Chapter 18 Vaccination
• Vaccination applies immunological principles to human health. Adaptive immunity and the ability of lymphocytes to develop memory for a pathogen’s antigens underlie vaccination. Active immunization is known as vaccination.
• A wide range of antigen preparations are in use as vaccines, from whole organisms to simple peptides and polysaccharides. Living and non-living vaccines have important differences, living vaccines being generally more effective.
• Adjuvants enhance antibody production, and are usually required with non-living vaccines. They concentrate antigen at appropriate sites or induce cytokines.
• Most vaccines are still given by injection, but other routes are being investigated.
• Vaccine efficacy needs to be reviewed from time-to-time.
• Vaccine safety is an overriding consideration. When immunization frequencies fall, the population as a whole is not protected. Fears over the safety of the MMR vaccine resulted in measles epidemics and increases in incidence of rubella.
• Vaccines in general use have variable success rates. Some vaccines are reserved for special groups only and vaccines for parasites and some other infections are only experimental.
• Passive immunization can be life-saving. The direct administration of antibodies still has a role to play in certain circumstances, for example when tetanus toxin is already in the circulation.
• Non-specific immunotherapy can boost immune activity. Non-specific immunization, for example by cytokines, may be of use in selected conditions.
• Immunization against a variety of non-infectious conditions is being investigated.
• Recombinant DNA technology will be the basis for the next generation of vaccines. Most future vaccines will be recombinant subunit vaccines incorporated into viral or bacterial vectors. This should provide enhanced efficacy and safety.
Vaccination
Vaccines apply immunological principles to human health
• priming of specific lymphocytes to expand the pool of memory cells;
• use of harmless forms of immunogen – attenuated organisms, subcellular fragments, toxoids or vectors;
• use of adjuvants to enhance immune responses; and
• production of safe, affordable vaccines to promote herd immunity.
Vaccines can protect populations as well as individuals
• if the organism is highly infectious so that one individual can rapidly infect several non-immune individuals, as is the case for measles, a high proportion of the population must be immune to maintain herd immunity;
• if the infection is less readily transmitted, immunity in a lower proportion of the population may be sufficient to prevent disease transmission.
Antigen preparations used in vaccines
A wide variety of preparations are used as vaccines (Fig. 18.1). In general, the more antigens of the microbe retained in the vaccine, the better, and living organisms tend to be more effective than killed organisms. Exceptions to this rule are:
• diseases where a toxin is responsible for the pathology – in this case the vaccine can be based on the toxin alone;
• a vaccine in which microbial antigens are inserted into vector and expressed in a host cell.
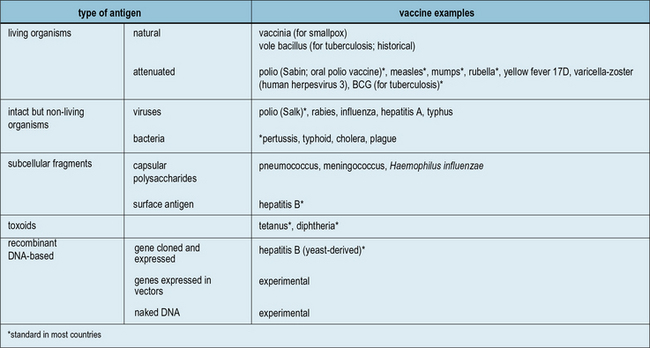
Fig. 18.1 The main antigenic preparations
A wide range of antigenic preparations are used as vaccines.
Live vaccines can be natural or attenuated organisms
Natural live vaccines have rarely been used
Apart from vaccinia, no other completely natural organism has ever come into standard use. However:
The new rotavirus vaccines should prevent many infants from dying in developing countries
Greater knowledge of the viral molecular biology and techniques manipulating the virus in vitro, have now led to the development of two new and highly effective vaccines against rotavirus infection. These vaccines are marketed under the names Rotarix™ and RotaTeq™. The former is a live attenuated virus produced by repeated passage in animal cell lines in the laboratory (see below). The latter is a complex of 5 different hybrid viruses providing immunity to the 5 most prevalent viral strains. These viruses are based on the bovine rotavirus which is naturally attenuated in human hosts. Into this backbone have been incorporated the human rotavirus viral capsid proteins VP4 or VP7, which are known to be the targets of natural immunity in human rotavirus infection (Fig. 18.w1).
