CHAPTER 93 Treatment Applications of Cortical Stimulation
Although some attempts to use cortical stimulation for the treatment of neurological disorders were made in the latter half of the 20th century, only recently has chronic MCS been investigated seriously as a surgical treatment of both chronic pain and medically refractory movement disorders. Before the advent of viable chronically implanted stimulation systems, disorders of central nervous system function were treated by ablation or extirpation of the offending tissue. In 1909, Horsley reported suppression of athetosis by resection of the motor cortex.1 Interestingly, he localized the upper limb region of M1 by direct cortical stimulation. In the 1930s and 1940s, Bucy extirpated the motor cortex to treat PD and other tremor disorders.1,2 In 1954, Penfield and Jasper resected a patient’s primary sensory cortex (S1) to treat burning pain but did not achieve complete resolution of symptoms until they also resected the precentral gyrus.3 Later that same decade, White and Sweet reported minimal success treating refractory pain with postcentral resection.4
The contemporary history of nervous system stimulation as a treatment modality perhaps begins with Heath, who in 1954 stimulated the septal area with the goal of activating pleasure centers and alleviating pain.5 Twenty-five years later, Woolsey and colleagues demonstrated inhibition of tremor and rigidity in PD patients via precentral stimulation,6 although permanent implantation of electrodes was not attempted. It was not until the 1970s, when Mundinger stimulated the sensory thalamic nuclei to treat spasticity and athetosis, that chronic stimulation of the brain was used to treat movement disorders.7,8 Since then, deep brain stimulation (DBS) at several sites, including the thalamus,9 cingulum,10 periventricular and periaqueductal gray areas,11 and more recently the globus pallidus pars interna12 and subthalamic nucleus,13 has been attempted to treat a variety of functional brain disorders. During the mid-1980s, Hosobuchi implanted electrodes subcortically in the somatosensory region in 76 chronic deafferentation pain patients and noted beneficial results in 44.14 In 1985 and 1991, Tsubokawa and colleagues reported their results with chronic stimulation of the M1 region for the treatment of post-thalamic stroke pain.15,16 In 1998, Ngyuen and coauthors reported using stimulation of M1 to treat PD.17 A MEDLINE review of articles from 1991 to 2008 revealed 512 cases in which MCS was used for the treatment of pain (422 cases),15,18–58 poststroke rehabilitation (9 cases),19,59,60 or movement disorders (84 cases), 22 of which were combined with DBS.17,61–73 Some studies included previously reported cases from earlier references that the authors tried to differentiate but may have missed.
As of May 2008, Tsubokawa and associates at Nihon University had the largest published experience with MCS, 88 cases in all.29,33,65,66 Their work included comparisons of DBS and spinal cord stimulation (SCS) to MCS, predominantly for the treatment of chronic pain and movement disorders. They reported that 59% of patients who underwent DBS or MCS for involuntary movements resulting from stroke experienced some benefit, mostly relief of hemichorea and resting tremor.30 Nineteen percent of patients who underwent MCS primarily for pain control also showed improvement in their movement problems, which consisted of hemiparesis predominantly. The results for treating phantom limb pain were less promising.33 Only 6 of 19 patients had good pain relief from SCS alone. Ten of those who failed SCS eventually underwent ventrocaudal thalamic DBS. Of these, 6 patients had good long-term pain control. Of another 5 patients who failed SCS, only 1 experienced greater pain relief when subsequently treated with MCS. Nevertheless, this patient still experienced only moderate pain reduction. In another study of 12 patients with various brain lesions (6 with thalamic stroke, 3 with stroke in the posterior limb of the internal capsule, 1 with pontine stroke, 1 with multiple sclerosis, and 1 with postrhizotomy pain), 6 achieved “excellent” outcomes (defined as 100% pain improvement) at 1 year, with 5 of 11 patients maintaining this response after 2 years.15,51 Three patients whose responses were initially categorized as “good” (60% to 80% improvement) were recategorized as either “fair” (40% to 60% improvement) or “poor” (<40% improvement) at 1 year. In 1998, Katayama and coauthors reported that although satisfactory pain control could be achieved in 23 of 31 poststroke patients initially, at 2 years pain control was still satisfactory in only 8 of these initial 23 patients.31
Several groups in Italy have developed a moderate experience with the use of MCS primarily for the treatment of movement disorders.24,39,61,62,64,68,71 Early results from these studies were promising, but as time progressed the benefits diminished. In a study sponsored by the Italian Neurosurgical Society,68 10 patients were monitored for 3 to 30 months after MCS for PD. All but 1 patient were ineligible for DBS because of age, findings on magnetic resonance imaging (MRI), or neurocognitive difficulties. The mean duration of disease was 12.4 years. Three patients improved less than 25%, 6 improved 25% to 50%, and 1 improved more than 50% as measured with a global rating scale. The stimulation parameters were as follows: 2.5 to 6 V, 150 to 180 µsec, and 25 to 40 Hz. Over time, these beneficial effects were lost, as reported in later presentations.74,75
Programming and Complications
Historically, stimulation parameters for the treatment of chronic pain have varied widely. Stimulation amplitudes have ranged from a minimum of 0.5 V to the device’s maximal output of 10.5 V, stimulation frequencies from 5 to 210 Hz, and pulse widths from 1 to 500 µsec.* The most common adverse effect is induction of seizures, the majority of which occur during the initial testing phases. Hardware failure and infection are the second and third most likely complications, respectively. Both Myerson and colleagues35 and Nguyen and associates77 reported postoperative hematomas; one was asymptomatic and one required surgical intervention. Three groups have described subdural instead of epidural electrode placement.47,53,54 In one of these reports two of nine patients suffered hemorrhages, one of which was fatal.47 The other patient was left in a persistent vegetative state.
