ESP. A stronger ventricle contracts to a smaller ESV (i.e., empties more completely) at any given level of ventricular afterload. Changes in the inotropic state also can be viewed in the context of the idealized pressure-volume loop. Positive inotropic interventions shift the curve up and to the left, increasing the work that can be performed at any given EDV (preload). Conversely, negative inotropic interventions shift the curve down and to the right30 (Figure 19-10; see Chapters 5 and 14). These load-independent indices of contractility are largely research tools, but in the future, it may be possible to construct pressure-volume loops and quantify ESPVRs in real time with echocardiographic equipment featuring automated border detection31 (see Chapter 12).
Clinical studies of patients with relatively normal loading conditions have shown that variations in the ESV reliably correlate with changes in ejection phase indices (Figure 19-11).32 The ESPVR represents an index of contractility that depends on systolic ventricular pressure (i.e., afterload) but is independent of end-diastolic length (i.e., preload).28,33,34 Pressure-volume loops also provide a framework for considering the interactions between systolic (inotropic) and diastolic (lusitropic) function. Although the preload (i.e., EDV) is an independent determinant of SV, because of the circular nature of blood flow, the SV ultimately determines venous return and the resultant preload for the next cardiac cycle.30 The “inotropically determined” ESV is the other element besides the venous return that contributes to the EDV. Just as the ESPVR uniquely describes systolic function, the end-diastolic pressure-volume relation is a manifestation of the intrinsic relaxation (lusitropic) properties of the ventricle. Positive lusitropic interventions facilitate ventricular filling and shift the end-diastolic pressure-volume relation down and to the right, and a negative lusitropic intervention shifts it up and to the left (Figure 19-12).
Aortic stenosis

Clinical Features and Natural History
Aortic stenosis (AS) is the most common cardiac valve lesion in the United States. Approximately 1% to 2% of the population is born with a bicuspid aortic valve, which is prone to stenosis with aging, and the population is aging. Clinically significant aortic valve stenosis is present in 2% of unselected individuals older than 65 years, and in 5.5% of those older than 85 years.35 Incipient aortic valve stenosis with calcification and stiffening is observed in 50% of people in the 75 to 80 age group and in up to 75% in those older than 85 years. Bicuspid aortic valve is a common congenital cardiac abnormality, occurring in approximately 1% of the population.36 The heritability coefficient is estimated at 89%, suggesting that the bicuspid aortic valve is almost entirely genetic in nature.37 It is a risk factor for premature AS and ascending aortic aneurysms. The ascending aorta in bicuspid valvular disease has the same histopathologic features as Marfan syndrome, such as medial degeneration, decreased fibrillin-1, and enhanced matrix metalloproteinase activity in the aortic wall. Recent data from the International Registry of Acute Aortic Dissection demonstrate that patients with bicuspid aortic valve have a 6.14% lifetime risk rate of aortic dissection (nine times that of the general population), compared with 40% for patients with Marfan syndrome. Yet, bicuspid aortic valvular disease is a hundred times more common than Marfan syndrome and is, therefore, responsible for a larger number of aortic dissections.36 Calcific AS has several features in common with CAD. Both conditions are more common in men, older people, and patients with hypercholesterolemia, and both result, in part, from an active inflammatory process. There is clinical evidence of an atherosclerotic hypothesis for the cellular mechanism of aortic valve stenosis. There is a clear association between clinical risk factors for atherosclerosis and the development of AS: increased lipoprotein levels, increased low-density lipoprotein (LDL) cholesterol, cigarette smoking, hypertension, diabetes mellitus, increased serum calcium and creatinine levels, and male sex.38 Homozygous familial hypercholesterolemia produces a severe form of AS in children.39 The early lesion of aortic valve sclerosis may be associated with CAD and vascular atherosclerosis. The extent of aortic valve calcification was observed as an important predictor of poor outcome in patients with AS.40 These and other studies suggest that aortic valve calcification is an inflammatory process promoted by atherosclerotic risk factors.