Attenuated live vaccines have been highly successful
The real successes were with viruses, starting with the 17D strain of yellow fever virus obtained by passage in mice and chicken embryos (1937), and followed by a roughly similar approach with polio, measles, mumps, and rubella (Fig. 18.2)
Just how successful the vaccines for polio, measles, mumps, and rubella are is shown by the decline in these four diseases between 1950 and 1980 (Fig. 18.3).
Attenuated microorganisms are less able to cause disease in their natural host
Killed vaccines are intact but non-living organisms
Killed vaccines are the successors of Pasteur’s killed vaccines mentioned above:
• some are very effective (rabies and the Salk polio vaccine);
• some are moderately effective (typhoid, cholera, and influenza);
• some are of debatable value (plague and typhus); and
• some are controversial on the grounds of toxicity (pertussis).
Figure 18.4 lists the main killed vaccines in use today. These are gradually being replaced by attenuated or subunit vaccines. However, in the case of polio, some countries are reverting to the use of killed vaccine which is safer than the attenuated vaccine, even though it is less effective. This choice only becomes relevant when the risk of contracting the disease is low in comparison with the risk of developing adverse reactions to the vaccine.
Inactivated toxins and toxoids are the most successful bacterial vaccines
The most successful of all bacterial vaccines – tetanus and diphtheria – are based on inactivated exotoxins (Fig. 18.5), and in principle the same approach can be used for several other infections. An inactive, mutant form of diphtheria toxin (CRM197) has been used as the basis for a number of newly generated conjugate vaccines (see below).
Subunit vaccines and carriers
Aside from the toxin-based vaccines, which are subunits of their respective microorganisms, a number of other vaccines are in use which employ antigens either purified from microorganisms or produced by recombinant DNA technology (Fig. 18.6). For example, a recombinant Hepatitis B surface antigen synthesized in baker’s yeast, has been in use since 1986.
Conjugate vaccines are effective at inducing antibodies to carbohydrate antigens
Starting with Haemophilus influenzae (Hib) in the early 1990s, conjugate vaccines for Neisseria meningitis strains A, C, Y and W-135 are also now in widespread usage. In the UK up until 1992 when the vaccine was introduced, Hib was the major cause of infantile meningitis leading to many hundreds of cases per year. The introduction of the vaccine led to a very rapid decline making Hib meningitis now a very rare occurrence (Fig. 18.7).
Adjuvants enhance antibody production
With modern understanding of the processes leading to lymphocyte triggering and the development of memory, it is hoped that better adjuvants can be developed. Considerable efforts have been made to produce better adjuvants, particularly for T cell-mediated responses. Figure 18.8 gives a list of these, most of which are still highly experimental or too toxic for use in humans. One recent exception however, is monophosphoryl lipid A (MPL). This compound is derived from chemical degradation of lipopolysaccharide (LPS), the major component of the cell wall of gram negative bacteria. MPL retains potent adjuvant activity but, unlike LPS itself, has low toxicity. It is combined with alum in an adjuvant product AS04, which is incorporated in the new HPV vaccine, Cervarix™. In another adjuvant formulation (AS02) MPL is combined with a saponin (QS21) in an oil-water emulsion. AS02 has proven to be of major importance in boosting the immunogenicity of the candidate malaria vaccine RS,S.
Adjuvants concentrate antigen at appropriate sites or induce cytokines
It appears that the effect of adjuvants is due mainly to two activities:
• the concentration of antigen in a site where lymphocytes are exposed to it (the ‘depot’ effect); and
• the induction of cytokines that regulate lymphocyte function.