Methods
Surgical and Cortical Mapping Technique
Although the M1 region can be accessed via bur hole,24,39,40,64,68,74,75 we prefer a small craniotomy, which allows us to perform detailed electrophysiologic mapping of the region and to suture the stimulating electrode to the dura so that it remains in place. The operation is performed with the patient supine. The anesthetic technique must be tailored so that neurophysiologic mapping can be performed. We prefer a complete total intravenous anesthetic protocol using a continuous infusion of propofol combined with either fentanyl or remifentanil. Standard doses are in the range of 75 to 150 µg/kg/min of propofol and 0.05 to 0.5 µg/kg/min of fentanyl or remifentanil. Inhaled agents are avoided, particularly nitrous oxide, because of their detrimental effects on motor evoked potentials. No muscle relaxants are used except during induction/intubation.
We do not use three-point cranial fixation because rigid fixation is unnecessary and could cause injury to the patient if a seizure is induced during cortical stimulation/mapping. To center the incision over the M1 region, the midpoint from nasion to inion is determined,* and a curved incision extending from approximately 1 cm behind this midpoint toward the anterior margin of the tragus is marked on the scalp before preparing and draping the surgical field (Fig. 93-1). This incision allows a 5- to 6-cm craniotomy, which can be adjusted superiorly or inferiorly along the convexity to map the hand or face, respectively. Even though the craniotomy lies mainly above the superior temporal line, the superior edge of the temporalis muscle may be detached when access to the facial region of M1 is required. One may leave a cuff of temporalis fascia for reattachment of the muscle during closure.
Some surgical teams advocate using functional MRI (fMRI) to localize M1 preoperatively.37,40,43,50 In our experience, these studies provide no information that cannot be obtained by intraoperative mapping; however, validation of fMRI might one day obviate the need for a small craniotomy or intraoperative mapping, or both. Nevertheless, the potential for target inaccuracies as a result of brain shifting, the desire to better secure the electrode to the dura, and the potential for misrepresentation of functional activity on fMRI have kept us from adopting this technique to date.
Intraoperative Neurophysiologic Mapping
We use both somatosensory and motor mapping to determine the course of the central sulcus and the position of the M1 region. Somatosensory testing consists of placing the paddle electrode (Resume, Medtronic, Inc., Minneapolis, MN) on the dura in a variety of orientations, mostly perpendicular to the suspected precentral gyrus. Median or ulnar nerve somatosensory evoked potentials (SSEPs), or both, are then performed by using a 20- to 50-mA, 100-µsec monopolar square pulse at a rate of 4.32 Hz. The SSEPs are recorded from the lead in both a bipolar (e.g., contacts 0-1, 1-2, and 2-3 for a 4-contact lead) and monopolar (all referenced to the 10-20 location of Fz) recording montage. The central sulcus is defined as the point at which the N20 response phase reverses polarity (Fig. 93-2). Mapping is performed epidurally over the entire exposed area and for short distances under the bone if needed. Typically, 7 to 10 recordings are obtained, which takes no more than 10 minutes of operative time. Early experience with this technique was gained with use of the 4-contact Resume lead (Medtronics, Minneapolis, MN); however, recently, we have used a 16-contact lead connected to a rechargeable implantable pulse generator (IPG) system (Precision Plus, Boston Scientific, Niatick, MA), a constant-current system that in our initial experience has yielded quicker onset of pain relief. Long-term results with this system still need to be evaluated.
