CHAPTER 7 Traumatic and Nontraumatic Spine Emergencies
TRAUMATIC SPINE INJURY
Background and Imaging Algorithms
valid indications are altered mental status, evidence of intoxication with ethanol or drugs, painful distracting injuries, neurologic deficits, and spine pain or palpation tenderness.
Patterns of Spine Injury and Imaging Findings
Careful analysis of the structures (vertebrae, intervertebral discs, spinal cord, and other soft tissues) and their normal and abnormal attributes (size, shape, alignment, density, and signal intensity) requires an understanding of mechanisms of injury, including magnitude and acuity, and underlying diseases. The mechanisms can generally be grouped into hyperflexion, hyperextension, rotation, axial loading, lateral flexion, and others. Box 7-1 attempts to categorize the injuries of the cervical spine based on these mechanisms. Combined mechanisms, such as flexion and rotation, are common and may lead to multiple injuries at different sites and vertebral levels within the same patient. Rather than relaxing after detecting an injury, the examiner should intensify the search for other lesions.
Injuries of the Cervicocranium
Occipital Condyle
Occipital condyle fractures are relatively rare and often classified into three types. Type I is a longitudinal fracture from axial loading. Type II is a continuation of a fracture from the flat part of the occipital bone. Type III is the most serious, an avulsion of the condyle at the attachment of the alar ligament, and may be unstable (Fig. 7-1).
Atlanto-occipital Dislocation
Atlanto-occipital dislocation (AOD) refers to disruption of the articulations of the skull and spine. Atlanto-occipital dissociation (synonymous with craniocervical dissociation) is a broader term encompassing partial (subluxation) and complete (dislocation) disruption of the articulations. Following high-speed motor vehicle collisions, this injury is more often diagnosed at autopsy than at the trauma center. Although no method is perfect, measurement of the basion-dental interval (BDI) or the basion-posterior axial line interval (BAI) of more than 12 mm was reported to be 100% sensitive in one published study (Fig. 7-2).
C1 Fractures
The classic Jefferson burst fracture of the atlas (C1) is due to disruption of the anterior and posterior arches (with several possible variations). Lateral displacement of the lateral masses of C1 with respect to C2 is seen on the open mouth view radiograph or the CT coronal reformatted image (Fig. 7-3). Isolated anterior arch (which goes against the pretzel ring theory) or lateral mass fractures may also occur at this level.
Atlantoaxial Dissociation
Atlantoaxial dissociation includes partial (subluxation) and complete (dislocation) disruptions of the articulations of C1 and C2. Disruption of the transverse atlantal ligament (TAL), the horizontal component of the cruciform ligament complex, allows for widening of the anterior atlantodental interval (AADI) (Fig. 7-4). Greater than 3 mm in adults or greater than 5 mm in children is considered abnormal. Conditions that may predispose to atlantoaxial dissociation include rheumatoid arthritis, Down syndrome, neurofibromatosis, and other syndromes and congenital anomalies. Rotatory dissociation (fixation) is rare and has been subdivided into four types based on extent and direction of displacement of the atlas. Type I may appear similar to physiologic rotation. Therefore, to confirm the diagnosis, CT may be repeated after voluntary contralateral rotation of the head to assess for a locked position. Torticollis refers to simultaneous lateral tilt and rotation of the head and may be caused by disorders affecting either the atlantoaxial joint or the sternocleidomastoid muscle. Since it may produce the same imaging findings as type I rotatory fixation, diagnosis may rely on clinical judgment and a trial of conservative treatment. Types II, III, and IV are determined based on direction and extent of displacement of the cranium.
C2 Fractures
Approximately 20% of cervical fractures involve the axis (C2). Of these, more than half are traumatic spondylolysis/spondylolisthesis—fracture between the superior and inferior facets (pars interarticularis) of C2—often described as the “hangman” fracture (Fig. 7-5). Due to the unique shape of C2, this fracture involves the pedicles, whereas a pars fracture of the subaxial spine is termed the “pillar” fracture. Aside from the mechanism implied by the name, other forms of hyperextension, such as motor vehicle dashboard impact, are usually to blame. At least three types have been described, based on degree of fragment distraction, angulation at the fracture site, and disruption of the C2-C3 disc.