Particulate antigens such as virus-like particles (polymers of viral capsid proteins containing no viral DNA or RNA) are highly immunogenic and have the useful property that they may also induce cross-priming (i.e. enter the MHC class 1 processing pathway though not synthesized within the APC, see Chapter 8).
Q. Many bacterial carbohydrates and glycolipids are good adjuvants, even though they are not good immunogens. Why should this be so?
A. The discovery of Toll-like receptors (TLRs, see Fig. 6.20) and other pattern recognition receptors, such as lectin-like receptors for carbohydrates (see Fig. 7.10), has provided an explanation for the long-known efficacy of many bacterial products as adjuvants. It is clear that they act mainly by binding to PRRs and stimulating the formation of appropriate cytokines by APCs.
Ligation of different PRRs may bias the response toward TH1 or TH2 cytokine production.
Vaccine administration
Most vaccines are delivered by injection
The main difficulty with cutaneous delivery is penetrating below the outer, cornified layer of the skin. Techniques to improve this such as the uses of microneedle arrays (Fig. 18.9) are under development and may one day allow vaccination using skin patches, similar to those used currently for delivering (small molecule) drugs, such as contraceptives.
Mucosal immunization is a logical alternative approach
• if the bacteria are non-pathogenic they may not immunize;
• if the bacteria are too pathogenic they may cause unpleasant symptoms.
Vaccine efficacy and safety
An effective vaccine must induce the right sort of immunity:
• antibody for toxins and extracellular organisms such as Streptococcus pneumoniae;
• cell-mediated immunity for intracellular organisms such as the tubercle bacillus.
• be stable on storage – this is particularly important for living vaccines, which normally require to be kept cold (i.e. a complete ‘cold chain’ from manufacturer to clinic, which is not always easy to maintain);
• have sufficient immunogenicity – with non-living vaccines it is often necessary to boost their immunogenicity with an adjuvant.
Live vaccines are generally more effective than killed vaccines.
Induction of appropriate immunity depends on the properties of the antigen
Living vaccines have the great advantage of providing an increasing antigenic challenge that lasts days or weeks, and inducing it in the right site – which in practice is most important where mucosal immunity is concerned (Fig. 18.10).
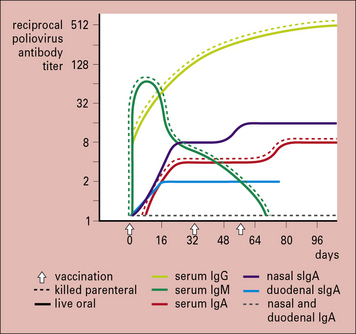
Fig. 18.10 Antibody responses to live and killed polio vaccine
The antibody response to orally administered live attenuated polio vaccine (solid lines) and intramuscularly administered killed polio vaccine (broken lines). The live vaccine induces the production of secretory IgA (slgA) in addition to serum antibodies, whereas the killed vaccine induces no nasal or duodenal sIgA. As slgA is the immunoglobulin of the mucosa-associated lymphoid tissue (MALT) system (see Chapter 2), the live vaccine confers protection at the portal of entry of the virus, the gastrointestinal mucosa.
(Courtesy of Professor JR Pattison, Ch. 26 in Brostoff J, et al., eds. Clinical immunology. London: Mosby; 1991.)
• purified subunits may be relatively poorly immunogenic and require adjuvants;
• the smaller the antigen, the more histocompatibility complex (MHC) restriction may be a problem (see Chapters 5 and 8); and
• purified polysaccharides are typically thymus independent – they do not bind to MHC and therefore do not immunize T cells.
Vaccine safety is an overriding consideration
Vaccine safety is of course a relative term, with minor local pain or swelling at the injection site, and even mild fever, being generally acceptable. More serious complications may stem from the vaccine or from the patient (Fig. 18.11):
• vaccines may be contaminated with unwanted proteins or toxins, or even live viruses;
• supposedly killed vaccines may not have been properly killed;
• attenuated vaccines may revert to the wild type; and
• the patient may be hypersensitive to minute amounts of contaminating protein, or immunocompromised, in which case any living vaccine is usually contraindicated.