In our experience, the mean stimulation amplitude required to generate an evoked response is 13.0 ± 4.2 mA (range, 7 to 22.4 mA). Stimulation is halted when the first electromyographic response is noted. Figure 93-3 displays electromyographic recordings taken from the extensor carpi ulnaris and abductor pollicis brevis muscles with this technique. We reiterate the importance of anesthetic technique for successful performance of physiologic mapping.
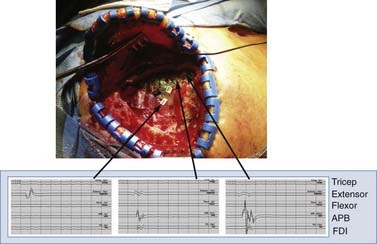
FIGURE 93-3 Use of cortical mapping to determine the location of M1 subregions. The electromyogram from muscles in the upper extremity is shown after stimulation with a ball-tipped electrode in three locations on the dura. The tracing at the far left shows activation of the extensor muscles in the forearm, with the other two tracings showing activation of the abductor pollicis brevis (APB). This technique corroborates the SSEP method in Figure 93-2 and helps determine more precisely the underlying thresholds for individual muscle groups. Extensor, extensor carpi ulnaris; FDI, first dorsal interosseus; Flexor, flexor carpi ulnaris.
Programming the Implanted Device
The implanted stimulator is activated within 24 hours of surgery. During the initial programming session we evaluate the effects of cathodal stimulation via each contact in a monopolar configuration (i.e., referenced to the IPG) by using pulses 210 µsec in duration at 130 Hz. The amplitude is raised slowly to 4.0 V while the patient is assessed for adverse motor and sensory phenomena. The use of cathodal stimulation might be questioned given that anodal stimulation administered via transcranial electrical and magnetic stimulation generates D waves and motor evoked potentials at lower intensities.78 Our use of cathodal stimulation is dictated by the design of contemporary implantable stimulators. Moreover, work by Hanajima and coworkers has revealed that chronic cathodal stimulation activates neurons in the motor cortex at lower stimulation intensities than does anodal stimulation.79
In our four PD patients, we stimulated M1 via contacts 1 and 2 referenced to the IPG at an amplitude of 3.0 V. If the patient could not tolerate these settings, either contact 3 or 0 alone was used instead. In all cases, at least two contacts were activated at the initial programming session. If minimal benefit was noted from these initial settings, additional contacts were activated. If monopolar stimulation failed, one contact (either contact 0 or contact 3) was set as the cathode with the other three contacts set as the anode. We investigated additional parameter adjustments, including increasing the stimulation pulse width to 410 µsec, increasing the amplitude to 4.5 V, or changing the frequency to either 185 or 80 Hz. Table 93-1 shows the stimulation parameters relative to the surgical implant date.
Clinical Results
Through July 2008, we had performed MCS on 16 patients (Table 93-2). Among the 4 PD patients, complications were restricted to one intraoperative seizure and one infection, which required device removal. At the time of explantation this patient had improved approximately 50% in comparison to baseline but regressed significantly when the device was removed. A new device was implanted 1 year later, again with nearly 50% improvement. Two PD patients (MCS1 and MCS2) experienced 20% to 30% reductions in their medication requirement at 3 months, but patient MCS2 required more medication at the 6- and 12-month time points. One patient (MCS4) required 37% more dopaminergic medications postoperatively.
The motor subscores of the Unified Parkinson’s Disease Rating Scale (UPDRS-III) for our four PD patients are given in Tables 93-3 and 93-4. A significant improvement in the UPDRS-III scores is discerned up to 6 months postoperatively, after which motor function appears to regress. Two-year follow-up in two patients (MCS2 and MCS3) showed a return to their preoperative baseline as measured with the UPDRS-III while the patients were taking medications. Both patients noted reduced motor function. In contrast, patient MCS3 was wheelchair and bed bound after his generator lost power and is now able to feed and dress himself and walk with assistance with a new generator.
