CHAPTER 65 Topectomy and Multiple Subpial Transection
Topectomy
The prevalence of epilepsy is approximately 1%. In the majority of cases, it can be effectively treated medically with antiepileptic drugs, but 30% to 40% of all new epilepsy patients will ultimately become refractory to treatment.1 Some investigators report that ongoing medication trials can be a satisfactory approach to managing refractory epilepsy.2 However, it is important to consider surgery for such patients.3
In the Montreal Neurological Institute series covering the period from 1929 to 1980, the anatomic distribution of surgical resection in 2177 patients was as follows: temporal, 56%; frontal, 18%; central, 7%; parietal, 6%; occipital, 1%; and multilobar, 11%.4
“Topectomy” generally indicates resection of focal cerebral cortex from the frontal, parietal, or occipital lobe. Outside the temporal lobe, localization of the seizure origin is much more difficult, seizure outcome is less favorable, and surgery in some brain sites is associated with higher risk for major morbidity than with temporal lobe resection.5 Although temporal lobe resection is much more common in modern epilepsy practice, topectomy is the foundation of modern epilepsy surgery.6 In 1886, Sir Victor Horsley performed what would now be considered lesionectomies with excellent results. Before the invention of electroencephalography (EEG) in 1928, epilepsy surgery was exploratory and based on clinical localization pioneered by Jackson and identification of visibly abnormal tissue. EEG removed this requirement and decreased negative explorations considerably through the 1950s and 1960s.7 Magnetic resonance imaging (MRI) now provides a priori knowledge of structural cortical abnormalities. Table 65-1 shows types of procedures for epilepsy.
A fundamental concept underlying focal cortical resection is that the epileptic focus contains electrically abnormal neurons that are the source of seizures. Hence, if this epileptogenic focus can be accurately identified and resected, the seizure will cease. Epileptogenicity is assumed to derive from physiologic events that occur within the cell body and dendrites of neurons rather than axons.8 Therefore, resection of only epileptogenic gray matter (cerebral cortex) rather than white matter should in theory be sufficient to eliminate seizures. Recent work suggests that epilepsy may arise as a result of abnormalities of a distributed cellular network.9 Therefore, intrinsic cellular abnormalities may not be required to create an epileptic condition. The most characteristic and ubiquitous pathologic changes are the presence of gliosis, loss of neurons, and dendritic abnormalities.10,11 This chapter reviews the principles and methods of surgical localization and cortical resection in patients who may be candidates for topectomy or multiple subpial transection (MST).
Patient Selection
In general, surgical treatment of epilepsy should be considered when (1) the seizures have not been controlled by adequate attempts at treatment with maximally tolerable doses of correct anticonvulsant medications; (2) the seizures interfere with psychological and intellectual development, employment, or social performance; (3) all potentially epileptogenic areas have matured, the seizure tendency (pattern and frequency) is stable, and there is no tendency toward spontaneous regression; and (4) the patient must be strongly motivated to cope with an exhaustive diagnostic regimen and a lengthy operative procedure, possibly under local anesthesia.12–14 Chronic psychosis is a contraindication to surgical treatment, but epilepsy-related acute psychosis is not. Mental retardation is not a contraindication.15,16
The length of time that patients have seizures before being referred for surgery has become shorter as referring physicians recognize that superior surgical results may be obtained when the period of uncontrolled seizures is shortened, particularly in children.17 Currently, adequate, but unsuccessful antiepileptic drug therapy for 1 to 5 years may be a sufficiently long trial period to warrant referral for consideration of epilepsy surgery.16 In exceptional cases, urgent cortical resection may be considered for the relief of status epilepticus.18,19
Table 65-2 is a representative list of neurological diagnoses that may be considered for surgery involving focal cortical resection.20 Topectomy can be considered when the seizure arises from a focal and functionally silent area of the brain, which usually means that the focus is not in the speech or motor cortex. Additionally, neuropsychological testing is performed to identify any functional deficits that may be related to the epileptic cortical region.21 Multiple widespread or bilateral foci are generally contraindications to resective surgery. A series of diagnostic studies, including EEG, are necessary to confirm the site of seizure onset. In eloquent brain regions, MST may be considered.
