7 Trauma and multiple injury
Trauma epidemiology
However, experience of trauma cannot be extrapolated from other countries to predict events or outcomes. For example, in the UK, RTAs, falls and interpersonal violence account for the majority of major trauma (Fig. 7.1). Fewer than 1 in 10 patients with major trauma have penetrating injury, and this is usually caused by knives. In the USA, approximately 20% of the population owns a gun, and RTA deaths are matched by firearm injuries. Staggeringly, having a gun in the home increases the risk of homicide three-fold, and that of suicide five-fold; and for 15–24-year-olds, these figures increase by a factor of 10. A recent UK study found that the total number of homicides over a 2-year period for a population of 0.8 million was similar to that seen in a single day among the same-sized population of many American cities. In so-called developed countries, annually, 1 person in 50 will be involved in an RTA. Of these, 1% will die, 10% will need hospital treatment and 25% will be temporarily disabled.
Injury biomechanics and accident prevention
where E = energy transfer, T = time, V = volume of tissue, and C = tissue factors (a constant).
These physical principles underpin strategies of accident prevention and protection. Obviously, reducing the chance of direct contact helps; separating pedestrians and traffic is the single most important factor in reducing pedestrian injury rates. This is illustrated by the fact that, in the US, less than 2% of traffic fatalities are pedestrians, whereas in the UK they account for 36% of the total. In a similar fashion, the central reservation barriers on motorways dramatically reduce the chances of high-speed head-on collisions (Fig. 7.2).
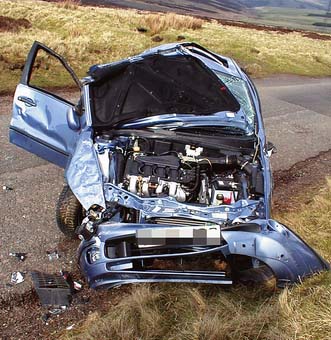
Fig. 7.2 Car involved in a high-speed, head-on collision, showing the magnitude of structural damage.
Contact factors can be minimized by vehicle design: crumple zones, energy-absorbing materials, preventing the ejection of passengers from the vehicle and reducing intrusion into the passenger compartment. For the occupants, seatbelts, airbags, collapsible steering columns and soft fascia compartments enable contact deceleration to take place over a longer time period, reducing the potential for injury (Fig. 7.3). Properly used, seatbelts reduce the risk of death/serious injury by 45%. Airbags further reduce the risk of death by 10% for belted drivers, and by 20% for unbelted front-seat passengers, but may not provide protection from side-impact events, or if the vehicle rolls over.
Summary Box 7.1 Accident features associated with major trauma
Wounds
Classification and production
• Abrasions or grazes. These are caused by the tangential application of blunt force. Dirt is often ingrained in the surface layers of skin, with the risk of short-term infection and, if untreated, later permanent ‘tattooing’. The abrasion’s site and nature may give useful clues as to the direction and magnitude of injury forces.
• Contusions, ecchymoses or bruises. These result from blunt force disrupting superficial capillaries. The overlying skin is intact. When small blood vessels are involved, a large collection of blood (haematoma) may develop. It is impossible to tell the age of a bruise accurately by its colour, but if it is yellow (caused by breakdown of haemoglobin pigment to biliverdin and bilirubin), the bruise is likely to be at least 18 hours old.
• Lacerations. Blunt forces tear, shear or crush skin and soft tissues, producing lacerations. The wound edges are irregular and often abraded, contused and crushed, as are the surrounding tissues.
• Incised wounds or ‘cuts’. These are produced by sharp edges, such as knives or glass shards, and have characteristically clean edges with clear margins. The greatest dimension of an incised wound is its length (cf. puncture wound).
• Puncture wounds. Sharp points or edges produce puncture wounds, in which the greatest dimension is the depth. When the wound pierces a body cavity, it is ‘penetrating’; if it passes through a viscus, it is ‘perforating’.
Gunshot wounds
Gunshot wound data (Fig. 7.4) highlight the gulf between UK and US practice. In the US, deaths from gunshot wounds are the fourth leading cause of years of potential life lost before the age of 65. Guns are used in over 60% of suicides and 70% of all homicides. Non-fatal gunshot wounds outnumber fatal ones two- to three-fold.
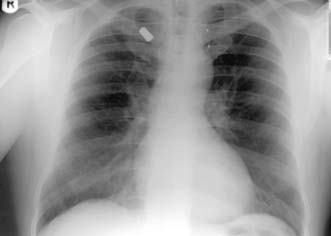
Fig. 7.4 Chest X-ray showing a bullet to the right of the upper mediastinum.
(Courtesy of Miss Kate Wilson.)
Shotgun events are relatively more common in the UK than handgun or rifle injuries (http://www.crimeandjustice. org.uk/). The muzzle velocity of these weapons is relatively high, but dissipation of the shot and air resistance on the pellets quickly decrease their velocity and limit the wounding potential (Fig. 7.5). These weapons are lethal at close range but, unless ‘choked’, are relatively less wounding at greater distances, where they tend to cause superficial injury to skin and subcutaneous tissues.
