Chapter 25 Tricuspid Atresia
Tricuspid atresia was described in 1817,1 but almost a century elapsed before the great arterial relationships were defined.2 Because of the morphologic heterogeneity of the malformation, “manifold anatomic combinations can result in this haemodynamic arrangement.”3,4 The incidence rate has been estimated at 0.06 per 1000 live births, with a prevalence rate of 1% to 3% of congenital heart disease.5,6 An unguarded tricuspid orifice is different again.7 This chapter is concerned with hearts in situs solitus without ventricular inversion in which a physiologic or anatomic connection does not exist between the morphologic right atrium and the morphologic right ventricle. In 95% of patients, absence of the right atrioventricular connection is the result of fibro-fatty tissue that is interposed between the muscular floor of the right atrium and the parietal wall of the ventricular mass.8 In the remaining 5%, atresia is produced by an imperforate tricuspid valvular membrane.4,8 Systemic venous return cannot directly reach the ventricular portion of the heart but instead crosses the atrial septum from a morphologic right atrium into a morphologic left atrium, where it mixes with pulmonary venous return before traversing a solitary atrioventricular valve into a morphologic left ventricle, which is the only pumping chamber for the pulmonary and systemic circulations (Figure 25-1).3 This arrangement has been referred to as a “functionally” univenricular heart.4 Atresia of the tricuspid valve with Ebstein’s anomaly and atresia of the right atrioventricular valve with single ventricle are dealt with in Chapters 13 and 26.
Tricuspid atresia, as just defined, has certain anatomic features that consistently recur and certain features that are variable.6,9,10 Consistent features include: (1), physiologic and anatomic absence of a connection between the morphologic right atrium and the morphologic right ventricle; (2), hypoplasia of the morphologic right ventricle; (3), an interatrial communication; and (4), a morphologic left ventricle equipped with a morphologic mitral valve. The variable features provide the rationale for a clinical classification based on three gross morphologic features4,6,9–11: (1), transposed or nontransposed great arteries; (2), the presence or absence of pulmonary stenosis (Figures 25-2 and 25-3); and (3), the size of the coexisting ventricular septal defect. An embryologic classification is based on two microscopic features: (1), rudiments of an atretic tricuspid apparatus that create a dimple on the floor of the right atrium that can be localized with transillumination by a light source placed within the hypoplastic right ventricle10,12,13; and (2), a fibrous atrioventricular remnant that forms a microscopic tract from the right atrium to a tiny inlet component of the subjacent right ventricle.14 Failure of expansion of this exceedingly small inlet component during early embryogenesis is believed to be the pathogenetic mechanism responsible for most cases of tricuspid atresia.14 Congenital tricuspid stenosis is a less severe form of the malformation in which a well-formed tricuspid valve joins the small inlet portion of the right ventricle (Figure 25-4).12
In approximately three fourths of cases, an interatrial communication exists in the form of a restrictive patent foramen ovale.15 The valve of the foramen ovale occasionally protrudes aneurysmally as the obstructed right atrium vainly seeks an exit.16,17 The aneurysmal protrusion can obstruct left atrial flow.17 A much less common form of interatrial communication is an atrial septal defect that is almost always ostium secundum.9,15
The great arteries are nontransposed in approximately 90% of cases.11,15 Pulmonary blood flow then depends on the condition of the ventricular septum (see Figure 25-2). The arrangement at birth is usually a restrictive ventricular septal defect (see Figure 25-2B) that constitutes a zone of subpulmonary stenosis, which is physiologically advantageous when blood flow to the lungs is adequate but not excessive. The advantage is lost in about 40% of cases because the defect decreases in size or closes altogether—acquired pulmonary atresia (see Figures 25-2A, and 25-6).15,18–20 The time course of spontaneous closure is similar to that of isolated perimembranous ventricular septal defects (see Chapter 17), with most that are destined to close doing so in the first year of life.15,18–20 Rarely, the ventricular septum is congenitally intact and the pulmonary valve is atretic, an arrangement that completely denies the left ventricle access to the pulmonary circulation (see Figure 25-2A). Also rarely, obstruction is exclusively at valve level because a bicuspid pulmonary valve is stenotic.21 A nonrestrictive ventricular septal defect (Figures 25-2C, and 25-5) permits unobstructed flow from left ventricle to main pulmonary artery. The right ventricle is well-developed, and the pulmonary valve is normally formed. Pulmonary blood flow is regulated by pulmonary vascular resistance.6
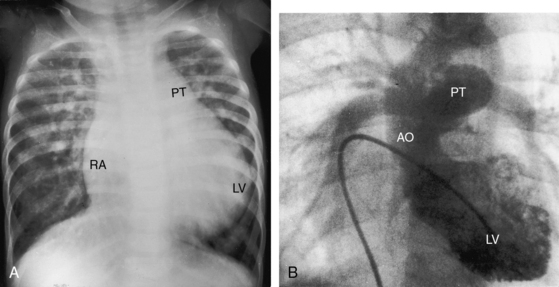
Figure 25-5 A, X-ray from a 6-year-old girl with tricuspid atresia, normally related great arteries, a nonrestrictive ventricular septal defect, low pulmonary vascular resistance, and a large ostium secundum atrial septal defect (see Figure 25-2C). Pulmonary blood flow is markedly increased, the pulmonary trunk (PT) is prominent, the right atrium (RA) is enlarged, and a dilated left ventricle (LV) occupies the apex. B, Left ventriculogram from a 4-year-old girl with tricuspid atresia, normally related great arteries, a nonrestrictive ventricular septal defect, and low pulmonary vascular resistance. The pulmonary trunk (PT) and its branches are dilated, and the left ventricle is enlarged. Compare with Figure 25-2C.
