Chapter 23 Immediate Hypersensitivity (Type I)
• The classification of hypersensitivity reactions is based on the system proposed by Coombs and Gell.
• Historical observations have shaped our understanding of immediate hypersensitivity. The severity of symptoms depends on IgE antibodies, the quantity of allergen, and also a variety of factors that can enhance the response including viral infections and environmental pollutants.
• Most allergens are proteins.
• Production of IgE depends on genotype. In genetically predisposed individuals, IgE production occurs in response to repeated low-dose exposure to inhaled allergens such as dust mite, cat dander, or grass pollen.
• Allergens are the antigens that give rise to immediate hypersensitivity and contribute to asthma rhinitis or food allergy.
• Mast cells and basophils contain histamine. IgE antibodies bind to a specific receptor, FcεRI, on mast cells and basophils. This Fc receptor has a very high affinity, and when bound IgE is cross-linked by specific allergen, mediators including histamine, leukotrienes, and cytokines are released.
• Multiple genes have been associated with asthma in different populations. Multiple genetic loci influence the production of IgE, the inflammatory response to allergen exposure, and the response to treatment. Polymorphisms have been identified in the genes, in promoter regions, and in the receptors for IgE, cytokines, leukotrienes, and the β2-receptors.
• Skin tests are used for diagnosis and as a guide to treatment.
• Several different pathways contribute to the chronic symptoms of allergy.
• Immunotherapy can be used for hayfever and anaphylactic sensitivity.
• New approaches are being investigated for treating allergic disease.
Classification of hypersensitivity reactions
• Immediate (Type I) hypersensitivity responses are characterized by the production of IgE antibodies against foreign proteins that are commonly present in the environment (e.g. pollens, animal danders, or house dust mites) and can be identified by wheal and flare responses to skin tests which develop within 15 minutes.
• Antibody-mediated (Type II) hypersensitivity reactions occur when IgG or IgM antibodies are produced against surface antigens on cells of the body. These antibodies can trigger reactions either by activating complement (e.g. autoimmune hemolytic anemia) or by facilitating the binding of natural killer cells (see Chapter 24);.
• Immune complex diseases (Type III hypersensitivity) involve the formation of immune complexes in the circulation that are not adequately cleared by macrophages or other cells of the reticuloendothelial system. The formation of immune complexes requires significant quantities of antibody and antigen (typically microgram quantities of each). The classical diseases of this group are systemic lupus erythematosus (SLE), chronic glomerulonephritis, and serum sickness (see Chapter 25).
• Cell-mediated reactions (Type IV hypersensitivity) are those in which specific T cells are the primary effector cells (see Chapter 26). Examples of T cells causing unwanted responses are:
The original Coombs and Gell classification is shown in Figure 23.1.
Historical perspective on immediate hypersensitivity
• human anaphylaxis had no familial characteristics (unlike most of the other allergic diseases); and
• natural exposure to inhaled allergens did not cause anaphylaxis or urticaria.
Anaphylaxis may also occur as a result of eating an allergen such as peanut or shellfish, or following the rupture of hydatid cysts with the rapid release of parasite antigens (Fig. 23.2).
Characteristics of type I reactions
Most allergens are proteins
Substances that can give rise to wheal and flare responses in the skin and to the symptoms of allergic disease are derived from many different sources (see http://www.allergen.org/). When purified they are almost all found to be proteins and their sizes range from 10–40 kDa. These proteins are all freely soluble in aqueous solution, but have many different biological functions including digestive enzymes, carrier proteins, calycins, and pollen recognition proteins.
Any allergen can be described or classified by its source, route of exposure, and nature of the specific protein (Fig. 23.3).