Falls
where g = gravitational constant (9.8 m/s) and h = height fallen in meters
The body’s position during landing affects the contact area and the propagation of energy since, if the same force is dissipated over a larger area, there is less force per unit area and hence less damage (Figs 7.6 and 7.7). Feet-first falls involve a relatively small area of contact, but deceleration forces can be reduced by flexing the knees and hips. Regardless of the position on landing, however, for falls > 5 m there is a high incidence of deceleration injuries to intrathoracic and intra-abdominal structures, particularly where these are relatively immobile or tethered: for example, the aortic root and the mesenteric arteries. Overall, falls on to an unyielding surface from 15–20 m are associated with a greater than 50% mortality. Nevertheless, bizarre descriptions of survival from high falls do exist. There are well documented accounts of individuals surviving after falling from aircraft onto trees and deep snow. Clothing may also play a role in slowing the rate of fall: in 1885, Miss Sarah Ann Henley survived after a fall from the Clifton suspension bridge (∼︀75 m) as her hooped crinolines acted as a parachute and the tide was out so that the landing surface was thick mud.
Injury severity assessment
The second type of classification is physiological. The best-known physiological scoring system is the Glasgow Coma Scale (Table 7.1), which is used to assess the neurological state of injured patients objectively, and which also has prognostic value. The Glasgow Coma score (GCS), in conjunction with two other physiological recordings, systolic blood pressure and respiratory rate, can be used to produce the ‘Revised Trauma Score’. Although widely used, physiological scoring systems have intrinsic problems. Some patients with severe injury may not be identified initially, usually because the assessment has been performed before detectable physiological compromise has had time to occur. The system may also overestimate injury severity if physiological changes occur (due, for example, to alcohol) that are not reflected in the measured parameters, or which modify these factors. In addition, no allowance is made for factors such as comorbid features e.g. underlying cardiac or pulmonary disease or medications.
Eyes open | |
Trauma centres
Independent evaluation of the pilot trauma centre in England, however, failed to show a reduction in death rates. Two facts may explain this disappointing result. The first is the difference in trauma epidemiology, in relation to both the nature of the trauma and the volume of patients presenting. Secondly, despite having run for several years, the centre was not fully integrated into a comprehensive regionalized system (EBM 7.1). For the foreseeable future in the UK, the provision of care will be by a trauma team approach (Fig. 7.8), although reorganization and regionalization of trauma services in some UK areas is developing aspects of trauma centre care (http://www.rcseng.ac.uk/publications/docs/provision-of-trauma-care-1).
Resuscitation in the emergency department
The first 10 minutes
• number, age and sex of patients
• nature of the incident and any special features, e.g. associated chemical/radioactive contamination, helicopter transportation etc
• brief details of injuries, treatment given at the scene/in transit and current condition.
A trauma team, consisting of 4–5 experienced doctors and nurses, is used for the patient’s initial assessment and treatment (Fig. 7.8). Each team member has a pre-assigned role and performs this, unless directed otherwise by the team leader, who must have sufficient seniority and competence to direct and control the entire resuscitation process. The team members must be entirely familiar with the tasks required of them and perform them with minimal delay or questioning.
Airway
Control of the cervical spine
Orotracheal intubation is the advanced airway technique of choice (Fig. 7.9). It protects the airway from aspiration of vomit or blood, and allows ventilation with controlled levels of oxygen and airway suctioning to remove debris. It does, however, require expertise in using anaesthetic and neuromuscular paralyzing agents. Prior to intubation, the patient is pre-oxygenated and must be carefully monitored throughout the process. A ‘surgical’ airway is extremely rarely needed; if one is required, a percutaneous cricothyrotomy is the simplest, safest and quickest surgical approach (see chapter 8).
Breathing
Clinical inspection, palpation and auscultation of the neck and chest (including the back) should detect immediately life-threatening injuries such as flail segment, penetrating wounds, tension or open pneumothoraces, major haemothorax and cardiac tamponade. These conditions need immediate treatment, e.g. needle thoracocentesis for tension pneumothorax, or the insertion of an intercostal drain for haemothorax. Open or sucking chest wounds (Fig. 7.10) are rare but, if present, allow equalization of atmospheric and intrathoracic pressures. With large defects, atmospheric air passes through the wound into the intrathoracic space with each inspiration, and the lung collapses. To prevent this, the open wound is covered with a sterile occlusive dressing, taped on three sides. This acts as a flutter valve, and formal tube thoracostomy is then performed at a separate site from the open wound.
Repeated arterial blood gas analyses are needed to ensure that hypoxia is not present and that alveolar ventilation is sufficient to prevent hypercapnoea. For patients who are intubated and ventilated, additional problems may develop. Positive-pressure ventilation may reduce cardiac output (manifest initially by tachycardia ± hypotension) because of decreased venous return to the heart, resulting from increased intrathoracic pressure during the ‘inspiratory’ phase of ventilation. The risk of pneumothorax (Table 7.2) in patients with coexisting chest injuries is markedly increased by positive-pressure ventilation. If a pneumothorax is already present, tension may be induced. For these reasons, tube thoracostomy is mandatory if a pneumothorax is present and positive-pressure ventilation, for whatever reason, is to be undertaken.