The dens (odontoid process) may be involved in approximately 25% of C2 fractures. The classification system of Anderson and D’Alonso is commonly applied. Type I is uncommon—an avulsion of the tip by the alar ligament—and may be associated with AOD. Type II is the most common (about 60%) and involves the base of the dens (Fig. 7-6). Operative repair via transoral screw fixation or posterior arthrodesis of C1 and C2 is often necessary. Type III involves the dens and body of C2. Due to the larger surface area, this type of fracture is more likely to heal without the need for instrumentation (Fig. 7-7). As the plane may be nearly horizontal, dens fractures may be quite subtle on axial CT. Sagittal reformats therefore demand careful review. Beware of misregistration artifact of axial CT, due to patient movement between adjacent images, although this has become less of a problem since the advent of helical and MDCT techniques. Since the scanning process is so fast with these techniques, a different type of artifact can result—motion blur. Distorted images should signal the need to repeat the scan. For uncooperative patients, a lateral plain film may provide complementary demonstration of proper alignment.
Injuries of the Subaxial Cervical Spine
Hyperflexion
Injury of the subaxial spine generally follows a set of patterns based on the mechanism and degree to which the subaxial spine has been stressed beyond physiologic limits. Of the injuries caused by hyperflexion, the simple wedge compression fracture (involving the anterior column) and the isolated spinous process (clay shoveler) fracture are relatively straightforward in terms of diagnosis. Although generally considered stable injuries, they can occur in association with other, more insidious injuries, and therefore flexion/extension radiographs or MR may be indicated. Anterior subluxation (or hyperflexion sprain) is indicative of injury to the posterior ligamentous structures. It can be quite subtle, presenting with focal kyphotic angulation, very mild malalignment of facets, and widening of the posterior margin of the disc space and interspinous distance. Failure to diagnose and treat this injury may result in delayed instability in up to 50% of cases. As the degree of anterior vertebral body translation, facet joint distraction, and “fanning” of spinous processes increases, the signs of instability become more obvious. Facets may become perched or jumped (complete dislocation). Anterior translation of a vertebral body by more than 50% relative to the subjacent body is a sign of bilateral interfacetal dislocation (BID) (Figs. 7-8 and 7-9). With perched facets, the “naked facet” sign will be seen on axial CT. With BID, the convex surfaces of the articular masses will be apposed rather than the usual concave articular surfaces. Anterior, middle, and the posterior ligamentous structures will be disrupted, and the extent of soft tissue injuries (including cord compression and contusion) will be best assessed by MR. If there is any rotational component to the mechanism of injury, unilateral interfacetal dislocation (UID) or combination of perched and jumped facets may occur. Fractures of the subjacent vertebral body or articular masses will result in a fracture-dislocation. The flexion teardrop fracture is thought to result from a combination of hyperflexion and axial loading. It is feared because of the high incidence of permanent neurologic damage resulting from the more complex variety, which usually includes fractures of the posterior elements. Although it can be difficult to distinguish this from the hyperextension teardrop fracture based on the configuration of the vertebral body fracture alone, the kyphosis and posterior element distraction are important clues.