In our series of patients with neuropathic pain the results are even less consistent. Among the nine patients in whom pain was the dominant symptom, six experienced poststroke pain, one (P3) had atypical head pain secondary to trauma, one (P9) had a complex regional pain syndrome (CRPS) secondary to brachial plexus avulsion, and one had CRPS of the wrist. Of the six stroke patients, four suffered from both pain and a movement disorder. One of these four (P7) received no benefit from MCS. Two others (P2 and P4) experienced varying degrees of benefit. Patient P2 has good control of her upper extremity and face pain, but less pain control in her leg and minimal control of sensations of a “third limb.” Patient P4 has fair control of both her pain and sensation of an “internal” tremor. The patient with atypical head pain (P3) has experienced no relief in his scalp contralateral to the stimulation but is receiving benefit in his ipsilateral scalp region, which is not easily explained but may be related to stimulation of the trigeminal branches innervating the dura.80 In addition, this patient found that his posttraumatic seizures improved with MCS, although it should be noted that he had no EEG seizures during a 24-hour EEG evaluation. A wide range of stimulation parameters have been used in these patients, with amplitudes ranging from 2.2 to 4.2 V, pulse widths from 120 to 330 µsec, and frequencies from 50 to 130 Hz. Stimulation in all patients started with monopolar configurations, but bipolar settings were often used with more anodal contacts.
Conclusion
It is important to compare MCS directly with DBS, even at this early stage of development, because DBS is now recognized as the most effective surgical therapy for medically refractory movement disorders. In light of the documented efficacy of DBS, the primary reason to investigate MCS as a treatment option is the potential to reduce neurological complications by eliminating the need to penetrate the brain parenchyma. Most published series suggest that the risk for hemorrhage related to DBS is less than 2%, with a range of 0.2% to 9.5%.81 Additional advantages of MCS include the ability to perform the surgery under general anesthesia, elimination of the stereotactic head frame, and the speed with which M1 can be localized intraoperatively. Consequently, if MCS were shown to be as effective as DBS for treating the cardinal symptoms of PD, it would become the preferred surgical option.
In contrast to previous reports,68 our results suggest that the benefits of MCS for PD are more modest and short-lived than the responses generated by DBS. Nevertheless, 2 of our patients (M3 and M4) still derive benefit from MCS years after implantation. One other preliminary study of MCS for PD in which the stimulating electrodes were placed subdurally found benefit only for tremor.82 Akinesia, rigidity, and gait were not improved. It must be remembered that to date, only 27 patients with movement disorders have undergone MCS.62,68,82 Therefore, more data are required to determine whether MCS is a viable option for patients with movement disorders who cannot undergo DBS, whether for general medical or neurocognitive reasons. Moreover, the optimal technique for performing MCS remains to be determined because electrode orientation (perpendicular or parallel to M1) and stimulation parameters (electrode polarity, frequency, pulse width, and so on) are matters of great debate.
Another disadvantage of MCS is a delay in efficacy, which in some cases has been many months. Drouot and colleagues hypothesized that secondary messengers or long-term potentiation may account for the delayed response.83 One may also hypothesize that chronic MCS alters not only firing patterns in the basal ganglia but also interactions between the pyramidal and extrapyramidal systems, which requires both the thalamocorticobasal ganglionic and corticocortical loops to adjust to these new signal patterns.
Amassian VE, Stewart M, Quirk GJ, et al. Physiological basis of motor effects of a transient stimulus to cerebral cortex. Neurosurgery. 1987;20:74-93.
Arle JE, Apetauerova D, Zani J, et al. Motor cortex stimulation for Parkinson’s disease:12 month follow-up in 4 patients. J Neurosurg. 2008;109:133-139.
Canavero S, Bonicalzi V, Paolotti R, et al. Therapeutic extradural cortical stimulation for movement disorders: a review. Neurol Res. 2003;25:118-122.
Canavero S, Paolotti R, Bonicalzi V, et al. Extradural motor cortex stimulation for advanced Parkinson disease. J Neurosurg. 2002;97:1208-1211.
Franzini A, Ferroli P, Dones I, et al. Chronic motor cortex stimulation for movement disorders: a promising perspective. Neurol Res. 2003;25:123-126.
Garcia-Larrea L, Peyron R, Mertens P, et al. Electrical stimulation of motor cortex for pain control: a combined PET-scan and electrophysiological study. Pain. 1999;83:259-273.
Hanajima R, Ashby P, Lang AE, et al. Effects of acute stimulation through contacts placed on the motor cortex for chronic stimulation. Clin Neurophysiol. 2002;113(5):635-641.
Katayama Y, Fukaya C, Yamamoto T. Control of post-stroke involuntary and voluntary movement disorders with deep brain or epidural cortical stimulation. Stereotact Funct Neurosurg. 1997;69:73-79.
Katayama Y, Oshima H, Fukaya C, et al. Control of post-stroke movement disorders using chronic motor cortex stimulation. Acta Neurochir Suppl. 2001;79:89-92.