The early lesion of AS resembles that of the initial plaque of CAD, and there is a close correlation between calcification found in coronary arteries and the amount of calcification found in the aortic valve. Pathologic studies of aortic valves revealed the presence of LDL, suggesting a common cellular mechanism for the genesis of valvular and vascular atherosclerotic disease.38 Degenerative lesions on aortic valves contain an inflammatory infiltrate of nonfoam cells and foam cell macrophages, T lymphocytes, and other inflammatory cells. Lipid is accumulated in the fibrosa immediately below the endothelium layer on the aortic side of the valve. LDL- and apolipoprotein E–containing lipoproteins are present as well. Little is known about the synthesis of bone matrix proteins in calcific aortic valve stenosis. The calcifications are composed of hydroxyapatite on a matrix of collagen, osteopontin, and other bone matrix proteins.
A potential mechanism for this cellular inflammation process involves the combination of macrophages, LDL, and the secretion by macrophages of transforming growth factor-β and platelet-derived growth factor to stimulate the conversion of valvular fibroblast cells into osteoblasts that produce bone proteins.38 Most surprising are the findings that 3-hydroxy-3-methylglutaryl coenzyme A reductase inhibitors (e.g., statins) retard the progression of CAD and AS.41,42 The odds ratio of AS progression was 0.46 in 38 treated patients compared with 118 untreated patients.43 Other studies have shown similar results, and it is possible that therapy with statins or other drugs may be used to block or slow the progression of valve lesions in the future.38,44 In addition to recruitment of inflammatory cells and lipid accumulation into valves, calcification is a prominent feature that contributes to leaflet thickness and rigidity. Early development of aortic valve calcification may be related to vitamin D–receptor genotypes or mutations in the Notch gene.35 Calcification is an active process that includes osteopontin, osteonectin, osteocalcin, and other bone morphogenic proteins that regulate calcification and ossification. Active osteoblastic bone formation and osteoclastic bone resorption occur in stenotic valves. Increased fibroblasts produce collagen, leading to fibrosis. Concomitant elastin degradation also contributes to valve stiffening. Whereas normal valves are avascular, microvessels form in thickened stenotic valves, assuring the continued supply of inflammatory cells and lipids. Recent advances in genetic epidemiology have demonstrated a strong inheritability of bicuspid aortic valve with three loci on chromosomes 18q, 5q, and 13q, which likely contain genes responsible for bicuspid aortic valve.37 The mode of inheritance of other valvular diseases is unclear. No inheritability or inheritance studies have been reported for calcific aortic valve disease. In smaller genetic studies, a small number of candidate genes such as VDR, APOE, APOB, IL-10, and ESR1 have been identified as possibly playing a role in aortic valve disease. A recent breakthrough in the genetics of aortic valve disease was the identification of the Notch1 signaling pathway, involved in embryonic patterning, and that is highly expressed within the developing embryonic aortic valve. Notch1 is a repressor of Runx2, a critical regulator of osteoblast development. This supports the concept that a developmental program might be reactivated in disease processes.37
The rate of progression is, on average, a decrease in aortic valve area (AVA) of 0.1 cm2/year, and the peak instantaneous gradient increases by 10 mm Hg/year.45 The rate of progression of AS in men older than 60 is faster than in women, and it is faster in women older than 75 than in women 60 to 74 years old.46 Treatment with hemodialysis, the use of calcium supplements, and increased serum creatinine levels correlated with rapid progression of AS.47 Plasma brain natriuretic peptide, produced to a large extent by the ventricles, and the N-terminal part of the propeptide may serve as early markers of left ventricular hypertrophy (LVH), whereas atrial natriuretic peptide and the N-terminal part of the propeptide reflect atrial pressure increase.48 Repeated measurements of this marker may reflect information on the stage of AS and its hemodynamic impact.49
Angina, syncope, and congestive heart failure (CHF) are the classic symptoms of the disease, and their appearance is of serious prognostic significance because postmortem studies indicate that symptomatic AS is associated with a life expectancy of only 2 to 5 years.50–52 These early natural history studies were completed before the availability of cardiac catheterization studies, and some patients, although symptomatic, may have had objectively less severe degrees of stenosis. The “hemodynamically insignificant murmur of aortic stenosis” seen so often in cardiologic consultations does not portend such dire consequences, although it is sometimes difficult to correlate AS severity with clinical symptoms. There is evidence that patients with moderate AS (i.e., valve areas of 0.7 to 1.2 cm2) are also at increased risk for the development of complications, with the appearance of symptoms further increasing their risk.53 According to recent American College of Cardiology (ACC) and American Heart Association (AHA) guidelines, peak velocity greater than 4 m/sec, a mean gradient greater than 40 mm Hg, and a valve area less than 1.0 cm2 are considered hemodynamically severe AS.54 Aortic valve surgery should be performed promptly in symptomatic patients. In asymptomatic patients, high aortic valve calcium content and a positive exercise test are features that suggest a benefit from early aortic valve replacement (AVR).