Hyperextension
The types of injuries to the subaxial spine resulting from hyperextension mechanisms are analogous to those sustained by hyperflexion, but with a few differences. Hyperextension sprain, dislocation, and fracture-dislocation encompass a spectrum that may result from a blow to the face, as from impact against the dashboard during a motor vehicle collision, an assault, or a fall. Soft tissue structures are involved in a progressive fashion from anterior to posterior, including prevertebral muscles, anterior longitudinal ligament, anterior portion of annulus, intervertebral disc, posterior longitudinal ligament, and so on. A sprain usually leaves the middle and posterior columns intact. A dislocation also disrupts the middle and possibly posterior ligaments. A fracture-dislocation may result in fractures of the posterior aspect of the vertebral body and any of the posterior elements due to impaction forces. When underlying ankylosis is present, such as in diffuse idiopathic skeletal hyperostosis or ankylosing spondylitis, a relatively mild hyperextension force may result in fracture-dislocation (see Fig. 7-6). This is due to the loss of normal ligamentous laxity, a lever arm up to several spinal segments long, and coexistent osteoporosis. An extension teardrop fracture fragment is the result of avulsion by the anterior longitudinal ligament. The vertical dimension of the fragment is generally greater than the horizontal dimension (Fig. 7-10).
Extension combined with rotation and lateral or tilting forces may result in articular mass (also known as pillar) fractures (Fig. 7-11). A fracture of the pedicle and ipsilateral lamina may result in a freely mobile articular mass (pedicolaminar separation). Occasionally, isolated fracture(s) of the lamina(e) will be occult on anteroposterior and lateral films. This is one advantage of oblique radiographs. Anotheradvantage is confirmation of unilateral facet dislocation, but, alas, MDCT has made these films nearly obsolete. It is the rare technologist who can still obtain perfect trauma oblique views taken without removing the cervical collar or turning the patient’s head. It seems that most centers have abandoned oblique views from the routine cervical spine series. The extra clues provided by oblique views may still have value in centers where trainees interpret plain film exams on call.
Lateral Bending
Injuries due to lateral bending do not seem to be very common, but an occasional uncinate process or transverse process fracture may be detected by CT. Fracture involving the foramen transversarium brings a risk of dissection or occlusion of the vertebral artery and, with it, the possibility of stroke. A discussion of the workup of vascular injuries of the neck and head is included in Chapter 1 in the section on cerebrovascular disorders.
Pitfalls in Spine Imaging
One must become familiar with the normal pattern of development and congenital anomalies in children, such as os odontoideum, incomplete ossification of the neural arches, and pseudosubluxation in order to avoid false-positive diagnoses (Fig. 7-12).
It is often helpful to realize that abnormalities need to be evaluated in context. For example, not every malalignment is an acute one. Mild subluxations of the cervical spine are quite common due to chronic osteoarthritis. Therefore, analysis of the pattern of facet hypertrophy may provide confidence that the deformity is chronic. Dynamic testing with flexion-extension radiographs may support such a conclusion. On the other hand, sometimes it is necessary to call attention to a clearly chronic finding detected serendipitously. An os odontoideum that is well corticated but chronically unstable provides just one such example (Fig. 7-13).
Injuries of the Thoracolumbar Spine
Although similar mechanisms of injury apply, differences in biomechanics between the cervical and thoracolumbar spine result in different patterns of findings. Compression fractures due to flexion are very common (Fig. 7-14). Osteoporotic compression fractures are common, with more than 700,000 occurring annually in the United States, with costs from treatment approaching $1.5 billion. While the majority may be considered stable, medical therapies required to treat the associated pain may lead to other serious complications. The increase in age-adjusted 5-year mortality approaches 25% for female patients. Use of alcohol and certain medications, including steroids, anticonvulsants, cytotoxic drugs, thyroid hormones, and heparin, may all be associated with osteoporotic compression fractures. Metastatic disease may predispose to the development of pathologic compression fractures. One fracture also increases the risk for development of other fractures due to altered biomechanics of the kyphotic spine. Vertebroplasty and kyphoplasty are minimally invasive spine procedures that are possible treatments for those patients who do not respond to an appropriate trial of conservative therapies. With more than 50% loss of anterior vertebral body height, disruption of the posterior longitudinal ligament may occur, leading to the possibility of instability.
Axial loading mechanism may result in a burst fracture. These fractures occur most commonly near the thoracolumbar junction but may also affect the mid- and lower lumbar levels. The extent of retropulsion of the posterior portion of the vertebral body, the relative size of the spinal canal, and the position of the conus medullaris are factors affecting the risk of neurologic injury. Screening exam of the thoracolumbar spine should be performed when calcaneal fractures result from a jump or fall from a height due to the high association of these injuries (Fig. 7-15).