Katayama Y, Tsubokawa T, Yamamoto T. Chronic motor cortex stimulation for central deafferentation pain: experience with bulbar pain secondary to Wallenberg syndrome. Stereotact Funct Neurosurg. 1994;62:295-299.
Kleiner-Fisman G, Fisman DN, Kahn FI, et al. Motor cortical stimulation for parkinsonism. Arch Neurol. 2003;60:1554-1558.
Nguyen JP, Lefaucheur JP, Decq P, et al. Chronic motor cortex stimulation in the treatment of central and neuropathic pain. Correlations between clinical, electrophysiological and anatomical data. Pain. 1999;82:245-251.
Nuti C, Peyron R, Garcia-Larrea L, et al. Motor cortex stimulation for refractory neuropathic pain: four-year outcome and predictors of efficacy. Pain. 2005;118:43-52.
Pagni C, Altibrandi MG, Bentivoglio A, et al. Extradural motor cortex stimulation (EMCS) for Parkinson’s disease. History and first results by the study group of the Italian neurosurgical society. Acta Neurochir Suppl. 2005;93:113-119.
Saitoh Y, Shibata M, Hirano S, et al. Motor cortex stimulation for central and peripheral deafferentation pain. J Neurosurg. 2000;92:150-155.
Saitoh Y, Shibata M, Sanada Y, et al. Motor cortex stimulation for phantom limb pain. The Lancet. 1999;353:212.
Tsubokawa T, Katayama Y, Yamamoto T, et al. Chronic motor cortex stimulation in patients with thalamic pain. J Neurosurg. 1993;78:393-401.
Tsubokawa T, Katayama Y, Yamamoto T, et al. Chronic motor cortex stimulation for the treatment of central pain. Acta Neurochir Suppl. 1991;52:137-139.
1 Gabriel EM, Nashold BS. Evolution of neuroablative surgery for involuntary movement disorders: an historical review. Neurosurgery. 1998;42:575-591.
2 Vilensky JA, Gilman S. Using extirpations to understand the human motor cortex: Horsley, Foerster, and Bucy. Arch Neurol. 2003;60:446-451.
3 Lende RA, Kirsch WM, Druckman R. Relief of facial pain after combined removal of precentral and postcentral cortex. J Neurosurg. 1971;34:537-543.
4 White JC, Sweet WH. Pain: Its Mechanisms and Neuro-surgical Control. Springfield, IL: Charles C Thomas; 1955.
5 Heath RG. Psychiatry. Annu Review Med. 1954;5:223-236.
6 Woolsey CN, Erickson TC, Gilson WE. Localization in somatic sensory and motor areas of human cerebral cortex as determined by direct recording of evoked potentials and electrical stimulation. Neurosurgery. 1979;51:476-506.
7 Mundinger F. New stereotactic treatment of spasmodic torticollis with a brain stimulation system [author’s transl]. Med Klin. 1977;72:1982-1986.
8 Siegfried J. Deep brain stimulation for movement disorders. In: Gildenberg PH, Tasker R, editors. A Textbook of Stereotactic and Functional Neurosurgery. Philadelphia: McGraw-Hill; 1996:1081-1086.
9 Yamamoto T, Katayama Y, Obuchi T, et al. Thalamic sensory relay nucleus stimulation for the treatment of peripheral deafferentation pain. Stereotact Funct Neurosurg. 2006;84:180-183.
10 Spooner J, Yu H, Kao C, et al. Neuromodulation of the cingulum for neuropathic pain after spinal cord injury. Case report. J Neurosurg. 2007;107:169-172.
11 Owen SL, Green AL, Nandi DD, et al. Deep brain stimulation for neuropathic pain. Acta Neurochir. 2007;97:111-116.
12 Gross C, Rougier A, Guehl D, et al. High-frequency stimulation of the globus pallidus internalis in Parkinson’s disease: a study of seven cases. J. Neurosurg. 1997;87:491-498.
13 Krack P, Limousin P, Benabid AL, et al. Chronic simulation of subthalamic nucleus improves levodopa-induced dyskinesias in Parkinson’s disease. Lancet. 1997;350:1676.
14 Hosobuchi Y. Subcortical electrical stimulation for control of intractable pain in humans. Report of 122 cases (1970-1984). J Neurosurg. 1986;64:543-553.
15 Tsubokawa T, Katayama Y, Yamamoto T, et al. Chronic motor cortex stimulation for the treatment of central pain. Acta Neurochir Suppl. 1991;52:137-139.