An interesting question is whether the natural history of AS has changed significantly in recent years.55 The question is prompted by two trends. The first, at least in North America, is related to the steadily diminishing number of patients with rheumatic disease. Today, AS is essentially the bicuspid, the calcific, or what has been traditionally referred to as the senile variety. The second trend is related to the senile attribution. People are living longer, particularly those with heart disease. The typical patient with AS is older and much more likely to have other significant medical problems, including major coexisting cardiac disease, most often CAD.
Angina is a frequent and classic symptom of the disease, occurring in approximately two thirds of patients with critical AS, and about half of symptomatic patients are found to have anatomically significant CAD.56–58 However, a controversy persists about the incidence of CAD in patients with AS who do not have angina, and the controversy probably reflects this underlying sense of a change in the natural history of the disease. Some studies report that the absence of angina virtually excludes the possibility of atherosclerotic heart disease.59,60 In contrast, a 25% incidence rate of angiographically significant (> 70% obstruction) coronary occlusions was described in angina-free patients, most of whom had single-vessel disease.61 However, a much larger study found that 14% of patients with triple-vessel or left main CAD and AS presented without angina.62 Underscoring the continued lack of a consensus on this topic is the finding of a review that the reported incidence rates of significant CAD in asymptomatic patients range from 0% to 33%.63 Identification of asymptomatic patients is important because although coexistent, untreated (i.e., unbypassed) CAD has a detrimental effect on early and late survival after AVR, concomitant CABG with AVR does not increase perioperative mortality.64–67 It appears that a substantial number of patients with coexisting CAD may have symptoms other than chest pain.68 However, there do not appear to be other significant differences in the clinical or hemodynamic findings between patients with normal coronary arteries and those with coexistent coronary stenoses.
If the traditional natural history of AS is perhaps more ominous in the elderly patient with likely coexistent CAD, is there any rationale for prophylactic AVR? With steady improvement in surgical results, this question has been raised, although rigorous studies still suggest that asymptomatic patients with hemodynamically significant disease face a very low risk for sudden death before the onset of symptoms.69–71 As if by way of settling this particular question, an editorial by Braunwald72 stated that there was no role for prophylactic AVR, and that “operative treatment is the most common cause of sudden death in asymptomatic patients with aortic stenosis.” These patients warrant careful follow-up, and the case against prophylactic surgical intervention is further supported by studies showing that ventricular function is preserved and myocardial hypertrophy regresses after successful valve replacement.73–75
It is probably never too late to operate on patients with symptomatic AS.76,77 First, unlike AR, most symptomatic patients undergo valve replacement when left ventricular function is still normal. Second, even when impaired left ventricular function develops in AS, the relief of pressure overload almost always restores normal function or at least produces considerable improvement. Morbidity rates, mortality rates, and clinical results are favorable even in the oldest surgical candidates.78,79 Recent advances in operative techniques and perioperative management have contributed to excellent results after AVR in patients 80 years of age or older, with minimal incremental postoperative morbidity.80 The principal postoperative complication is respiratory failure.
The preoperative assessment of AS with Doppler echocardiography includes measurement of the AVA and the transvalvular pressure gradient.81,82 The latter is calculated from the Doppler-quantified transvalvular velocity of blood flow, which is increased in the presence of AS. This maximal velocity (v) is then inserted into the modified Bernoulli equation to determine the pressure gradient (PG) between the LV and the aorta (see Figure 19-2):
The pressure gradient is the maximal difference between the LV and aortic pressures that occurs during ventricular systole.83,84 This maximal instantaneous gradient is not the same as the peak-to-peak gradient determined by cardiac catheterization. The peak-to-peak gradient is determined by separate measurements of events that are not synchronous in real time. Of more practical interest is the fact that the best estimate of obstruction severity, as determined from pressure data alone, is the mean systolic gradient, which is calculated online by Doppler equipment85 (see Chapters 3 and 12).