Presurgical Evaluation
The optimal surgery for epilepsy is resection of just enough neuronal tissue to eliminate the patient’s seizures without causing unacceptable neurological deficits. Presurgical evaluation enables the surgeon to determine the volume of cortex involved in seizure initiation and propagation and whether it can be safely resected. As stated by Rasmussen, epileptogenic lesions outside the temporal lobe are considerably more varied in extent and geographic configuration than the more common temporal lobe epileptogenic lesions.22 A variety of diagnostic tests are used for this purpose, but there is no consensus on how much information is actually needed before a particular surgical intervention can be recommended.23
The initial preoperative evaluation process includes careful examination of clinical seizure characteristics, ictal and interictal scalp EEG, neuroimaging, and neuropsychological testing. The results of this testing should provide considerable lateralizing and localizing information. Video-EEG monitoring is the “gold standard” of the evaluation. When noninvasive tests do not show the source of seizures clearly, invasive testing is considered. Localization of the seizure focus is difficult in patients with extratemporal epilepsy, and invasive EEG is almost always required.5
Noninvasive Evaluation
Symptomatic Localization
In frontal lobe epilepsy, clinical localization of the seizure origin is difficult because the ictal behavioral manifestations in patients with frontal lobe epilepsy are largely due to spread of seizures to neighboring or distant functionally connected brain regions.24 Rasmussen characterized six distinct convulsion patterns, some of which he believed indicated a frontal lobe focus:25,26
In parietal lobe seizure, most patients have attacks consisting of unilateral motor or sensory phenomena with additional features such as dizziness, cephalic sensation, contraversion, perceptual illusions, informed visual hallucinations, mental confusion, epigastric sensation, dysphasia, or automatisms.26,27
In central epilepsies, the attacks are somatomotor or somatosensory. The attacks sometimes remain localized, but in most patients a certain portion of the attacks progress to generalized convulsive seizures. Epilepsia partialis continua is particularly common.26,27
In occipital lobe seizure, visual symptoms such as transient blindness or visual hallucinations are usually characterized by flashes of lights, colored balls, and other geometric patterns. These symptoms have a strong relationship with seizure activity.26
However, some initial seizure symptoms may not reflect the region of seizure origin because many cortical regions are clinically silent. Seizures originating in these regions produce signs and symptoms only after spreading outside the epileptogenic region.27
Extracranial Electroencephalography
Scalp EEG often provides important information about the location and size of the epileptogenic area. An interictal epileptiform abnormality consisting of spikes, spike and slow wave complexes, sharp waves, and sharp and slow wave complexes repeated on several occasions may be particularly informative.28 Activation procedures such as withdrawal of medication, hyperventilation, or drug-induced sleep may enhance abnormalities or even provoke a seizure.13
In addition, long-term video-EEG monitoring is generally performed in an epilepsy monitoring unit to correlate clinical seizure activity with the accompanying electrical abnormalities with the intent of identifying the seizure origin electrographically.5 The specific ictal symptomatology can help greatly in some cases in which localization of the focus is problematic. The EEG pattern of seizure onset is most frequently rhythmic, fast, spike-like discharges, often building in amplitude.9
Neuroimaging
MRI is the imaging modality of choice in patients with intractable partial epilepsy. Computed tomography (CT) is also helpful because it demonstrates intracranial calcifications more clearly than MRI does. MRI provides visualization of focal dysplastic cortical lesions in many patients previously assigned a diagnosis of cryptogenic neocortical epilepsy.5,29 An MRI-identified lesion may prove to be the cause of the seizure disorder. These abnormalities are then correlated with the results of electrophysiologic studies to accurately identify the source of the patient’s seizures.30 MRI is also useful in the diagnosis of structural and developmental epileptogenic pathologies. The most common findings in this category are ischemic lesions or developmental abnormalities, specifically, neuronal migration disorders.31 Structural lesions include ischemic infarctions (prenatal, perinatal, or postnatal), Sturge-Weber syndrome, Rasmussen’s encephalitis, and others. Progressive unilateral cortical atrophy is demonstrated in patients with Rasmussen’s encephalitis. Unilateral megalencephaly is one characteristic example of a developmental pathology associated with seizures. The most common neuronal migration disorders are lissencephaly and diffuse pachygyria. The complete absence of gyri and sulci in lissencephaly and the development of a few thick, wide gyri separated by shallow sulci in diffuse pachygyria are clearly identifiable on MRI.31
