Chapter 21 Transplantation and Rejection
• Transplantation is the only form of treatment for most end-stage organ failure.
• The barrier to transplantation is the genetic disparity between donor and recipient.
• The immune response in transplantation depends on a variety of factors. Host versus graft responses cause transplant rejection. Histocompatibility antigens are the targets for rejection. Minor antigens can be targets of rejection even when donor and recipient MHC are identical. Graft versus host reactions result when donor lymphocytes attack the graft recipient.
• Rejection results from a variety of different immune effector mechanisms. Hyperacute rejection is immediate and caused by antibody. Acute rejection occurs days to weeks after transplantation. Chronic rejection is seen months or years after transplantation.
• HLA matching is one of two major methods for preventing rejection of allografts. The better the HLA matching of donor and recipient, the less the strength of rejection.
• Successful organ transplantation depends on the use of immunosuppressive drugs. 6-MP, azathioprine, and MPA are antiproliferative drugs. Ciclosporin, tacrolimus, and sirolimus are inhibitors of T cell activation. Corticosteroids are anti-inflammatory drugs used for transplant immunosuppression. Antibodies to the IL2 receptor, or to leukocytes, are widely used.
• The ultimate goal in transplantation is to induce donor-specific tolerance. There is evidence for the induction of tolerance in humans and novel methods for inducing tolerance are being developed. Alloreactive cells can be made anergic. Immune privilege can be a property of the tissue or site of transplant.
• Shortage of donor organs and chronic rejection limit the success of transplantation. Living donation is one way to overcome the shortage of donor organs. Alternative approaches are being investigated. The favored animal for xenotransplantation is the pig.
• the first is that transplantation is an important clinical procedure;
• the second is that transplantation has proved an important tool for understanding immunological mechanisms – for example, the major histocompatibility complex (MHC; see Chapter 5) was first described in the context of transplantation, and transplantation models continue to be widely used as tools in basic as well as applied immunology.
In modern practice many transplants are performed routinely (Fig. 21.1). In addition to routine transplantation of the cornea, kidney, heart, lungs, and liver there is increasing interest in transplanting other organs, such as whole pancreas or islet cells for diabetes mellitus and also small bowel.
Genetic barriers to transplantation
Transplantation is normally performed between individuals of the same species who are not genetically identical, and the antigenic differences are known as allogeneic differences, and result in an allospecific immune response (Fig. 21.2).
In the case of autografts and isografts there should be no antigenic differences between donor and recipient, and so no immune response. This can be readily illustrated using transplantation of skin or organs between inbred strains of animals (Fig. 21.3).
Graft rejection
Host versus graft responses cause transplant rejection
Q. What are the main genetic differences that are recognized by the host, and why should this be so?
A. Differences in MHC molecule allotypes are most important. The reason is that all nucleated cells express MHC molecules, and the T cell receptor on host T cells has a basic structure that interacts with and recognizes MHC molecules. Also the MHC class I and class II molecules have an extremely high level of genetic variability (see Chapter 5).
The nature of the host versus graft response is discussed in more detail below.
There is a high frequency of T cells recognizing the graft
Thus, in a naive or unimmunized individual fewer than 1/100 000 T cells respond upon exposure to a virus or a protein immunization; however, 1/100–1/1000 T cells respond to allogeneic antigen-presenting cells (APCs). This is reflected in the strong T cell response (proliferation) seen when naive T cells are stimulated with allogeneic dendritic cells (Fig. 21.4)
Histocompatibility antigens are the targets for rejection
Early experiments showed that the bulk of the allospecific response is against molecules of the MHC. We now know that these molecules are the MHC class I or class II molecules (see Chapter 5), which are responsible for presenting antigen (in the form of peptides) to either:
As discussed in Chapter 8, MHC molecules are highly polymorphic, and it is these polymorphic differences that are seen by alloreactive T cells.
Why is there such a high frequency of allospecific T cells?
The first model (high determinant density model) (Fig. 21.w1) suggests that allospecific T cells recognize the foreign MHC molecules directly, with a low affinity, in a peptide-independent manner. The affinity of the interaction would normally be too low to activate the T cells; however, because the T cells see the MHC molecules directly, and are not recognizing the peptide, this low affinity is compensated for by the high concentration of MHC molecules on allogeneic cells.
Indirect recognition is important in chronic rejection
In a primary alloresponse (see Fig. 21.4), most of the alloreactive CD4 or CD8 T cells directly recognize the donor MHC molecules.