The Doppler-calculated gradient is subject to the same flow limitation as invasively calculated gradients. Best understood by considering the extreme, this means that a patient with true end-stage AS would exhibit a relatively low calculated gradient because of minimal flow across a critically narrowed valve. In part because of this flow dependency, pressure gradients determined invasively or by Doppler echocardiography correctly classify AS severity in less than 50% of cases compared with estimates of AVA.86 However, the latter also can be determined by Doppler echocardiographic techniques. The preferred method requires only two Doppler-generated velocities: those proximal and those distal to the stenotic valve. These values are inserted into the continuity equation, which relates the respective velocities and cross-sectional areas proximal and distal to a stenotic area (see Figure 19-3). Specifically, the equation states:
in which AVA is the aortic valve area, V is the volume, and LVOT is the left ventricular outflow tract. Several studies have demonstrated the reliability of these Doppler-determined valve areas.83–86
Although advances in Doppler technology allow completely noninvasive evaluation of a large number of patients, coronary angiography is probably indicated in all patients older than 50 years who are demonstrated to have significant AS. Angiography and, if indicated, CABG may improve the long-term outlook for patients with CAD who undergo AVR.62 Angiography also can identify a smaller number of patients whose CAD alone would warrant CABG.

Pathophysiology
The normal AVA is 2.6 to 3.5 cm2, with hemodynamically significant obstruction usually occurring at cross-sectional valve areas of 1 cm2 or less. Generally accepted criteria for critical outflow obstruction include a systolic pressure gradient greater than 50 mm Hg, with a normal cardiac output, and an AVA of less than 0.4 cm2. In view of the ominous natural history of severe AS (AVA < 0.7 cm2),55,87 symptomatic patients with this degree of AS are generally referred for immediate AVR.88 The Hakki equation is a simplification of the Gorlin equation to calculate the AVA based on the cardiac output (CO) and the peak pressure gradient (PG) across the valve.
in which P is the intraventricular pressure, R is the inner radius, and h is the wall thickness.
This increase of wall tension is believed to be the direct stimulus for the further parallel replication of sarcomeres, which produces the concentrically hypertrophied ventricle characteristic of chronic pressure overload.89,90 The consequences of this LVH include alterations in diastolic compliance, potential imbalances in the myocardial oxygen supply and demand relationship, and possible deterioration of the intrinsic contractile performance of the myocardium.
Figure 19-13 shows a typical pressure-volume loop for a patient with AS. Two differences from the normal curve are immediately apparent. First, the peak pressure generated during systole is much greater because of the high transvalvular pressure gradient. Second, the slope of the diastolic limb is steeper, reflecting the reduced left ventricular diastolic compliance that is associated with the increase in chamber thickness.13 Clinically, this means that small changes in diastolic volume produce relatively large increases in ventricular filling pressure.
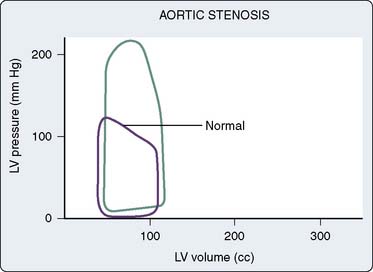
Figure 19-13 Pressure-volume loop in aortic stenosis.
(From Jackson JM, Thomas SJ, Lowenstein E: Anesthetic management of patients with valvular heart disease. Semin Anesth 1:239, 1982.)
This increased chamber stiffness places a premium on the contribution of atrial systole to ventricular filling, which in patients with AS may account for up to 40% of the LVEDV, rather than the 15% to 20% characteristic of the normal LV. Echocardiographic and radionuclide studies have documented that diastolic filling and ventricular relaxation are abnormal in patients with hypertrophy from a variety of causes, with significant prolongation of the isovolumic relaxation period being the most characteristic finding.91–94 This necessarily compromises the duration and amount of filling achieved during the early rapid diastolic filling phase and increases the relative contribution of atrial contraction to overall diastolic filling (Figure 19-14). A much greater mean left atrial (LA) pressure is necessary to distend the LV in the absence of the sinus mechanism. One treatment of junctional rhythm is volume infusion.