Tricuspid atresia with complete transposition of the great arteries typically occurs with a nonrestrictive ventricular septal defect without pulmonary stenosis (see Figure 25-3).11,15 Left ventricular blood has unobstructed access to the transposed aorta through a well-developed right ventricle. Pulmonary blood flow is regulated by pulmonary vascular resistance because the transposed pulmonary trunk originates from the left ventricle (see Figure 25-3). Pulmonary vascular disease usually develops in the first year of life (Figures 25-7 and 25-8).15 A decrease in size or spontaneous closure of the ventricular septal defect constitutes a zone of subaortic stenosis because the transposed aorta arises from the right ventricle.22 Pulmonary stenosis is infrequent, and pulmonary atresia is rare (see Figure 25-3).23
Distribution of the coronary arteries in tricuspid atresia is analogous to, if not identical with, that of univentricular hearts with a single morphologic left ventricle and an outlet chamber (see Chapters 26 and 32).24 The rudimentary right ventricle of tricuspid atresia and the right ventricular remnant of single ventricle are both delimited by coronary arteries.24
Additional anatomic variables associated with tricuspid atresia involve the mitral valve,25 the ductus arteriosus, the ascending aorta, the aortic isthmus, the atrial appendages, and the pulmonary valve.26 Abnormalities of the mitral valve are represented by myxomatous, redundant, or prolapsing leaflets; a cleft anterior leaflet; and direct attachment of leaflets to papillary muscles.25 When the great arteries are nontransposed and the ventricular septum is congenitally intact, which is physiologic pulmonary atresia, the fetal ductus arteriosus functions as a small malformed aortic tributary.27 The ascending aorta and isthmus are large because the aorta receives the entire cardiac output. When the great arteries are transposed and the ventricular septal defect is restrictive, left ventricular blood is diverted into the pulmonary trunk, so the ductus arteriosus enlarges while the ascending aorta and isthmus are underfilled and hypoplastic.15,28 Very rarely, the pulmonary valve is absent.26 Juxtaposition of the atrial appendages, a condition in which both appendages lie on one side of the great arteries,15,29–31 almost always means that the great arteries are transposed (see Chapter 27). Juxtaposition is present in about 50% of patients with tricuspid atresia and complete transposition.
The physiologic consequences of tricuspid atresia begin with the obligatory right-to-left shunt at the atrial level. The left atrium receives the normal pulmonary venous return together with the systemic venous return across the interatrial communication.32 The left atrial mixture flows across a morphologic mitral valve into a morphologic left ventricle, which is the sole pumping chamber for the systemic and pulmonary circulations. When the great arteries are not transposed, pulmonary blood flow is reduced because a restrictive ventricular septal defect constitutes a zone of subpulmonary stenosis (see Figures 25-2 and 25-6). This arrangement accounts for about 90% of cases. Left ventricular volume overload is curtailed at the price of increased cyanosis. When the ventricular septal defect is nonrestrictive and pulmonary vascular resistance is low, pulmonary blood flow and left ventricular volume overload are excessive, and cyanosis is mild (see Figure 25-5). When the great arteries are transposed, the ventricular septal defect is usually nonrestrictive and pulmonary stenosis is usually absent (see Figure 25-3). Low pulmonary vascular resistance results in increased pulmonary blood flow, mild cyanosis, and left ventricular volume overload.33,34 The degree of pulmonary vascular resistance that achieves adequate but not excessive pulmonary blood flow is a delicate balance that is seldom realized (see Figures 25-7 and 25-8).15
History
When tricuspid atresia occurs with normally related great arteries, males and females are equally represented,15 but when the great arteries are transposed, males predominate15 unless there is juxtaposition of the atrial appendages.31 Tricuspid atresia has been reported in siblings,35–37 in families,38 and in experimental animals.39
In about 6% of infants with tricuspid atresia, birth is premature.5 Survival depends on an adequate interatrial communication and adequate regulation of pulmonary blood flow.15,23 Increased longevity incurs the risk of infective endocarditis, paradoxical emboli, and brain abcess.15,22,40 Life span is less than 6 months when tricuspid atresia occurs with normally related great arteries and pulmonary atresia (see Figure 25-2A), but exceptional survivals have been recorded at age 21 years41 and 22 years.42 Acquired pulmonary atresia takes the form of spontaneous closure of the ventricular septal defect (see previous), an eventuality that usually occurs in the first year of life.15,19 Survival then depends on patency of the ductus arteriosus, an advantage that is seldom realized. Exceptional survivals have nevertheless been reported at age 8 years (see Figure 25-1), 18 years,15 and 27 years,20 in addition to a 21-year-old woman who survived because adequate pulmonary blood flow was achieved by an anomalous artery connecting the ascending aorta to the pulmonary trunk.3 The most exceptional survival was a 65-year-old man with tricuspid atresia, pulmonary atresia, an ostium secundum atrial septal defect, and large aortic-to-pulmonary arterial collaterals.43