16 Tsubokawa T. Chronic stimulation of deep brain structures for treatment of chronic pain. In: Tasker RR, editor. Neurosurgery State of the Arts Review, vol 2. Stereotactic Surgery. Philadelphia: Hanley and Belfus; 1985:253-255.
17 Nguyen JP, Lefaucher JP, Le Guerinel C, et al. Improvement of action tremor by chronic cortical stimulation. Mov Disord. 1998;13:84-88.
18 Pascual-Leone A, Wagner T. A brief summary of the history of noninvasive brain stimulation. Annu Rev Biomed Eng. 2007;9:527-565.
19 Brown JA, Lutsep HL, Weinand M, et al. Motor cortex stimulation for the enhancement of recovery from stroke: a prospective, multicenter safety study. Neurosurgery. 2006;58:464-473.
20 Canavero S, Bonicalzi V, Castellano G, et al. Painful supernumerary phantom arm following motor cortex stimulation for central post stroke pain. J Neurosurg. 1999;91:121-122.
21 Canavero S, Bonicalzi V. Therapeutic extradural cortical stimulation for central and neuropathic pain: a review. Clin J Pain. 2002;18:48-55.
22 Carroll D, Joint C, Maartens N, et al. Motor cortex stimulation for chronic neuropathic pain: a preliminary study of 10 cases. Pain. 2000;84:431-437.
23 Ebel H, Tronnier V, Boker D, et al. Chronic precentral stimulation in trigeminal neuropathic pain. Acta Neurochir. 1996;138:1300-1306.
24 Fanzini A, Ferroli P, Servello D, et al. Reversal of thalamic hand syndrome by long-term motor cortex stimulation. J Neurosurg. 2000;93:873-875.
25 Fujii M, Ohmoto Y, Kitahara T, et al. Motor cortex stimulation therapy in patients with thalamic pain. No Shinkei Geka. 1997;25:315-319.
26 Garcia-Larrea L, Peyron R, Mertens P, et al. Electrical stimulation of motor cortex for pain control: a combined PET-scan and electrophysiological study. Pain. 1999;83:259-273.
27 Herregodts P, Stadnik F, De Ridder F, et al. Cortical stimulation for central neuropathic pain: 3-D surface MRl for easy determination of the motor cortex. Acta Neurochir Suppl. 1995;64:132-135.
28 Hosobuchi Y. Motor Cortex Stimulation for Control of Central Deafferentation Pain. Electrical and Magnetic Stimulation of the Brain and Spinal Cord. New York: Raven Press; 1993.
29 Katayama Y, Tsubokawa T, Yamamoto T. Chronic motor cortex stimulation for central deafferentation pain: experience with bulbar pain secondary to Wallenberg syndrome. Stereotact Funct Neurosurg. 1994;62:295-299.
30 Katayama Y, Fukaya C, Yamamoto T. Control of post-stroke involuntary and voluntary movement disorders with deep brain or epidural cortical stimulation. Stereotact Funct Neurosurg. 1997;69:73-79.
31 Katayama Y, Fukaya C, Yamamoto T. Poststroke pain control by chronic motor cortex stimulation neurological characteristics predicting a favorable response. J Neurosurg. 1998;89:585-591.
32 Katayama Y, Oshima H, Fukaya C, et al. Control of post-stroke movement disorders using chronic motor cortex stimulation. Acta Neurochir Suppl. 2001;79:89-92.
33 Katayama Y, Yamamoto T, Kobayashi K, et al. Motor cortex stimulation for phantom limb pain: a comprehensive therapy with spinal cord and thalamic stimulation. Stereotact Funct Neurosurg. 2001;77:159-161.
34 Mertens P, Nuti C, Sindou M, et al. Precentral cortex stimulation for the treatment of central neuropathic pain. Stereotact Funct Neurosurg. 1999;73:122-125.
35 Meyerson BA, Lindblom U, Linderoth B, et al. Motor cortex stimulation as treatment of trigeminal neuropathic pain. Acta Neurochir Suppl. 1993;58:150-153.
36 Migita K, Uozumi T, Arita K, et al. Transcranial magnetic coil stimulation in patients with central pain. Technique and application. Neurosurgery. 1995;36:1037-1040.
37 Mogilner AY, Rezai AR. Epidural motor cortex stimulation with functional imaging guidance? Neurosurg Focus. 2001;11(3):E4.