The systolic limb of the pressure-volume loop shows preservation of pump function, as evidenced by maintenance of the SV and EF (see Figure 19-13). It is likely that use of preload reserve and adequate LVH are the principal compensatory mechanisms that maintain forward flow. Clinical studies have confirmed that ejection performance is preserved at the expense of myocardial hypertrophy, and the adequacy of the hypertrophic response has been related to the degree to which it achieves normalization of wall stress, in accordance with the Laplace relation.95–97 LVH can be viewed as a compensatory physiologic response that completes a negative feedback loop (Figure 19-15). It is possible, however, that severe afterload stress and proportionately massive LVH could decrease subendocardial perfusion and superimpose a component of ischemic contractile dysfunction.
In patients with AS, LVH develops as a result of the increase in pressure load. The development of LVH and its regression after therapeutic interventions are accompanied by changes in the cardiac extracellular matrix guided by an increase in ECM1 gene expression during LVH and complete regression after complete correction.98
Systemic hypertension and AS represent an increase in afterload to the LV, and each contributes to left ventricular remodeling and LVH. In a large series of 193 patients with AS, 62 of whom were hypertensive, symptoms manifested with larger AVAs and lower stroke work loss.99 Patterns of left ventricular remodeling (i.e., concentric vs. eccentric remodeling and hypertrophy) were not different between hypertensive and normotensive patients. Left ventricular mass decreased by 23% 1 year after AVR, back into the normal range, in patients with normal preoperative ventricular function; diastolic function improved concomitantly. Improvements in myocardial blood flow and coronary vasodilator reserve after AVRs result from reduced extravascular compression and increased diastolic perfusion time.100–102
Ejection phase indices of contractile function are abnormal in many patients with AS.103,104 However, indices of contractile function, which are exquisitely sensitive to afterload, are inherently unreliable for quantitating the inotropic state in a disease such as AS, in which the essence of the hemodynamic insult is the severely increased ventricular afterload. This in no way excludes the possibility that a subset of patients also may experience some intrinsic depression of myocardial contractility. For example, patients with AS may be particularly at risk for superimposed, ischemic ventricular dysfunction, but this possibility can be assessed only by the application of load-insensitive measurements of contractile function.
In most patients, however, load-insensitive indices of contractility (i.e., end-systolic stress-diameter determinations) are virtually identical before and after the development of hypertrophy, suggesting that the increase in chamber thickness compensates for the afterload stress, and myocardial contractility is, therefore, normal, albeit appropriate for the higher afterload. Figure 19-16 illustrates the adaptation of the LV to chronic pressure overload. Figure 19-16A shows the pressure-volume relation before (loop A) and after (loop B) the development of concentric hypertrophy. The linear ESPVR is shifted up and to the left after concentric hypertrophy occurs. However, the ESPVR may not be as accurate or load insensitive in assessing contractility at the extremes of afterload. Figure 19-16B shows normalization of this apparently supranormal contractility, when wall stress (rather than pressure) is used as a more exact measure of ventricular afterload. Concentric hypertrophy normalizes wall stress, and contractility remains unchanged. A decline in any index of myocardial contractility in patients with AS may represent relatively inadequate hypertrophy for the degree of wall stress, some intrinsic depression of contractility, or a combination of these two factors.56,105,106 The fact that most patients have normal contractility is the reason for the usually favorable response to AVR.
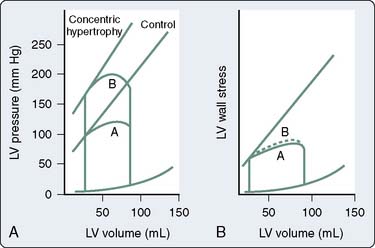
Figure 19-16 Adaptation to pressure overload.
(From Ross J Jr: Afterload mismatch in aortic and mitral valve disease: Implications for surgical therapy. J Am Coll Cardiol 5:811, 1985.)
On the supply side, the greater LVEDP of the poorly compliant ventricle inevitably narrows the diastolic coronary perfusion pressure (CPP) gradient. With severe outflow obstruction, decreases in SV and resultant systemic hypotension may critically compromise coronary perfusion. A vicious cycle may develop because ischemia-induced abnormalities of diastolic relaxation can aggravate the compliance problem and further narrow the CPP gradient.107 This sets the stage for ischemic contractile dysfunction, additional decreases in SV, and worsening hypotension.