Most patients with tricuspid atresia and normally related great arteries die in the first year because an already restrictive ventricular septal defect decreases in size or closes altogether.15 When the ventricular septal defect adequately regulates pulmonary blood flow, survivals have been realized from the second into the fifth decade.44,45 Two patients lived to 57 years of age,23 and a 30-year-old woman had a relatively uneventful pregnancy and a dysmature but otherwise healthy offspring.46 If the ventricular septal defect is nonrestrictive (see Figure 25-2C), increased pulmonary blood flow results in excessive volume overload of the left ventricle and congestive heart failure. However, one such patient was alive at age 6 years (see Figure 25-5), and survivals have been reported to age 32 years and 45 years,40,47 with an exceptional survival to age 57 years.48
The same longevity patterns occur with tricuspid atresia, complete transposition of the great arteries, and a nonrestrictive ventricular septal defect (see Figure 25-3C) in which regulation of pulmonary blood flow depends on pulmonary vascular resistance (see Figure 25-8). Exceptional survivals have been reported to the mid and late teens.15,49 Satisfactory regulation of pulmonary blood flow is more likely to be achieved by pulmonary stenosis (see Figure 25-3B)15 with which isolated patients have lived into the second, third, and fourth decades,21,23,40 with one patient dying at age 56 years.50
Survival in congenital tricuspid stenosis depends on the degree of obstruction and on an adequate interatrial communication.51,52 A 20-year-old woman was acyanotic (see Figure 25-11),52 a cyanotic patient underwent surgical repair at age 50 years (see Figure 25-4), and a cyanotic man survived to age 57 years.51
Hypoxic spells precipitated by a reduction in size or spontaneous closure of a restrictive ventricular septal defect are characterized by sudden deepening of cyanosis followed by paroxysmal dyspnea, lethargy, and syncope.6,15,52 Older children squat for relief of breathlessness.23,44 Syncope has been attributed to intermittent occlusion of the interatrial communication by a large spinnaker-like valve of the sinus venosus.53
Physical appearance
Physical appearance in tricuspid atresia reflects the cyanosis of venoarterial mixing and decreased pulmonary blood flow and the catabolic effects of congestive heart failure and excessive pulmonary blood flow. A left precordial bulge does not occur because the right ventricle is underdeveloped. The cat-eye syndrome is a distinctive although uncommon appearance characterized by fissures of the iris (congenital coloboma) and an oblong pupillary shape.54
Arterial pulse
The arterial pulse is normal (Figure 25-9B) unless pulmonary blood flow is excessive and the left ventricle has failed. The pulses are feeble if not undetectable when tricuspid atresia occurs with transposition of the great arteries, a restrictive ventricular septal defect, and systemic hypoperfusion.55
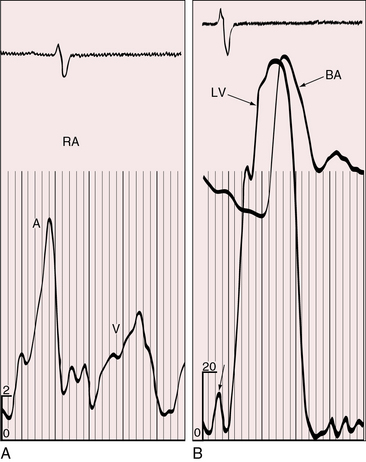
Figure 25-9 Tracings from an 18-year-old man with tricuspid atresia, complete transposition of the great arteries, and pulmonary vascular disease. X-rays are shown in Figure 25-8. A, Right atrial (RA) pressure pulse showing a prominent A wave. B, Presystolic distention of the left ventricle (unmarked lower left arrow) reflected the prominent right atrial A wave that was transmitted into the left ventricle (LV) through a nonrestrictive atrial septal defect. (BA = brachial artery pulse.)
Jugular venous pulse
The A wave is increased when a restrictive interatrial communication obstructs egress from the right atrium (Figure 25-10). Large A waves are also features of congenital tricuspid stenosis (Figure 25-11). The Y descent is slow when a restrictive interatrial communication impedes right atrial flow during the passive filling phase. The A wave increases when a thick-walled left ventricle resists right atrial contraction (see Figure 25-9A). A and V waves are both increased when left ventricular failure provokes a rise in mean pressure in a left atrium that is in continuity with the right atrium. Large V waves of mitral regurgitation are transmitted into the right atrium when the interatrial communication is nonrestrictive.44
Precordial movement and palpation
A key to the clinical recognition of tricuspid atresia is the combination of a left ventricular impulse in a cyanotic patient in whom a right ventricular impulse is conspicuously absent. The left ventricle remains palpable even when pulmonary blood flow is reduced because the chamber handles the entire output of the systemic and pulmonary circulations. When pulmonary blood flow is increased, the impulse of the volume overload left ventricle is also increased.33 A gentle right ventricular impulse is reserved for the occasional patient with a nonrestrictive ventricular septal defect and a relatively well-developed right ventricle.