However, there are other forms of alloresponse, including:
• those against minor MHC antigens (see below); or
• the indirect response in which the recipient CD4 T cells recognize donor MHC molecules that have been processed by recipient APCs and are presented as peptides in the context of recipient MHC class II molecules (Fig. 21.5).
Minor antigens can be targets of rejection even when donor and recipient MHC are identical
Perhaps the best studied minor histocompatibility antigen system is the H-Y system. These are antigens encoded by the Y chromosome, and so are expressed only on male cells. Thus, following immunization, it is possible to demonstrate immune responses and rejection of male organs or skin following transplantation mediated by female animals (2X chromosomes) against male cells (X and Y chromosome). It is not possible to show responses against female antigens by male animals because the male animals have one X chromosome, and so are tolerant to all antigens encoded on it (Fig. 21.6).
Graft versus host reactions result when donor lymphocytes attack the graft recipient
GvHD can be lethal, causing damage in particular to the skin and gut. It can be demonstrated in animal models by transfer of bone marrow to irradiated recipient animals (Fig. 21.7). It can be avoided by:
Immune effector mechanisms in graft rejection
Rejection of organs or tissues can occur at various times, each of which is associated with different immune effector mechanisms (Fig. 21.8). These are:
• hyperacute rejection, which occurs within minutes to hours and is principally mediated by antibody;
• acute rejection, which usually occurs in days to weeks in animal models and is initiated by alloreactive T cells; and
• chronic rejection, which is seen months to years following transplantation.
Hyperacute rejection is immediate and mediated by antibody
• the individual has been sensitized to the donor MHC, for example by previous transplants, multiple blood transfusions or pregnancy;
• the animal may have natural pre-existing antibodies (e.g. as a result of ABO blood group incompatibility).
Complement activation can lead to death of the endothelium, or, when the damage is sub-lethal, activation of the endothelial cells. This not only causes an inflammatory response, increasing vascular leakage, but can also cause blood coagulation The result is rapid destruction of the graft (Fig. 21.9).
Acute rejection occurs days to weeks after transplantation
The importance of these dendritic cells can be shown by ‘parking experiments’ in which:
• a kidney is transplanted from one strain of rat to another under cover of immunosuppression (to prevent rejection) (Fig. 21.10);
• the kidney is kept in that animal long enough to ensure that all the resident dendritic cells have migrated out of the organ;
• the kidney is then transplanted into a third animal, of the same strain as the original recipient, where it shows prolonged graft survival.
Once activated the T cells migrate to the organ and lead to tissue damage by standard immunological effector mechanisms (Fig. 21.11). These include:
• the generation of TC cells; and
• the induction of delayed-type hypersensitivity reactions. The role of the T cells in graft rejection can be demonstrated by depletion studies in which antibodies against T cell subsets are administered in vivo. Both of the major T cell subsets, CD4+ and CD8+, can cause graft rejection.
If the animal or patient has already been exposed to the alloantigens expressed by the graft, and as a consequence has been immunized, there will be alloreactive memory cells. This will lead to a much more rapid (accelerated) rejection of the graft (Fig. 21.12).
Chronic rejection is seen months or years after transplantation
In vascularized organs chronic rejection presents as occlusion of blood vessels, which on histological analysis show a thickening of the intima, similar in some respects to the thickening seen as a result of atherosclerosis (Fig. 21.13). Smooth muscle cell proliferation is often seen, together with a macrophage infiltrate (together with some lymphocytes). This eventually leads to blockage of the blood vessels and subsequent ischemia of the organ.
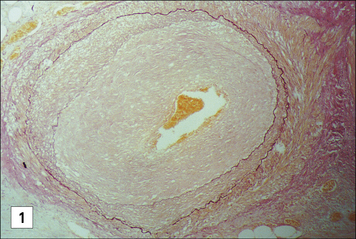
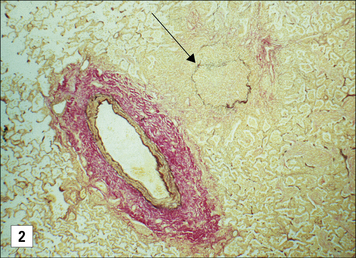
Fig. 21.13 Chronic rejection
(Kindly supplied by Professor Marlene Rose, Imperial College London, Harefield Hospital, and Dr Margaret Burke, Pathology Department, Royal Brompton Hospital and Harefield Hospital.)