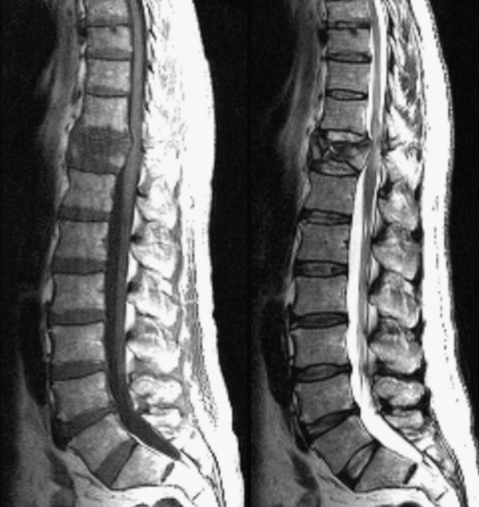
Thoracic burst fracture. Sagittal T1-weighted image (left) and T2-weighted image (right) show retropulsion of the posterior portion of the T11 vertebral body resulting in cord compression. Cord T2 hyperintensity is consistent with edema. A contusion should be suspected based on the degree of compression; however, there is no T2 or gradient-echo hypointensity to confirm the presence of hemorrhage.
The combination of flexion and distraction, common in lap belt deceleration injuries, results in a rather unique pattern of findings. A horizontally oriented fracture plane through the intervertebral disc and posterior elements has come to be called the “Chance” fracture. Since there are many variations in terms of bony and ligamentous components, the term “Chance-type fracture” may be applied to these horizontal fractures most commonly affecting the upper lumbar spine (Fig. 7-16). The abdominal viscera should be carefully evaluated in patients with Chance-type fractures, since there is strong association with intestinal and solid organ injuries.
Evaluation of Spinal Soft Tissue Injuries
Spinal Cord Injury
Diagnosis of injury to any of the other soft tissue structures listed below can be based on T2 hyperintensity. In addition, anterior and posterior longitudinal ligaments may be displaced (elevated) or disrupted. Discs may be widened or extruded. Facet capsules may be widened and fluid-filled, or facets may be dislocated. Ligamentum flavum and interspinous ligaments may be disrupted. Cervical root avulsion as a cause of brachial plexopathy may present with abnormal enlargement of nerve root sleeve(s)—pseudomeningocele (Fig. 7-17).
Spinal Hematomas
On the other hand, hemorrhage confined by the thecal sac must be within either the subdural or subarachnoid space. Differentiation of the three types of extramedullary spinal hemorrhage can sometimes be quite difficult. Extension into the neural foramen, beyond the expected confines of the thecal sac, is a sure indication that the hemorrhage is in the epidural space. Subarachnoid hemorrhage often collects in a uniform circumferential configuration around the spinal cord, mixes uniformly with cerebrospinal fluid and intermingles with the roots of cauda equina, and seeks a dependent position in the spinal canal. Spinal subdural hematoma is easiest to differentiate when the arachnoid mater and roots are displaced by a hematoma confined to the thecal sac (Figs. 7-18 to 7-20).
NONTRAUMATIC SPINE EMERGENCIES
Degenerative Disease/Arthropathy
As mentioned in the section on spine trauma, underlying conditions resulting in narrowing of the spinal canal are often found in patients presenting with spinal cord injuries, sometimes resulting from rather minor trauma. There are many causes of canal stenosis, including routine spondylosis, ossification of the posterior longitudinal ligament (OPLL) ossification of ligamentum flavum, synovial cyst, and epidural lipomatosis. Underlying disorders such as these are often discovered in the setting of an acute disc extrusion that results in a new neurologic deficit. Degenerative disc disease is ubiquitous, and the imaging findings are generally straightforward. One should not be fooled by the T2 hyperintensity of a disc extrusion that simulates fluid or by a sequestered fragment displaced a considerable distance from the remainder of the disc. Gadolinium-enhanced images may help to identify root inflammation or arachnoiditis and differentiate granulation tissue (scar) from a recurrent disc extrusion in the setting of a postoperative patient presenting with recurrent symptoms. Familiarity with expected routine postoperative findings is important, but one must also be able to analyze features in a systematic fashion, for example, to determine if a cerebrospinal fluid leak/pseudomeningocele might be causing root compression (Fig. 7-21).