Auscultation
Auscultatory signs are determined by the anatomic variations beyond the mitral valve. The single first heart sound is necessarily the mitral component because the tricuspid valve is atretic.56 When the great arteries are not transposed, a holosystolic systolic murmur is generated across the restrictive ventricular septal defect (Figures 25-12, 25-13, and 25-14). The murmur is maximal at the mid to lower left sternal edge but radiates to the second left intercostal space when the left ventricle ejects through the ventricular septal defect into the pulmonary trunk (see Figure 25-13). When the ventricular septal defect decreases in size or closes spontaneously, the holosystolic murmur becomes early systolic before vanishing altogether.18 The second heart sound is represented by the aortic component, although a soft delayed pulmonary component is occasionally detected because the restrictive ventricular septal defect is functionally subpulmonary stenosis (see Figure 25-13). When the ventricular septum is intact from birth (functional congenital pulmonary atresia; see Figure 25-2A), there is either no murmur at all or a short midsystolic murmur into a dilated aortic root. Continuous murmurs through aortopulmonary collaterals are exceptional (Figure 25-15).20
Tricuspid atresia with transposition of the great arteries (see Figure 25-3C) is accompanied by a holosystolic murmur that is generated across the ventricular septal defect, which is usually nonrestrictive (Figure 25-16). A single loud second heart sound is aortic because the transposed aorta is anterior (see Figure 25-16). A third heart sound introduces a mid-diastolic murmur generated by increased flow across the mitral valve (see Figure 25-16). When elevated pulmonary vascular resistance reduces pulmonary blood flow, left ventricular stroke volume falls, the ventricular septal defect murmur decreases or disappears (Figure 25-17), and a soft midsystolic murmur is generated into the anterior aortic root. The second heart sound is audibly split because the hypertensive dilated posterior pulmonary trunk generates a loud pulmonary component (see Figure 25-17). Pulmonary or subpulmonary stenosis generates a midsystolic murmur, the loudness and length of which vary inversely with the degree of obstruction because the more severe the stenosis, the more left ventricular blood is diverted into the aorta via the ventricular septal defect (see Figure 25-3B). Flow across the stenotic sites falls reciprocally, so the pulmonary stenotic murmur softens and shortens (Figure 25-18). The posterior position of the pulmonary trunk decreases the murmur still further.
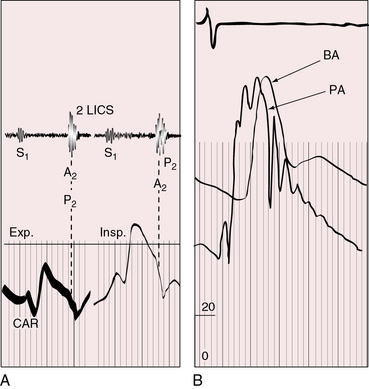
Figure 25-17 Tracings from an 18-year-old man with tricuspid atresia, complete transposition of the great arteries, and pulmonary vascular disease. His x-rays are shown in Figure 25-8. A, Phonocardiogram from the second left intercostal space (2 LICS) recorded a single first heart sound (S1) and no murmur. There was close inspiratory splitting of the second heart sound (A2/P2) because pulmonary vascular resistance was lower than systemic resistance (see diastolic pressures in panel B). The pulmonary component of the second sound was prominent because the posterior pulmonary trunk was dilated and hypertensive. (CAR = carotid.) B, Brachial arterial (BA) and pulmonary arterial (PA) pressures were equal during systole but diverged during diastole.
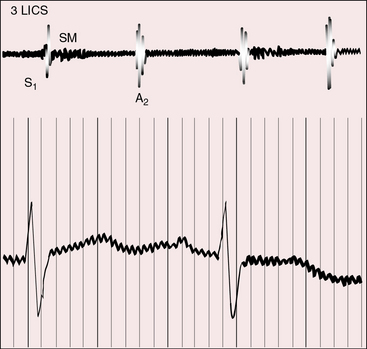
Figure 25-18 Phonocardiogram from the third left intercostal space (3 LICS) of an 18-month-old boy with tricuspid atresia, transposition of the great arteries, and severe pulmonary stenosis. The pulmonary stenotic murmur (SM) was trivial because left ventricular blood was diverted away from the pulmonary trunk across a nonrestrictive ventricular septal defect into the transposed aorta (see Figure 25-3B). The aortic component of the second heart sound was prominent (A2) because the transposed aorta was anterior.
Left ventricular fourth heart sounds occur when the interatrial communication is nonrestrictive and the increased force of right atrial contraction is transmitted into the left ventricle. Presystolic murmurs are expected in congenital tricuspid stenosis (Figure 25-19)52 and are possible in tricuspid atresia when powerful right atrial contraction is exerted against a restrictive interatrial communication.