Ictal perfusion single-photon emission computed tomography (SPECT) may demonstrate increased blood flow at the site of seizure onset.15,32–34 The tracer isotope (usually technetium 99m–labeled hexamethyl-propyleneamine-oxime [HMPAO]) is administered immediately after seizure onset, and the scan should be performed within several hours. There are some reports of localized hyperperfusion in ictal studies of patients with frontal, parietal, or occipital epilepsy.15,16 Interictal SPECT has been of only limited value; it occasionally shows local reduced blood flow.33 Timing demands and other technical issues associated with SPECT scanning present barriers to its use. There is some evidence that functional anatomic correlation is more reliable with a technique called SISCOM, an acronym for subtraction ictal single-photon tomography coregistered to MRI.35
Interictal positron emission tomography (PET) with a tracer for glucose metabolism (18F-fluorodeoxyglucose) has regularly demonstrated hypometabolism in the epileptic focus of temporal lobe epilepsy.15,36 However, PET is less reliable in identifying extratemporal, nonlesional foci.29
Neuropsychological Testing
Neuropsychological examinations contain a personality inventory and tests of memory, language function, and intelligence. These tests are useful in localization and lateralization of speech, memory, spatial, cognitive, and cerebral dominance functions. They also evaluate mental states such as mental retardation.29,37 These traditional roles of neuropsychological evaluation are now augmented by the discipline’s ability to predict cognitive and behavioral outcomes after surgery.22 Additionally, neuropsychology can have therapeutic benefit as well,38 and it can be combined with advanced imaging techniques to make highly specific predictions about cognitive function after epilepsy resection.39
Invasive Evaluation
The Wada Test
The Wada test (intracarotid amobarbital [Amytal] test) is performed to identify the dominant hemisphere for language functions and to determine the degree to which memory functions are subserved by each hemisphere.40 In the series from the Montreal Neurological Institute, in right-handers, 96% had speech lateralized to the left cerebral hemisphere and 4% to the right hemisphere. In left-handed or ambidextrous individuals 70% had speech on the left, 15% had it on the right, and 15% had some representation of speech in each hemisphere. Therefore, the Wada test is necessary in all left-handed and ambidextrous patients and in right-handed patients with some ambiguous evidence of lateralization from psychological tests, x-ray films, EEG studies, or the seizure pattern.13 Unusual language lateralization can be seen in patients who sustain left hemisphere injury in early life or have early onset of intractable epilepsy.15 Recently, work has been done to supplant the invasive Wada test with functional MRI (fMRI).41,42 Although fMRI has some cost and risk advantage,43 the diagnostic accuracy of the technique is insufficient to completely supplant the WADA test at this time.
Invasive Electroencephalography
When an epileptogenic focus is not clearly lateralized, invasive EEG monitoring is necessary for cortical resection. In extratemporal epilepsy, subdural strip electrodes are used primarily to lateralize an epileptogenic focus (Fig. 65-1), and large subdural grids are used to define the limits of a focus that has already been lateralized but not sufficiently localized (Fig. 65-2). It is important to cover as much of the suspected area as possible for accurate lateralization and localization.29,44 In our center, we routinely identify electrode locations in image space by coregistering postoperative MRI and CT images.45 If the electrodes are properly indexed in the image, an electroradiographic record of the eloquent and epileptogenic regions can be obtained and used as a navigational tool during resection.