• because many childhood infectious diseases have become uncommon in developed countries, the populations of these countries are no longer aware of the potentially devastating effects of infectious diseases;
• unlike most drugs, vaccinations are given to people who have previously been perfectly well;
• the public is becoming increasingly aware of the possibilities of profitable litigation and companies correspondingly more defensive.
MMR controversy resulted in measles epidemics
In 1998 a paper was published that received wide publicity in the UK media, purporting to support an association between MMR vaccination and the development of autism and chronic bowel disease. Although a large amount of subsequent work failed to substantiate these findings and the original paper has now been retracted, take-up of MMR in the UK and Ireland declined over several years and epidemics of measles occurred because of declining herd immunity (Fig. 18.12).
Vaccines in general use have variable success rates
The vaccines in standard use worldwide are listed in Figure 18.13. Four of them – polio, measles, mumps, and rubella – are so successful that these diseases are earmarked for eradication early in the 21st century. If this happens, it will be an extraordinary achievement, because mathematical modeling suggests that they are all more ‘difficult’ targets for eradication than smallpox was.
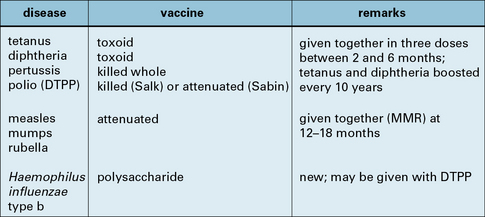
Fig. 18.13 Vaccines in general use
Vaccines that are currently given, as far as is possible, to all individuals.
• the carrier state – eradication of hepatitis B would be a major triumph, but it will require the breaking of the carrier state, especially in the Far East, where mother to child is the normal route of infection;
• suboptimal effectiveness – effectiveness of BCG varies markedly between countries, possibly due to variation in environmental mycobacterial species (tuberculosis is increasing, especially in patients with immune deficiency or AIDS), and the pertussis vaccine is only about 70–80% effective;
• safety fears – especially when the risk of infection is low, often lead to reduced levels of uptake. In the UK the spurious association of the MMR vaccine with autism has continued to affect the public’s willingness to be vaccinated;
• free-living forms and animal hosts – the free-living form of tetanus will presumably survive indefinitely and it will not be possible to eradicate diseases that also have an animal host, such as yellow fever.
Some vaccines are reserved for special groups only
In the developed world BCG and hepatitis B fall into this category, but some vaccines will probably always be confined to selected populations – travelers, nurses, the elderly, etc. (Fig. 18.14). In some cases this is because of:
• geographical restrictions (e.g. yellow fever);
• the rarity of exposure (e.g. rabies);
• problems in producing sufficient vaccine in time to meet the demand (e.g. each influenza epidemic is caused by a different strain, requiring a new vaccine).
Vaccines for parasitic and some other infections are only experimental
Some of the most intensively researched vaccines are those for the major tropical protozoal and worm infections (see Chapter 15). However, none has come into standard use, and some have argued that none will because none of these diseases induces effective immunity and ‘you cannot improve on nature’.
• none of the small animal models of malaria shows such extensive antigenic variation as Plasmodium falciparum, the protozoon causing malignant tertian malaria in humans;
• similarly, rats appear to be much easier to immunize against schistosomiasis than other animals, including possibly humans.
A vaccine against Plasmodium falciparum, has been one of the most sought after for over a generation, as the burden of disease and death in endemic malarial regions in Africa is huge. Malaria is unusual in that its life cycle offers a variety of possible targets for vaccination (Fig. 18.15). Over the years several trials of clinical malaria vaccine have been published, using antigens derived from either the liver or the blood stage, with only very moderate success.