Cardiac hypertrophy also is associated with structural abnormalities of the coronary circulation (Figure 19-17).108,109 Animal models have documented that epicardial coronary vessels do not enlarge proportionately when the LV is subjected to a chronic pressure load.110,111 LVH accompanying chronic hypertension is associated with an increased wall lumen ratio of the coronary arterioles, a change that limits vessel dilation and augments active constriction.112,113 Animal models of pressure-induced myocardial hypertrophy also have shown a decrease in the capillary density of about 20% to 30%.108,110,112–116 There is considerable evidence that in LVH caused by pressure overload, a reduction in the coronary vascular reserve may underlie episodes of myocardial ischemia117,118 (see Chapter 6).
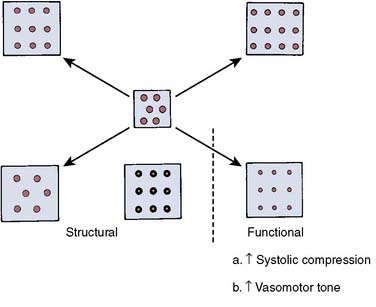
Figure 19-17 Possible coronary circulatory changes accompanying ventricular hypertrophy.
(From Marcus ML: Effects of cardiac hypertrophy on the coronary circulation. In Marcus ML [ed]: The coronary circulation in health and disease. New York: McGraw-Hill, 1983.)
Echocardiographic and hemodynamic assessments of the myocardial oxygen supply and demand ratio are not significantly different in the presence or absence of angina in patients with AS.119 However, considerable data indicate that dynamic factors relative to oxygen supply may be crucial to the pathogenesis of angina in these patients.120 Several clinical studies have documented a decrease in coronary vascular reserve in adult and pediatric patients with significant LVH or right ventricular hypertrophy (RVH).121–123 It is further postulated that repeated episodes of subendocardial ischemia may contribute to the development of subendocardial fibrosis, a component of ischemic contractile dysfunction.108
It is possible that myocardial ischemia underlies the impaired ventricular relaxation that has been documented in patients with myocardial hypertrophy because of a variety of causes.91,93,94 Prolongation of ventricular relaxation and resultant diastolic dysfunction (i.e., poor compliance) are probably universal features of myocardial ischemia in a variety of clinical settings.124–128 Experimental data suggest that ischemia-induced impairment of calcium sequestration by the sarcoplasmic reticulum may underlie the increased diastolic chamber stiffness.129 Prevention of ischemia-induced myoplasmic calcium overload may be the mechanism by which the calcium channel blockers are able to improve diastolic dysfunction in patients with CAD.130 These drugs also ameliorate ventricular relaxation and diastolic filling in patients with HCM, although the mechanism of action is controversial.91,131–133 The absence of diastolic filling abnormalities in patients with physiologic, as opposed to pathologic, hypertrophy also may reflect the relative likelihood of ischemia in the two conditions.134–136
In summary, the pathophysiologic response to chronic pressure overload is a complex one characterized by unique interaction among the divergent effects of hypertrophy on systolic and diastolic function. Mural thickening enhances systolic performance, maximizing the mechanical work performed while minimizing its metabolic cost. The price of this systolic efficiency is relatively inadequate growth of the coronary microcirculation, which contributes to relaxation abnormalities, diastolic dysfunction, and the potential for superimposition of ischemia-induced abnormalities of systolic dysfunction (Table 19-1).137 The potentially deleterious effects of LVH cannot be overemphasized. Even in the absence of AS, in which LVH can be viewed as a compensatory and potentially beneficial pathophysiologic response, LVH has been found to have an independent adverse effect on survival.138,139
Beneficial Aspects | Detrimental Aspects |
---|---|
Increases ventricular work | Decreases ventricular diastolic distensibility |
Normalizes wall stress | Impairs ventricular relaxation |
Normalizes systolic shortening | Impairs coronary vasodilator reserve, leading to subendocardial ischemia |
From Lorell BH, Grossman W: Cardiac hypertrophy: The consequences for diastole. J Am Coll Cardiol 9:1189, 1987.