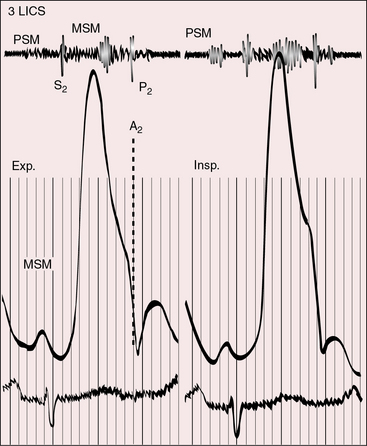
Figure 25-19 Phonocardiogram from the third left intercostal space (3 LICS) of the 20-year-old woman with tricuspid stenosis and pulmonary stenosis. Her right atrial and right ventricular pressure pulses are shown in Figure 25-11. The presystolic murmur of tricuspid stenosis (PSM) increased during inspiration (Insp.). The midsystolic murmur (MSM) was across the stenotic pulmonary valve. (CAR = carotid pulse; Exp. = expiration.)
Electrocardiogram
Tall peaked “Himalayan” right atrial P waves are typical, especially in lead 2 (Figures 25-20 and 25-21).15,57–60 The P terminal force can be dramatically negative in lead V1 (see Figure 25-21). The left atrium is seldom represented, even though it receives the entire return from both the systemic and the pulmonary veins.57,58 There is no necessary relationship between P wave morphology and the size of the interatrial communication.57 Tricuspid atresia with normally related great arteries and a restrictive ventricular septal defect is suspected when left axis deviation and left ventricular hypertrophy occur in a cyanotic patient (see Figures 25-20 and 25-21).15,57–59,61
The left axis deviation of tricuspid atresia was commented on in 1929,62 and its diagnostic value was emphasized by Helen Taussig63 in 1936. The cause has not been firmly established,57,64–66 but left anterior fascicular block is not the mechanism.64,66 The central fibrous body is abnormally formed whether or not the great arteries are transposed.64 A fibrous strand has been traced to the cavity of the rudimentary right ventricle,14 and the atrioventricular node pierces the central fibrous body to form the His bundle.64 The left bundle branches originate close to the atrioventricular (AV) node/His bundle junction and undergo early arborization, whereas the right bundle branch is elongated.58 It is doubtful that early arborization of the left bundle accounts for left axis deviation, which appears to depend largely on the relative masses of the right and left ventricles. Aborization patterns of the bundle branches are the same in all varieties of tricuspid atresia.57,58 Left axis deviation and adult progression of the precordial QRS pattern coexist (see Figures 25-20 and 25-21).58,59 Left ventricular hypertrophy is represented by deep S waves in right precordial leads and tall R waves with repolarization abnormalities in leads aVL and V5-6 (see Figures 25-20 and 25-21).
Tricuspid atresia with complete transposition of the great arteries typically occurs with a nonrestrictive ventricular septal defect and a well-developed right ventricle. Increased pulmonary blood flow adds materially to left atrial volume. Left and right atrial enlargement coexist, so the P waves are broad, bifid, and peaked (Figure 25-22).15,57,58,61 The relatively well-developed right ventricle contributes to the normal QRS axis and probably influences the QRS pattern in the precordial leads.15,58,59
X-ray
The cardiac silhouette can be distinctive in tricuspid atresia with a restrictive ventricular septal defect and normally related great arteries (Figures 25-23 and 25-24).21 The right cardiac contour exhibits a distinctive, prominent superior border caused by enlargement of the right atrium and its appendage and accentuated by a flat receding inferior border that reflects absence of the right ventricle (Figures 25-23B, 25-24, and 25-25B). A convex left ventricle occupies the apex (see Figures 25-23A, and 25-25A). Pulmonary vascularity is reduced and the main pulmonary artery segment is inconspicuous, but the ascending aorta is relatively prominent (see Figures 25-23 and 25-25). When a congenitally intact ventricular septum causes functional pulmonary atresia (see Figure 25-2A), the ascending aorta is more conspicuous, and the lung fields exhibit the lacy vascular pattern of systemic arterial collaterals (Figure 25-26).21 When the ventricular septal defect is nonrestrictive and pulmonary vascular resistance is low (see Figure 25-2C), pulmonary vascularity is increased, the pulmonary trunk and the left and right atria are enlarged, and a prominent left ventricle is apex-forming (see Figures 25-5A, and 25-7).
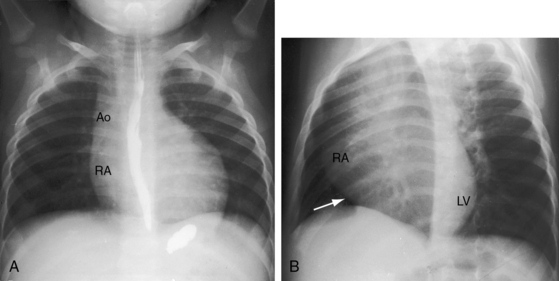
Figure 25-23 A, X-ray from a 10-month-old boy with tricuspid atresia, normally related great arteries, and a restrictive ventricular septal defect (see Figure 25-2B). Pulmonary vascularity is reduced, the ascending aorta (Ao) is prominent, the main pulmonary artery segment is inconspicuous, the dilated right atrium (RA) recedes acutely because of absence of the right ventricle, and a large left ventricle occupies the apex. B, Shallow left anterior oblique projection highlights the hump-shaped appearance caused by right atrial enlargement (RA) in the absence of a right ventricle (arrow). (LV = left ventricle.)