Circulation
To reduce blood loss is essential. External haemorrhage can invariably be controlled by simple direct pressure. Haemostasis from the sometimes profuse bleeding of scalp wounds is best achieved with carefully applied sutures or staples. Splinting of long-bone fractures reduces blood loss from fracture sites by up to 50%, makes the patient more comfortable, and reduces analgesic requirements (Fig. 7.11). In contrast, blood loss into the peritoneal cavity, thorax or pelvis is usually concealed, can be life-threatening, and cannot be simply controlled. Patients with major pelvic fractures pose a difficult management problem, as conventional splintage is impossible and massive and uncontrollable blood loss may result (Fig. 7.12). The optimal approach is the application of external fixator devices in the resuscitation phase, followed, if required, by angiographic embolization.
The choice of fluids for the replacement of traumatic blood loss is controversial and poorly understood. Intravenous volume replacement is begun with infusion of an isotonic crystalloid such as 0.9% saline or Ringer’s lactate. In the UK, after 1000–2000 ml of crystalloid, a colloid is commonly given prior to, or together with, blood. Theoretically, colloids (such as albumin solutions, gelatins, starches or dextrans) might be expected to be more effective, but there is no good evidence to suggest this in clinical practice (EBM 7.2).
Blood transfusion requirements depend upon the magnitude of blood loss and the physiological response. It is usual to replace losses with the aim of maintaining the patient’s haematocrit at ∼︀30%. Where there is immediately life-threatening haemorrhage, group O Rhesus-negative blood is given, but more usually fully cross-matched or type-specific blood can be supplied. Most transfusion services supply packed red cells. There is no evidence that ‘fresh’ whole blood is preferable. In situations of massive blood loss, where replacement of more than the equivalent of one circulating blood volume is needed, coagulation problems should be anticipated. Military experience has led to development of massive transfusion guidelines including early use of platelets and coagulation factors, often directed by ‘near-patient’ testing of coagulation. Recently, tranexamic acid (an antifibrinolytic agent) has been shown to improve morbidity and mortality in trauma patients at risk of major bleeding (EBM 7.3). Close liaison with the Blood Transfusion Service and haematology laboratories is essential for optimal management.
The next phase
The patient is examined from top to toe to ensure that no wound, bruise or swelling is missed. The back and spine are examined with the patient ‘log-rolled’, looking specifically for localized tenderness, swelling, bruising or a ‘step’. The perineum is examined and a rectal examination performed (Table 7.3).
The neurological status of the patient is recorded regularly, including the GCS, pupil sizes and reactions, and any focal deficit). The ears, nose and mastoid areas are carefully examined for evidence of skull-base injury, such as blood/cerebrospinal fluid otorrhoea or rhinorrhoea, or bruising. Muscle power should be tested and recorded using the MRC (Medical Research Council) scale (Table 7.4), and the tendon reflexes examined. It is vital to test sensation in a methodical fashion (Fig. 7.13). The perineal area must be included (this is most easily achieved at the time of rectal examination), as the lowest (sacral) dermatomes are in this area.
Table 7.4 Assessing muscle power: the MRC scale
Imaging and other diagnostic aids
There are three primary X-ray views in the blunt trauma patient, but these do have limitations:
• The chest X-ray may demonstrate thoracic injuries previously unrecognized on clinical examination, but even on a good-quality erect view over half of the rib fractures actually present will be missed (Fig. 7.14). The patient’s condition often precludes an erect film, and on a supine view pneumothoraces and/or haemothoraces are difficult to detect; even in the absence of pathology, the mediastinal contours are displaced and widened.
• The lateral view of the cervical spine should be a cross-table film and must include all of the vertebrae from C1 to T1. This provides valuable information on acute bony injury (Fig. 7.15), but a ‘normal’ neck X-ray does not exclude significant injury to soft tissues, including the cervical cord.
• On a plain antero-posterior view of the pelvis, injuries to the posterior elements are difficult to see (especially around the sacroiliac regions, which may lead to significant occult haemorrhage).
Summary Box 7.8 Initial X-rays in the patient with blunt trauma
X-rays (Fig. 7.16), CT defines the nature and magnitude of the intracranial insult (Fig. 7.17). It is therefore invaluable in providing the information the physician needs to determine the requirement for neurosurgical intervention (EBM 7.4).
After the resuscitation room
Summary Box 7.9 Information required by the receiving unit or hospital
• Previous health status and medications (if known)
• Pulse, blood pressure and respiratory rate (at scene, on arrival and current)
• Glasgow Coma Scale (at scene, on arrival and at present)
• Summary of injuries, including signs of head injury and any lateralizing signs
• Summary of i.v. fluids (including blood), and the haemodynamic and urine output responses
• X-ray, CT or other imaging results
• Blood grouping/cross-matching, haematology and biochemistry results
• Tetanus status/cover provided, antibiotics and other drugs given (include doses and timing).