38 Nguyen JP, Lefaucheur JP, Decq P, et al. Chronic motor cortex stimulation in the treatment of central and neuropathic pain. Correlations between clinical, electrophysiological and anatomical data. Pain. 1999;82:245-251.
39 Pagni CA, Zeme S, Zenga F, et al. Extradural motor cortex stimulation in advanced Parkinson’s disease: the Turin experience: technical case report. Neurosurgery. 2005;57(Suppl):E402.
40 Pirotte B, Voordecker P, Joffroy F, et al. The Zeiss-MKM system for frameless image-guided approach in epidural motor cortex stimulation for central neuropathic pain. Neurosurg Focus. 2001;11(3):E3.
41 Rainov NG, Fels C, Heidecke V, et al. Epidural electrical stimulation of the motor cortex in patients with facial neuralgia. Clin Neurol Neurosurg. 1997;99:205-209.
42 Rainov NG, Heidecke V. Motor cortex stimulation for neuropathic facial pain. Neurol Res. 2003;25:157-161.
43 Roux FE, Ibarrola D, Tremoulet M, et al. Methodological and technical issues for integrating functional magnetic resonance imaging data in a neuronavigation system. Neurosurgery. 2001;49:1145-1157.
44 Saitoh Y, Shibata M, Sanada Y, et al. Motor cortex stimulation for phantom limb pain. Lancet. 1999;353:212.
45 Saitoh Y, Shibata M, Hirano S, et al. Motor cortex stimulation for central and peripheral deafferentation pain. J Neurosurg. 2000;92:150-155.
46 Saitoh Y, Hirano S, Kato A, et al. Motor cortex stimulation for deafferentation pain. Neurosurg Focus. 2001;11(3):E1.
47 Saitoh Y, Kato A, Ninomiya H, et al. Primary motor cortex stimulation within the central sulcus for treating deafferentation pain. Acta Neurochir Suppl. 2003;87:149-152.
48 Smith H, Joint C, Schlugman D, et al. Motor cortex stimulation for neuropathic pain. Neurosurg Focus. 2000;11(3):E2.
49 Son BC, Kim MC, Moon DE, et al. Motor cortex stimulation in a patient with intractable complex regional pain syndrome type II with hemibody involvement. J Neurosurg. 2003;98:175-179.
50 Sol JC, Casaux J, Roux FE, et al. Chronic motor cortex stimulation for phantom limb pain. Correlations between pain relief and functional imaging studies. Stereotact Funct Neurosurg. 2001;77:172-176.
51 Tsubokawa T, Katayama Y, Yamamoto T, et al. Chronic motor cortex stimulation in patients with thalamic pain. J Neurosurg. 1993;78:393-401.
52 Velasco M, Velasco F, Brito F, et al. Motor cortex stimulation in the treatment of deafferentation pain. I. Localization of the motor cortex. Stereotact Funct Neurosurg. 2002;79:146-167.
53 Tani N, Saitoh Y, Hirata M, et al. Bilateral cortical stimulation for deafferentation pain after spinal cord injury. J Neurosurg. 2004;101:687-689.
54 Nuti C, Peyron R, Garcia-Larrea L, et al. Motor cortex stimulation for refractory neuropathic pain: four-year outcome and predictors of efficacy. Pain. 2005;118:43-52.
55 Rasche D, Ruppolt M, Stippich C, et al. Motor cortex stimulation for long-term relief of chronic neuropathic pain: a 10-year experience. Pain. 2006;121:43-52.
56 Peyron R, Faillenot I, Mertens R, et al. Motor cortex stimulation in neuropathic pain. Correlations between analgesic effect and hemodynamic changes in the brain. A PET study. Neuroimage. 2007;34:310-321.
57 Brown JA, Pilitsis JG. Motor cortex stimulation for central and neuropathic facial pain: a prospective study of 10 patients and observations of enhanced sensory and motor function during stimulation. Neurosurgery. 2005;56:290-297.
58 Kishima H, Saitoh Y, Osaki Y, et al. Motor cortex stimulation in patients with deafferentation pain: activation of the posterior insula and thalamus. J Neurosurg. 2007;107:43-48.
59 Rasche D, Ruppolt M, Stippich C, et al. Motor cortex stimulation for long-term relief of chronic neuropathic pain: a 10-year experience. Pain. 2006;121:43-52.
60 Brown JA, Lutsep H, Cramer SC, et al. Motor cortex stimulation for enhancement of recovery after stroke: case report. Neurol Res. 2003;25:815-818.
61 Canavero S, Paolotti R, Bonicalzi V, et al. Extradural motor cortex stimulation for advanced Parkinson disease. J Neurosurg. 2002;97:1208-1211.