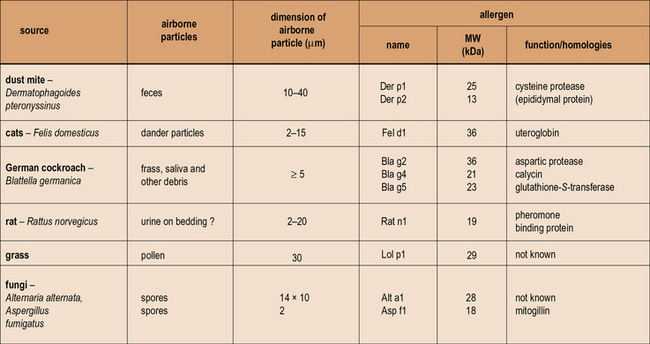
Fig. 23.3 Properties of allergens
Patients who become ‘allergic’ to one of the well-recognized sources of allergens have actually produced an IgE antibody response to one or more of the proteins produced by mites, trees, grass, cats, or fungi. The proteins are predominantly water soluble with a molecular weight (MW) ranging from 10–40 kDa. In many cases the function of the proteins is known, but it is not clear whether function such as enzymic activity alters the ability of these proteins to induce an allergic response. The properties of the particles carrying these allergens are very important because they influence both how much becomes airborne, and also where the allergen is deposited in the respiratory tract. The dimensions of the particles airborne vary from ≤2 μm for Aspergillus or Penicillium spores to ≥20 μm for mite fecal pellets and some pollen grains. (Sizes are given as diameter in μm. For a full list of allergens see http://www.allergen.org/.)
IgE is distinct from the other dimeric immunoglobulins
IgE is distinct from the other dimeric immunoglobulins because it has:
• an extra constant region domain;
• a different structure to the hinge region; and
• binding sites for both high- and low-affinity IgE receptors, FcεRI and FcεRII, respectively (see Fig. 3.19).
The properties of IgE can be separated into three areas:
• the characteristics of the molecule including its half-life and binding to IgE receptors;
• the control of IgE and IgG antibody production by T cells; and
• the consequences of allergen cross-linking IgE on the surface of mast cells or basophils.
The half-life of IgE is short compared with that of other immunoglobulins
• serum IgE has a much shorter half-life than other isotypes (~2 days compared with 21–23 days for IgG);
• IgE is produced in small quantities and is only produced in response to a select group of antigens (allergens and parasites); and
• IgE antibodies are sequestered on the high-affinity receptor on mast cells and basophils.
Q. What fundamental reason explains the low production of IgE?
A. The class switch from IgM to IgE happens infrequently and is controlled by T cells. The position of the IgE constant region gene is towards the distal end of the Ig gene stack (see Fig. 9.17), but the position alone cannot explain the infrequency of the switch to IgE.
Serum is constantly being taken up by endocytosis. Many macromolecules including IgE degrade in the endosome. One major exception is IgG, which is protected by binding to the neonatal Fc gamma receptor, FcγRn (Fig. 23.4).
T cells control the response to inhalant allergens
IgE production is dependent on TH2 cells
T cells that suppress TH2 responses including IgE production:
• act predominantly by producing interferon-γ (IFNγ); and
• are produced when the animal (e.g. mouse, rat, or rabbit) is primed in the presence of Freund’s complete adjuvant.
The main cytokines that are specifically relevant to a TH1 response include:
By contrast, the primary cytokines relevant to a TH2 response are:
Both IgE and IgG4 are dependent on IL-4
The genes for immunoglobulin heavy chains are in sequence on chromosome 14. The gene for ε occurs directly following the gene for γ4. Both of these isotypes are dependent on IL-4 and they may be expressed sequentially (Fig. 23.6).
Recently, it has been shown that children raised in a house with a cat can produce an IgG response, including IgG4 antibody, without becoming allergic. A modified TH2 response (increased IgG4 and decreased IgE) therefore represents an important mechanism of tolerance to allergens (Fig. 23.7). IgG4 antibody responses without IgE antibody are a feature of immunity/tolerance to insect venom, rat urinary allergens, and food antigens as well as cat allergens.
Characteristics of allergens
Allergens have similar physical properties
The primary characterization of allergens relates to their route of exposure. The routes includes:
The inhalant allergens cause hayfever, chronic rhinitis, and asthma
Allergens can only become airborne in sufficient quantity to cause an immune response or symptoms when they are carried on particles. Pollen grains, mite fecal particles, particles of fungal hyphae or spores, and animal skin flakes (or dander) are the best defined forms in which allergens are inhaled (Fig. 23.8).
In each case it is possible to define the approximate particle size and the quantity of protein on the particle as well as the speed with which the proteins in the particle dissolve in aqueous solution (see Fig. 23.3).
Thus, for grass pollen, mite fecal pellets, and cat dander:
• the relevant allergens are present in high concentrations within the particles (up to 10 mg/cm3);