Other arthritides such as amyloidosis or crystal pyrophosphate deposition may present with mass lesions or destructive changes of the spine, mimicking malignancy or infection (Fig. 7-22).
Neoplasms and Tumorlike Conditions
Extradural lesions are encountered most commonly, and these include the disc herniations and degenerative changes from arthritis and traumatic conditions already discussed. Metastatic disease makes up the majority of the neoplastic extradural masses, usually from carcinomas of the lung, breast, and prostate. Although metastasis to the bone with extradural extension is typical, metastasis to or primary involvement of the extradural soft tissues may also result in neural compromise. Lymphoma may present this way or by extension from the retroperitoneum through the neural foramen (Fig. 7-23). Vertebral involvement by multiple myeloma may present with an extradural mass or pathologic compression fracture (Fig. 7-24). Common osseous lesions such as aneurysmal bone cyst, osteoblastoma, osteochondroma, and Paget disease may occasionally present with symptoms related to cord compression. Chordoma, a destructive bone tumor arising from notochord rests, accounts for approximately 5% of primary bone tumors. Most commonly occurring in the sacrum, it may also arise in the clivus, cervical, or lumbar region. A large soft tissue mass, calcifications, and residual bone fragments are typical features.
Intramedullary tumors account for approximately 10% of primary intraspinal tumors; the majority of these are gliomas (approximately 70% ependymomas and the rest astrocytomas). These may be indistinguishable by imaging; both may show enlargement of the cord and irregular T2 hyperintensity and enhancement, and have associated cystic changes or syrinx formation (Fig. 7-25). Ependymomas have a predilection for the conus medullaris and a tendency to bleed. Slow-growing primary lesions may cause expansion of the spinal canal, scalloping of the posterior margins of the vertebral bodies, and pressure atrophy of pedicles. Other intramedullary tumors include hemangioblastoma, medulloblastoma, and metastatic disease from pineal or other intracranial site.
Inflammation/Demyelination
Multiple sclerosis (MS) may be the first disorder that comes to mind in this category. The cause of this disorder, characterized by breakdown of the myelin sheath and inflammation, is unknown. Spinal cord plaques tend to occur most frequently in the cervical region and show imaging features similar to those found in the brain—T1 iso- to hypointense, T2 hyperintense—and may enhance following contrast administration (Fig. 7-26). The cord may be swollen due to active inflammation. Lesions in different states of activity and the presence of lesions in the brain add to confidence in the diagnosis of MS. Cord atrophy and lack of enhancement may be expected in the chronic phase.
Infection
Epidural (bacterial) abscess may arise via hematogenous route or from direct extension of discitis/osteomyelitis or other paraspinal source (Fig. 7-27). Staphylococcus aureus is the most common organism, and risk factors include spinal instrumentation, intravenous drug use, immunodeficiency, diabetes, renal failure, alcoholism, and malignancy. Because of the loose connection of the dura to the spine, infection may spread along multiple contiguous levels. Lumbar and cervical regions are most commonly affected. On MR, this appears as a rim-enhancing, tapered fluid collection that displaces the dura. The collection may be located anteriorly, posteriorly, or circumferentially around the thecal sac and may cause compression of the spinal cord or cauda equina. The term “phlegmon” may be applied to an enhancing inflammatory mass without a central fluid collection. Definitive treatment is surgical, although mild cases without a focal neurologic deficit may be treated with antibiotics and careful monitoring. Determination of the cranial and caudal extent of the infection is imperative prior to any planned intervention.