62 Canavero S, Bonicalzi V, Paolotti R, et al. Therapeutic extradural cortical stimulation for movement disorders: a review. Neurol Res. 2003;25:118-122.
63 Drouot X, Oshino S, Jarraya B, et al. Functional recovery in a primate model of Parkinson’s disease following motor cortex stimulation. Neuron. 2004;44:769-778.
64 Franzini A, Ferroli P, Dones I, et al. Chronic motor cortex stimulation for movement disorders: a promising perspective. Neurol Res. 2003;25:123-126.
65 Katayama Y, Fukaya C, Yamamoto T. Control of post-stroke involuntary and voluntary movement disorders with deep brain or epidural cortical stimulation. Stereotact Funct Neurosurg. 1997;69:73-79.
66 Katayama Y, Oshima H, Fukaya C, et al. Control of post-stroke movement disorders using chronic motor cortex stimulation. Acta Neurochir Suppl. 2001;79:89-92.
67 Kleiner-Fisman G, Fisman DN, Kahn FI, et al. Motor cortex stimulation for parkinsonism in multiple system atrophy. Arch Neurol. 2003;60:1554-1558.
68 Pagni C, Altibrandi MG, Bentivoglio A, et al. Extradural motor cortex stimulation (EMCS) for Parkinson’s disease. History and first results by the study group of the Italian Neurosurgical Society. Acta Neurochir Suppl. 2005;93:113-119.
69 Rodriguez RF, Contreras N. Bilateral motor cortex stimulation for the relief of central dysesthetic pain and intentional tremor secondary to spinal cord surgery: a case report. Neuromodulation. 2002;5:189-195.
70 Arle JE, Apetauerova D, Zani J, et al. Motor cortex stimulation for Parkinson’s disease: 12-month follow-up in 4 patients. J Neurosurg. 2008;109:133-139.
71 Cilia R, Landi A, Vergani F, et al. Extradural motor cortex stimulation in Parkinson’s disease. Mov Disord. 2007;22:111-114.
72 Kleiner-Fisman G, Fisman DN, Kahn FI, et al. Motor cortical stimulation for parkinsonism. Arch Neurol. 2003;60:1554-1558.
73 Munno D, Caporale S, Zullo G, et al. Neuropsychologic assessment of patients with advanced Parkinson disease submitted to extradural motor cortex stimulation. Cogn Behav Neurol. 2007;20:1-6.
74 Cioni B. Motor cortical stimulation for Parkinson’s disease: The Italian Neurosurgical Study Group. Presented at the 14th Meeting of the World Society for Stereotactic and Functional Neurosurgery; June 13-17, 2005; Rome, Italy.
75 Cioni B. Motor cortical stimulation for Parkinson’s disease: The Italian Neurosurgical Study Group. Presented at Intraoperative Monitoring in Neurosurgery: Vth International Symposium; November 16-18, 2006; New York.
76 Son BC, Lee SW, Choi ES, et al. Motor cortex stimulation for central pain following a traumatic brain injury. Pain. 2006;123:210-216.
77 Nguyen JP, Keravel Y, Feve A, et al. Treatment of deafferentation pain by chronic stimulation of the motor cortex: Report of a series of 20 cases. Acta Neurochir Suppl. 1997;68:54-60.
78 Amassian VE, Stewart M, Quirk GJ, et al. Physiological basis of motor effects of a transient stimulus to cerebral cortex. Neurosurgery. 1987;20:74-93.
79 Hanajima R, Ashby P, Lang AE, et al. Effects of acute stimulation through contacts placed on the motor cortex for chronic stimulation. Clin Neurophysiol. 2002;113:635-641.
80 Strassman A, Mason P, Moskowitz M, et al. Response of brainstem trigeminal neurons to electrical stimulation of the dura. Brain Res. 1986;379:242-250.
81 Alterman RL, Sterio D, Beric A, et al. Microelectrode recording during posteroventral pallidotomy: impact on target selection and complications. Neurosurgery. 1999;44:315-323.
82 Schwalb JM, Moro E, Hamani C, et al. Open label trial of subdural motor cortex stimulation (MCS) for Parkinson’s disease [abstract]. Presented at the Meeting of the American Society for Stereotactic and Functional Neurosurgery; June 1-4, 2006; Boston.
83 Drouot X, Oshino S, Jarraya B, et al. Functional recovery in a primate model of Parkinson’s disease following motor cortex stimulation. Neuron. 2004;44:769-778.