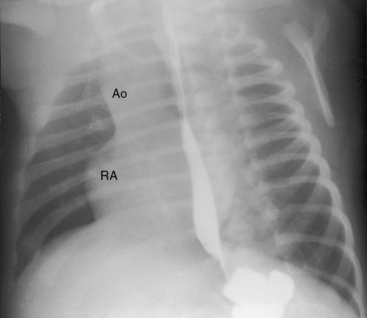
Figure 25-24 Left anterior oblique x-ray from a 4-month-old boy with tricuspid atresia, normally related great arteries, and a restrictive ventricular septal defect. The right atrium (RA) is distinctively hump-shaped. The appearance is highlighted by the prominent ascending aorta (Ao). (Also see Figure 25-23.)
The x-ray in tricuspid atresia with complete transposition of the great arteries, a nonrestrictive ventricular septal defect, and low pulmonary vascular resistance (see Figure 25-3C) is characterized by increased pulmonary vascularity, enlargement of the left and right atria, and a prominent apex-forming left ventricle. The vascular pedicle is narrow because the great arteries are transposed. The x-ray resembles uncomplicated complete transposition of the great arteries with analogous pulmonary vascular resistance (see Chapter 27). The left cardiac border is straight if there is left-sided juxtaposition of the atrial appendages. When pulmonary vascular resistance is increased, the lungs are oligemic and the heart size is normal or nearly so, but the right cardiac border may retain its hump-shaped contour even though the right ventricle is not rudimentary (see Figure 25-8).15
The x-ray in tricuspid atresia with transposition of the great arteries and pulmonary stenosis (Figures 25-3B, and 25-27) shows normal or reduced pulmonary vascularity, a prominent right atrium, a convex left ventricle, and a narrow vascular pedicle because of the transposed great arteries.
Echocardiogram
Echocardiography with color flow imaging and Doppler interrogation establishes the diagnosis of tricuspid atresia, the positions of the great arteries, the condition of the ventricular septum, and the nature of the interatrial communication (Figures 25-28 and 25-29 and Video 25-1).8,67 Computed tomography provides similar information.68 An echodense band produced by fibro-fatty tissue in the right atrioventricular groove represents absence of the right AV connection that characterizes tricuspid atresia (see Figures 25-28 and 25-29). When the great arteries are not transposed, the right ventricular cavity is small, the ventricular septal defect is restrictive, and the left ventricle is moderately enlarged (see Figure 25-28). When the interatrial communication is restrictive, the right atrium is enlarged and the atrial septum is shifted to the left. When the great arteries are transposed and pulmonary resistance is low, echocardiography identifies a nonrestrictive ventricular septal defect, a well-formed right ventricle, enlarged right and left atria, and a dilated volume-overloaded left ventricle (see Figure 25-29). Structural abnormalities of the mitral valve can be characterized.25 Color flow mapping with Doppler interrogation establishes the size of the interatrial communication and the condition of the ventricular septum. When the great arteries are transposed, continuous wave Doppler scan interrogates the left ventricular outflow tract for the presence and degree of pulmonary stenosis.
1 Rashkind W.J. Tricuspid atresia: a historical review. Pediatr Cardiol. 1982;2:85-88.
2 Kuhne M. Uber zwei falle kongenitaler atresia des ostium venosum dextrum. Jahrb Kinderheild Physi Erziehung. 1906;63:225.
3 Anderson R.H., Rigby M.L. The morphologic heterogeneity of tricuspid atresia. Int J Cardiol. 1987;16:67-73.
4 Anderson R.H., Ho S.Y., Rigby M.L. The morphologic variability in atrioventricular valvar atresia. Cardiol Young. 2000;10:32-41.
5 Report of the New England Regional Infant Cardiac Program. Pediatrics. 1980;65:375-461.
6 Sade R.M., Fyfe D.A. Tricuspid atresia: current concepts in diagnosis and treatment. Pediatr Clin North Am. 1990;37:151-169.
7 Mohan J.C., Passey R., Arora R. Echocardiographic spectrum of congenitally unguarded tricuspid valve orifice and patent right ventricular outflow tract. Int J Cardiol. 2000;74:153-157.
8 Martinez R.M., Anderson R.H. Echo-morphological correlates in atrioventricular valvar atresia. Cardiol Young. 2006;16(suppl 1):27-34.
9 Edwards J.E., Burchell H.B. Congenital tricuspid atresia; a classification. Med Clin North Am. 1949;33:1177-1196.
10 Rao P.S. A unified classification for tricuspid atresia. Am Heart J. 1980;99:799-804.
11 Tandon R., Edwards J.E. Tricuspid atresia. A re-evaluation and classification. J Thorac Cardiovasc Surg. 1974;67:530-542.
12 Riker W.L., Potts W.J., Grana L., Miller R.A., Lev M. Tricuspid stenosis or atresia complexes. A surgical and pathologic analysis. J Thorac Cardiovasc Surg. 1963;45:423-433.
13 Rosenquist G.C., Levy R.J., Rowe R.D. Right atrial-left ventricular relationships in tricuspid atresia: position of the presumed site of the atretic valve as determined by transillumination. Am Heart J. 1970;80:493-497.