A number of mechanisms can lead to chronic rejection. They include:
• a low-grade T cell response (mainly of the indirect allospecific pathway as a result of the loss of passenger leukocytes that activate T cells with direct pathway specificity);
• antibody can also be involved in chronic rejection, as indicated by the deposition of complement components (C4d) in tissues.
Non-immunological processes are also important, such as:
• the response of the graft to injury caused at the time of transplantation or by acute rejection episodes;
• recurrence of the original underlying disease; and
• drug-related toxicities (e.g. the immunosuppressive drug ciclosporin A is nephrotoxic and can damage the kidneys).
Chronic rejection responds poorly to current immunosuppressive therapy. Therefore, although there has been considerable improvement in overall graft survival over the past decades, this improvement is mostly seen in the first year following transplantation – the subsequent survival of grafts has hardly altered over the past 20–30 years (Fig. 21.14). This indicates the need to improve the treatment of chronic rejection.
HLA matching is important to prevent rejection
The two major methods for preventing rejection of allografts are:
• to match the donor and recipient to minimize the antigenic differences; and
• to use immunosuppressive regimens that block the immune response against the organ.
The better the HLA matching of donor and recipient, the less the strength of rejection
Immunosuppressive drugs
6-MP, azathioprine, and MPA are antiproliferative drugs
Ciclosporin, tacrolimus, and sirolimus are inhibitors of T cell activation
Ciclosporin inhibits the production of IL-2
Drugs that inhibit T cell activation are the mainstay of immunosuppressive regimens. The first such drug to be discovered was ciclosporin, a fungal metabolite (Fig. 21.w2).
Sirolimus inhibits signals transmitted by IL-2 binding to IL-2R
The third drug in the ‘inhibitors of T cell activation’ category is sirolimus (rapamycin).
Sirolimus has attracted much attention recently, for two reasons:
• first, because it appears to be a tolerance-permissive drug – that is, if T cells are exposed to antigen in tolerance promoting conditions, such as co-stimulatory blockade, sirolimus allows tolerance to occur, though in contrast there are some data to suggest that the calcineurin inhibitors may inhibit the development of tolerance under similar circumstances;
• second, the broader antiproliferative effects of sirolimus that almost confined it to the waste basket at the outset are now being claimed as beneficial because they reduce the incidence of some malignancies.
Corticosteroids are anti-inflammatory drugs used for transplant immunosuppression
• inhibition of proinflammatory cytokine secretion (IL-1, IL-3, IL-4, IL-5, IL-8 (CXCL8), TNFα);
• inhibition of nitric oxide synthase;
• inhibition of adhesion molecule expression leading to reduced inflammatory cell migration;
• induction of endonucleases leading to apoptosis in lymphocytes and eosinophils.
Antibody therapy
Antibodies have long been used for preventing graft rejection. One of the earliest forms of immunosuppression was anti-lymphocyte globulin or anti-thymocyte globulin; polyclonal antibodies raised by immunizing rabbits or horses with lymphocytes or thymocytes. These antibodies deplete circulating T cells. These are still used, though they are increasingly being replaced with monoclonal antibodies. The first monoclonal antibody licensed for any purpose was Muromonab-CD3 which was approved by the FDA in 1986 for the treatment of rejection episodes following renal transplantation. This binds to CD3 on T cells, and suppresses T cell responses. The action of Muromonab binding CD3 can result in a cytokine release syndrome on infusion, caused by the secretion of inflammatory cytokines by the T cells following engagment of CD3. Two anti IL2 receptors (daclizumab and basiliximab) are now widely used in transplantation, as part of standard immunosuppressive agents. These antibodies operate by blocking the IL2 receptor, rather than depleting T cells. Alemtuzumab (commonly known as CAMPATH-1 H), which depletes CD52 expressing leukocytes, has been widely used for transplantation though it is not yet licensed for that application. Other antibodies are being investigated for particular applications in transplantation (for example the depletion of B cells). Antibody like molecules (e.g. abatacept and belatacept which are two forms of a fusion protein of CTLA4 with the Fc portion of an antibody (see Chapter 8)) are also being evaluated for clinical use.
Induction of donor-specific tolerance
• the non-specific toxicity of the drugs;
• the need to stay indefinitely on medication; and
• the consequences of generalized immunosuppression such as the increased incidence of cancer and infection.
• performing a second graft (so showing that the immune system will no longer respond to the antigen); or
• demonstrating that an irrelevant third party graft is still rejected (demonstrating that graft survival is not due to a generalized immunosuppression).