Blackmore C.C., Emerson S.S., Mann F.A., et al. Cervical spine imaging in patients with trauma: Determination of fracture risk to optimize use. Radiology. 1999;211:759-765.
Blake S.P., Connors A.M. Sacral insufficiency fracture. Br J Radiol. 2004;77:891-896.
Darouiche R.O. Spinal epidural abscess. N Engl J Med. 2006;355:2012-2020.
Denis F. Spinal instability as defined by the three-column spine concept in acute spinal trauma. Clin Orthop. 1984;189:65-76.
Goradia D., Linnau K.F., Cohen W.A. Correlation of MR imaging findings with intraoperative findings after cervical spine trauma. Am J Neuroradiol. 2007;28:209-215.
Harris J.H., Carson G.C., Wagner L.K., et al. Radiologic diagnosis of traumatic occipitovertebral dissociation: 2. Comparison of three methods of detecting occipitovertebral relationships on lateral radiographs of supine subjects. AJR Am J Roentgenol. 1994;162:887-892.
Harris J.H.Jr. The cervicocranium: Its radiographic assessment. Radiology. 2001;218:337-351.
Harris J.H.Jr., Mirvis S.E. The Radiology of Acute Cervical Spine Trauma, 3rd ed. Baltimore: Williams and Wilkins; 1996.
Hogan G.J., Mirvis S.E., Shanmuganathan K., et al. Exclusion of unstable cervical spine injury in obtunded patients with blunt trauma: Is MR imaging needed when Multi-detector row CT findings are normal? Radiology. 2005;237:106-113.
Katzberg R.W., Benedetti P.F., Drake C.M., et al. Acute cervical spine injuries: Prospective MR imaging assessment at a level 1 trauma center. Radiology. 1999;213:203-212.
Koeller K.K., Rosenblum R.S., Morrison A.L. Neoplasms of the spinal cord and filum terminale: Radiologic-pathologic correlation. Radiographics. 2000;20:1721-1749.
Kwon B.K., Hilibrand A.S. Management of cervical fractures in patients with diffuse idiopathic skeletal hyperostosis. Curr Opin Ortho. 2003;14:187-192.
Labler L., Eid K., Platz A., et al. Atlanto-occipital dislocation: Four case reports of survival in adults and review of the literature. Eur Spine J. 2004;13:172-180.
Leone A., Cerase A., Colosimo C., et al. Occipital condylar fractures: A review. Radiology. 2000;216:635-644.
Lustrin E.S., Karakas S.P., Ortiz A.O., et al. Pediatric cervical spine: Normal anatomy, variants, and trauma. Radiographics. 2003;23:539-560.
Munday T.L., Johnson M.H., Hayes C.W., et al. Musculoskeletal causes of spinal axis compromise: Beyond the usual suspects. Radiographics. 1994;14:1225-1245.
Platzer P., Jaindl M., Thalhammer G., et al. Clearing the cervical spine in critically injured patients: A comprehensive C-spine protocol to avoid unnecessary delays in diagnosis. Eur Spine J. 2006;15:1801-1810.
Pollack C.V.Jr., Hendey G.W., Martin D.R., et al. Use of flexion-extension radiographs of the cervical spine in blunt trauma. Ann Emerg Med. 2001;38:8-11.
Schuster R., Waxman K., Sanchez B., et al. Magnetic resonance imaging is not needed to clear cervical spines in blunt trauma patients with normal computed tomographic results and no motor deficits. Arch Surg. 2005;140:762-766.
Schwartz E.D., Flanders A.E., editors. Spinal Trauma. Philadelphia: Lippincott Williams and Wilkins, 2007.
Sklar E.M., Post J.M., Falcone S. MRI of acute spinal epidural hematomas. J Comput Assist Tomogr. 1999;23:238-243.
Wang Y.F., Teng M.M., Chang C.Y., et al. Imaging manifestations of spinal fractures in ankylosing spondylitis. Am J Neuroradiol. 2005;26:2067-2076.