14 Wenink A.C., Ottenkamp J. Tricuspid atresia. Microscopic findings in relation to “absence” of the atrioventricular connexion. Int J Cardiol. 1987;16:57-73.
15 Dick M., Fyler D.C., Nadas A.S. Tricuspid atresia: clinical course in 101 patients. Am J Cardiol. 1975;36:327-337.
16 Freedom R.M., Rowe R.D. Aneurysm of the atrial septum in tricuspid atresia: diagnosis during life and therapy. Am J Cardiol. 1976;38:265-267.
17 Reder R.F., Yeh H.C., Steinfeld L. Aneurysm of the interatrial septum causing pulmonary venous obstruction in an infant with tricuspid atresia. Am Heart J. 1981;102:786-789.
18 Rao P.S. Natural history of the ventricular septal defect in tricuspid atresia and its surgical implications. Br Heart J. 1977;39:276-288.
19 Rao P.S., Sissman N.J. Spontaneous closure of physiologically advantageous ventricular septal defects. Circulation. 1971;43:83-90.
20 Roberts W.C., Morrow A.G., Mason D.T., Braunwald E. Spontaneous closure of ventricular septal defect, anatomic proof in an adult with tricuspid atresia. Circulation. 1963;27:90-94.
21 Kieffer S.A., Carey L.S. Tricuspid atresia with normal aortic root: roentgen-anatomic correlation. Radiology. 1963;80:605-615.
22 Patel R., Fox K., Taylor J.F., Graham G.R. Tricuspid atresia. Clinical course in 62 cases (1967–1974). Br Heart J. 1978;40:1408-1414.
23 Jordan J.C., Sanders C.A. Tricuspid atresia with prolonged survival. A report of two cases with a review of the world literature. Am J Cardiol. 1966;18:112-119.
24 Deanfield J.E., Tommasini G., Anderson R.H., Macartney F.J. Tricuspid atresia: analysis of coronary artery distribution and ventricular morphology. Br Heart J. 1982;48:485-492.
25 Ottenkamp J., Wenink A.C. Anomalies of the mitral valve and of the left ventricular architecture in tricuspid valve atresia. Am J Cardiol. 1989;63:880-881.
26 Litovsky S., Choy M., Park J., et al. Absent pulmonary valve with tricuspid atresia or severe tricuspid stenosis: report of three cases and review of the literature. Pediatr Dev Pathol. 2000;3:353-366.
27 Rudolph A.M., Heymann M.A., Spitznas U. Hemodynamic considerations in the development of narrowing of the aorta. Am J Cardiol. 1972;30:514-525.
28 Gyepes M.T., Marcano B.A., Desilets D.T. Tricuspid atresia, transposition, and coatation of the aorta. Radiology. 1970;97:633-636.
29 Becker A.E., Becker M.J. Juxtaposition of atrial appendages associated with normally oriented ventricles and great arteries. Circulation. 1970;41:685-688.
30 Charuzi Y., Spanos P.K., Amplatz K., Edwards J.E. Juxtaposition of the atrial appendages. Circulation. 1973;47:620-627.
31 Deutsch V., Shem-Tov A., Yahini J.H., Neufeld H.N. Juxtaposition of atrial appendages: angiocardiographic observations. Am J Cardiol. 1974;34:240-244.
32 Rao P.S. Left to right atrial shunting in tricuspid atresia. Br Heart J. 1983;49:345-349.
33 La Corte M.A., Dick M., Scheer G., La Farge C.G., Fyler D.C. Left ventricular function in tricuspid atresia. Angiographic analysis in 28 patients. Circulation. 1975;52:996-1000.
34 Nishioka K., Kamiya T., Ueda T., et al. Left ventricle volume characteristics in children with tricuspid atresia before and after surgery. Am J Cardiol. 1981;47:1105-1110.
35 Lin A.E., Rosti L. Tricuspid atresia in sibs. J Med Genet. 1998;35:1055-1056.
36 Kumar A., Victorica B.E., Gessner I.H., Alexander J.A. Tricuspid atresia and annular hypoplasia: report of a familial occurrence. Pediatr Cardiol. 1994;15:201-203.
37 Weigel T.J., Driscoll D.J., Michels V.V. Occurrence of congenital heart defects in siblings of patients with univentricular heart and tricuspid atresia. Am J Cardiol. 1989;64:768-771.
38 Bonnet D., Fermont L., Kachaner J., et al. Tricuspid atresia and conotruncal malformations in five families. J Med Genet. 1999;36:349-350.
39 Svensson E.C., Huggins G.S., Lin H., et al. A syndrome of tricuspid atresia in mice with a targeted mutation of the gene encoding Fog-2. Nat Genet. 2000;25:353-356.
40 Patterson W., Baxley W.A., Karp R.B., Soto B., Bargeron L.L. Tricuspid atresia in adults. Am J Cardiol. 1982;49:141-152.
41 Breisch E.A., Wilson D.B., Laurenson R.D., Mazur J.H., Bloor C.M. Tricuspid atresia (type Ia): survival to 21 years of age. Am Heart J. 1983;106:149-151.
42 Voci G., Diego J.N., Shafia H., Alavi M., Ghusson M., Banka V.S. Type Ia tricuspid atresia with extensive coronary artery abnormalities in a living 22 year old woman. J Am Coll Cardiol. 1987;10:1100-1104.