Difficulty of Low-Gradient, Low-Output Aortic Stenosis
A subset of patients with severe AS, left ventricular dysfunction, and low transvalvular gradient suffers a high operative mortality rate and poor prognosis.140 It is difficult to assess accurately the AVA in this low-flow, low-gradient AS because the calculated valve area is proportional to forward SV and because the Gorlin constant varies in low-flow states. Some patients with low-flow, low-gradient AS have a decreased AVA as a result of inadequate forward SV rather than anatomic stenosis. Surgical therapy is unlikely to benefit these patients because the underlying pathology is a weakly contractile myocardium. However, patients with severe anatomic AS may benefit from valve replacement despite the increased operative risk associated with the low-flow, low-gradient hemodynamic state.140 Guidelines from the ACC and AHA call for a dobutamine echocardiography evaluation to distinguish patients with fixed anatomic AS from those with flow-dependent AS with left ventricular dysfunction.141 Low-flow, low-gradient AS is defined as a mean gradient of less than 30 mm Hg and a calculated AVA less than 1.0 cm2.
Dobutamine echocardiography revealed three basic response patterns—fixed AS, relative AS, and absence of contractile reserve—in an initial study by deFilippi et al.142 In a series of 45 patients with low-flow, low-gradient AS, the 30-day operative mortality rate was 8% for patients with contractile reserve and 50% for those without contractile reserve.143 Dobutamine challenges during cardiac catheterization provided unique insights into low-flow, low-gradient AS, and the details are summarized in Figure 19-18.144 In Figure 19-18A, the transvalvular gradient and cardiac output increased, and the valve area did not change. Patients represented in Figure 19-18B increased their CO with little or no change in gradient, and the calculated valve area increased slightly. This group still benefited from surgery. The third group of patients had no contractile reserve because CO did not increase with dobutamine, and the transvalvular gradient decreased (see Figure 19-18C). Dobutamine infusion in the cardiac catheterization laboratory appears to be helpful in identifying patients with low-flow, low-gradient AS who have a truly fixed anatomic stenosis that may benefit from valve replacement. The findings of these studies also emphasize that contractile reserve is an important prognostic indicator in these patients and dobutamine challenge may help select patients for valve replacement. It appears that patients with contractile reserve and fixed AS have a relatively good prognosis with valve replacement. Left ventricular contractile reserve appears to be a critical variable for prognosis. Recent studies have focused on AS with low transvalvular gradients and normal ventricular EF. The pathophysiology of the low transvalvular gradient has been explained by decreased EDV caused by excessive ventricular hypertrophy accompanied by increased SVR. In a recent series of patients with severe AS (AVA < 0.8 cm2) with ventricular dysfunction (EF < 35%) and/or a low transvalvular gradient (< 30 mm Hg), mortality predictors were advanced age, low EF, renal insufficiency, and lack of AR. Regardless of ventricular function, patients who underwent AVR had a significantly better survival.36
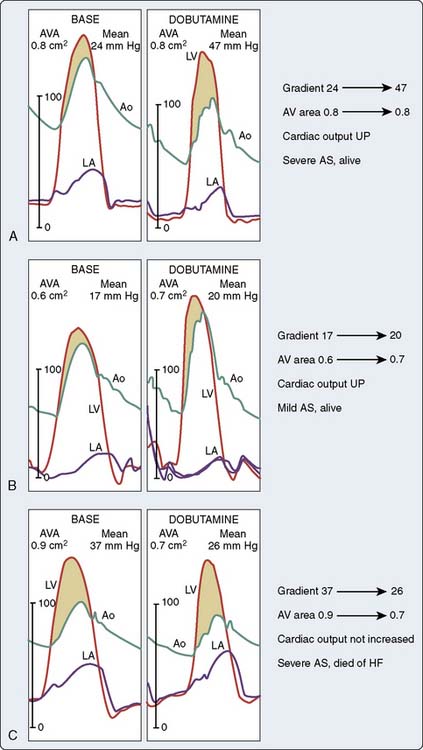
Figure 19-18 Hemodynamic tracings from three patients representing three different responses to dobutamine.
(Adapted from Nishimura RA, Grantham JA, Connolly HM, et al: Low-output, low-gradient aortic stenosis in patients with depressed left ventricular systolic function: The clinical utility of the dobutamine challenge in the catheterization laboratory. Circulation 106:809, 2002.)

Developments in the Hemodynamic Management of Critical Aortic Stenosis Patients
The use of vasodilators is traditionally contraindicated in patients with severe AS because cardiac output is relatively fixed across a narrowed orifice. Vasodilation reduces SVR without any possibility for a compensatory increase in CO and severe hypotension would typically result. This traditional paradigm recently was re-examined in patients with fixed, severe AS (area < 1.0 cm2) and left ventricular dysfunction (EF < 0.35).145