However, this is more difficult in humans.
There is evidence for the induction of tolerance in humans
There are two sources of evidence for the induction of tolerance in humans.
Second, it is possible to look at the frequency of alloreactive T cells in patients with grafts. In some groups there is a reduced frequency of these cells, but the response to other antigens remains normal (Fig. 21.15). Again, this is not a formal proof of tolerance because it is not yet known how the in-vitro assays relate to the response in patients. However, it does indicate that it might be possible to develop tests that will allow us to monitor the development of tolerance in patients, and so know how to tailor treatment to the individual (e.g. removing them from immunosuppression when indicated).
Novel methods for inducing tolerance are being developed
There are various ways in which tolerance (or the appearance of tolerance) can occur. Most of these are discussed in more detail in Chapter 19. An understanding of the mechanisms by which tolerance is induced and maintained allows the development of novel methods for inducing tolerance.
Alloreactive cells can be made anergic
Blockade of co-stimulatory molecules such as CD80 and CD86 with agents like CTLA-4–Ig (a fusion protein between CTLA-4, a ligand for CD80 and CD86, and the Fc part of an antibody molecule) can be used to induce anergy in alloreactive cells (Fig. 21.16). However, it should be noted that the situation can be more complex than this. In many APCs cross-linking of CD80 and CD86 with CTLA-4–Ig results in upregulation of an immunomodulatory enzyme indoleamine 2,3-dioxygenase (IDO). This enzyme catabolizes tryptophan, and as a result prevents T cell activation both as a result of:
• depriving T cells of this essential amino acid; and
• the products of tryptophan breakdown acting directly on the T cells.
Another alternative is to induce a regulatory response to the alloantigen. The phenomenon of T cell regulation has long been recognized (Fig. 21.17), and can be shown in experimental models by transferring T cells from a tolerant animal to a naive recipient, and showing that this results in a transfer of the tolerance. Several types of T cell are capable of regulating the immune response, and strategies that seek to expand these cells may be one method to induce tolerance in vivo.
Immune privilege can be a property of the tissue or site of transplant
Although a failure to reject a graft could be the result of tolerance (or immunosuppression), an alternative is that the graft is immune privileged and protected from the immune response against it (see Chapter 12):
• when corneal transplantation is performed in an animal model the rejection response is weaker than for other forms of transplantation, indicating the immune privileged nature of the graft;
• skin transplanted onto the anterior chamber (where the cornea is) shows a lower rate of rejection than skin transplanted into other sites.
Limitations on transplantation
Two major issues limit success of transplantation:
• the first is the shortage of donor organs – this means that not everyone who would benefit from a transplant receives one, and, given the high success rate of transplantation, an increasing number of patients would benefit;
• the second problem is chronic rejection, which results in a continual loss of transplanted organs and necessitates patients remaining on immunosuppression, with the consequences of drug toxicity, systemic immunosuppression, increased incidence of malignancy, and infection.
• we develop assays that will allow us to determine when tolerance has been induced; and
• translate the therapies developed in animal models to the clinic.
Critical thinking: Kidney transplantation (see p. 440 for explanations)
1. What are the difficulties in finding a donor organ?
2. Comment on the HLA relationships between Mrs X and her brothers.
3. Comment on the relationships between the children of Mrs X.
4. Classify each member of the family in terms of their HLA relationship to Mrs X (HLA identical, HLA haplotype match, complete HLA mismatch).
5. In terms of HLA matching alone, who was the best donor for Mrs X?
6. Consider what effect the blood group antigens had on the choice of donor. From whom could kidneys have been transplanted, and who would not have been suitable?
7. Of those who had a compatible blood group, who would you have chosen as the best donor? Explain your reasoning.
8. The outcome of the transplantation was a disaster! By what mechanism was the graft attacked?
9. Why was Mrs X at a greater risk of this untoward reaction?
10. What laboratory tests are used to avoid this rejection reaction, and what seems to have gone wrong on this occasion?
11. Four years after the first transplant it was decided to try again with a living related donor. Of all the family members, who would you have chosen as the donor and whose kidney was most likely to survive in Mrs X?
12. What is triple therapy immunosuppression?
13. What type of rejection occurred at 3 weeks after transplantation, and what immunological mechanisms were involved?
14. What is anti-rejection therapy?
15. There were no other problems with Mrs X. Can you think of some of the problems that might arise in a transplant recipient?
16. Why did the function of the transplant gradually decline, and why could the doctors not stop this process?