43 Beaver T.R., Shroyer K.R., Muro-Cacho C.A., Miller G.J., Blount S.G. Survival to age 65 years with tricuspid and pulmonic valve atresia. Am J Cardiol. 1988;62:165-166.
44 Brown J.W., Heath D., Morris T.L., Whitaker W. Tricuspid atresia. Br Heart J. 1956;18:499-518.
45 Cooley R.N., Sloan R.D., Hanlon C.R., Bahnson H.T. Angiocardiography in congenital heart disease of cyanotic type. II. Observations on tricuspid stenosis or atresia with hypoplasia of the right ventricle. Radiology. 1950;54:848-868.
46 Hatjis C.G., Gibson M., Capeless E.L., Auletta F.J., Anderson G.G. Pregnancy in a patient with tricuspid atresia. Am J Obstet Gynecol. 1983;145:114-115.
47 Hart A.S., Vacek J.L. Prolonged survival in tricuspid atresia with Eisenmenger’s physiology. Clin Cardiol. 1984;7:555-556.
48 Patel M.M., Overy D.C., Kozonis M.C., Hadley-Fowlkes L.L. Long-term survival in tricuspid atresia. J Am Coll Cardiol. 1987;9:338-340.
49 Shariatzadeh A.N., King H., Girod D., Shumacker H.B. Tricuspid atresia. A review of 68 cases. Chest. 1977;71:538-540.
50 Fontana R., Edwards J. Congenital cardiac disease: a review of 357 cases studied pathologically. Philadelphia: W.B. Saunders; 1962.
51 Karalis D.G., Chandrasekaran K., Victor M.F., Mintz G.S. Prolonged survival despite severe cyanosis in an adult with right ventricular hypoplasia and atrial septal defect. Am Heart J. 1990;120:701-703.
52 Steelman R.B., Perloff J.K., Cochran P.T., Ronan J.A. Congenital stenosis of the pulmonic and tricuspid valves. Clinical, hemodynamic and angiographic observations in a 20 year old woman. Am J Med. 1973;54:788-792.
53 Jones R.N., Niles N.R. Spinnaker formation of sinus venosus valve. Case report of a fatal anomaly in a ten-year-old boy. Circulation. 1968;38:468-473.
54 Freedom R.M., Gerald P.S. Congenital cardiac disease and the “cat eye” syndrome. Am J Dis Child. 1973;126:16-18.
55 Folger G.M.Jr. Systemic hypoperfusion in a neonate with tricuspid atresia and transposition of the great arteries. Similarity to the hypoplastic left heart syndrome. Angiology. 1980;31:721-724.
56 Perloff J.K. Physical examination of the heart and circulation, 4th ed. Shelton, Connecticut: People’s Medical Publishing House; 2009.
57 Davachi F., Lucas R.V.Jr., Moller J.H. The electrocardiogram and vectorcardiogram in tricuspid atresia. Correlation with pathologic anatomy. Am J Cardiol. 1970;25:18-27.
58 Guller B., Titus J.L., Dushane J.W. Electrocardiographic diagnosis of malformations associated with tricuspid atresia: correlation with morphologic features. Am Heart J. 1969;78:180-188.
59 Somlyo A.P., Halloran K.H. Tricuspid atresia. An electrocardiographic study. Am Heart J. 1962;63:171-179.
60 Reddy S.C., Zuberbuhler J.R. Images in cardiovascular medicine. Himalayan P-waves in a patient with tricuspid atresia. Circulation. 2003;107:498.
61 Gamboa R., Gersony W.M., Nadas A.S. The electrocardiogram in tricuspid atresia and pulmonary atresia with intact ventricular septum. Circulation. 1966;34:24-37.
62 Rihl J., Terplan K., Weiss F. Uber einen fall von agenesie der tricuspidalklappe. Medizinische Klinik. 1923;25:1543.
63 Taussig H.B. The clinical and pathological findings in congenital malformations of the heart due to defective development of the right ventricle associated with tricuspid atresia or hypoplasia. Bulletin of the Johns Hopkins Hospital. 1936:435.
64 Bharati S., Lev M. The conduction system in tricuspid atresia with and without regular (d-) transposition. Circulation. 1977;56:423-429.
65 Perloff J.K., Roberts N.K., Cabeen W.R.Jr. Left axis deviation: a reassessment. Circulation. 1979;60:12-21.
66 Schatz J., Krongrad E., Malm J.R. Left anterior and left posterior hemiblock in tricuspid atresia and transposition of the great vessels: observations and electrocardiographic nomenclature and electrophysiologic mechanisms. Circulation. 1976;54:1010-1013.
67 Orie J.D., Anderson C., Ettedgui J.A., Zuberbuhler J.R., Anderson R.H. Echocardiographic-morphologic correlations in tricuspid atresia. J Am Coll Cardiol. 1995;26:750-758.
68 Mochizuki T., Ohtani T., Higashino H., et al. Tricuspid atresia with atrial septal defect, ventricular septal defect, and right ventricular hypoplasia demonstrated by multidetector computed tomography. Circulation. 2000;102:E164-E165.