During the last five years or so, however, a realistic candidate vaccine has emerged from a partnership begun in the early 1980s, between the pharmaceutical company GlaxoSmithKline (GSK) and the US Walter Reed Army Institute of Research (WRAIS). The vaccine known as RS,S is based on a genetically engineered version of the circumsporozoite (CS) protein that is expressed on sporozoites and liver stage schizonts (see Fig. 18.15). Recombinant CS protein is expressed in yeast cells as a fusion protein with the Hepatitis B surface antigen (HBsAg), the basis of the successful recombinant HepB vaccine. Co-expression of the fusion protein along with unmodified HBsAg, allows the formation of VLP-type aggregates of the antigens. To make this preparation sufficiently immunogenic has required combination with the powerful new adjuvant AS02 (see above).
Other viral and bacterial vaccines that are also experimental are:
For many diseases there is no vaccine available
No vaccine is currently available for many serious infectious diseases, including staphylococci and streptococci, syphilis, chlamydia, leprosy, and fungal infections. The predominant problem is often the lack of understanding of how to induce effective immunity. HIV infection heads this list of diseases (see Chapter 17), which represent the major challenge for research and development in the coming decade (Fig. 18.w2)
Passive immunization can be life-saving
Driven from use by the advent of antibiotics, the idea of injecting preformed antibody to treat infection is still valid for certain situations (Fig. 18.16). It can be life-saving when:
• toxins are already circulating (e.g. in tetanus, diphtheria, and snake-bite);
• high-titer specific antibody is required, generally made in horses, but occasionally obtained from recovered patients.
Antibody genes can now be engineered to form Fab, single chain Fv, or VH fragments (see Chapter 3). Libraries of these can be expressed in recombinant phages and screened against antigens of interest. Selected antibody fragments can be produced in bulk in bacteria, yeasts, or mammalian cells, for use in vitro or in vivo. This technology has helped in the production of human and humanized mouse monoclonal antibodies for therapeutic application.
Non-specific immunotherapy can boost immune activity
Many of the same compounds that act as adjuvants for vaccines have also been used on their own in an attempt to boost the general level of immune activity (Fig. 18.w3). The best results have been obtained with cytokines, and among these IFNα is the most widely used, mainly for its antiviral properties (but also for certain tumors – see below).
Immunization against a variety of non-infectious conditions is being investigated
The most obvious candidate would be cancers, which are known to sometimes be spontaneously rejected as if they were foreign grafts (see Chapter 22). A large quantity of research is now directed at trying to induce autoimmune rejection of tumors, mainly utilizing genetic modification approaches to increase immunogenicity for the host.
Other uses of immunization that are being explored include:
• treatment of autoimmune disease;
• the treatment of drug dependency, because it is possible to neutralize the effect of a drug by pre-immunization with the drug coupled to a suitable carrier (the ‘hapten-carrier’ effect);
• reducing blood pressure to treat essential hypertension;
• lowering cholesterol by ‘neutralizing’ lipid-binding proteins; and
• preventing Alzheimer’s disease by immunizing against components of amyloid plaque.
Future vaccines
‘Naked’ DNA can be transfected into host cells
Critical thinking: Vaccination (see p. 439 for explanations)
1. Why have attenuated vaccines not been developed for all viruses and bacteria?
2. ‘A vaccine cannot improve on nature’. Is this unduly pessimistic?
3. ‘The smallpox success story is unlikely to be repeated.’ Is this true?
4. Will vaccines eventually replace antibiotics?
5. BCG: vaccine, adjuvant, or non-specific stimulant?
6. Why could an anti-worm vaccine do more harm than good?
7. By what means, other than their reaction with antibodies, might you identify antigens that could be used as vaccines?
Almond J.W. Vaccine Renaissance. Nat Rev Microbiol. 2007;5:478–481.
Kaufmann S.H.E. The contribution of immunology to the rational design of novel antibacterial vaccines. Nat Rev Microbiol. 2007;5:491–504.
Kusters I., Almond J.W. Vaccine Strategies. In: Mahy D.W.J., van Regenmortel M.H.V. Desk Encylopedia of General Virology. Oxford UK and San Diego USA: Academic Press; 2010:235–243.
Pollard A.J., Perret K.P., Beverly P.C. Maintaining protection against invasive bacteria with polysaccharide conjugate vaccines. Nat Rev Immunol. 2009;9:213–220.