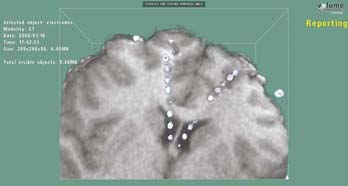
FIGURE 65-2 Cingular depth electrodes from the same patient as in Figure 65-1. The patient had intractable frontal lobe epilepsy. The goal was to determine from which side the seizures arose.
Subdural strip electrodes are placed through a bur hole and passed blindly into the subdural space. Multiple electrodes may be inserted through one bur hole to cover wide regions of the brain. In patients with bifrontal discharges, strip electrodes are placed over the medial and lateral surfaces of the posterior frontal lobe from bur holes at the coronal suture, just off the midline (see Fig. 65-1).15
Subdural grid electrodes are placed with a craniotomy (see Fig. 65-2). They are used not only for determining the site of seizure onset over the convexity of one hemisphere but also for extraoperative functional mapping (motor, sensory, speech, and so on) by stimulation of each electrode. The maximal extent of an epileptogenic focus and areas of cortical function are determined with these evaluation methods.4 With invasive EEG methods, the electrode leads are brought out through the scalp and the patient is monitored for many days. The most common complications are infection and leakage of cerebrospinal fluid, especially with a large subdural grid.42
Surgical Topectomy Procedure
General Principles from Anatomic Considerations
Anatomically, the frontal lobe Broca speech area is identified in the opercular, inferior frontal gyrus (usually the posterior 2.5 cm of this gyrus). It is difficult to identify Wernicke’s area by anatomic criteria. The parietal speech area is identified 1 to 4 cm above the sylvian fissure and 2 to 4 cm behind the postcentral sulcus. The temporal speech areas usually extend posteriorly behind the level of the postcentral sulcus and 2 to 3 cm from the adjacent convolution above, behind Heschl’s gyri. Lack of defining anatomic features for Wernicke’s area renders language mapping essential for cortical dominant hemisphere resections.13,14,46
Large frontal resections in the nondominant hemisphere can be carried out in front of the precentral gyrus. Identification of the precentral and postcentral gyri is best accomplished by stimulation under anesthesia without neuromuscular blockade.4,27 It can also be done more coarsely by identifying the somatosensory evoked potential (SSEP) phase reversal over the central sulcus.47 Resection of precentral arm or leg motor cortex is permitted only if significant contralateral paresis is already present.15,47 The lower precentral face area can be resected. The resulting contralateral facial paresis improves but may not return to normal.27
Resection of the postcentral sensory arm or leg area causes a profound proprioceptive deficit and is rarely indicated, although improvement over a period of several months is possible.27,47 Conversely, the lower 2.5 to 3 cm of the postcentral face area can be resected without significant deficit as long as the resection does not extend into the underlying white matter.27 In the nondominant hemisphere, the entire parietal cortex posterior to the postcentral gyrus can be removed without inducing a sensorimotor deficit.27,47,48 Resection in the parietal operculum may produce contralateral lower quadrantic hemianopia if resections are carried beyond the depths of the sulci into the white matter.47 In the dominant hemisphere, parietal lobe resections should be limited to the superior parietal lobule. Language functions are subserved by cortex of the inferior parietal lobule, and a disabling Gerstmann syndrome can also result from extensive parietal lobe resection.27
Large resections of occipital cortex produce a contralateral homonymous hemianopia. Therefore, if vision is intact preoperatively, the calcarine cortex and optic radiations are spared as much as possible. Because cortex essential for reading is often more widespread than that for naming, excision within 2 cm of Wernicke’s area may cause a persisting dyslexia.47
The vascular territory of each crucial artery or vein should be studied to assess the consequences of occlusion of the vessel during surgery. This approach is essential to minimize morbidity, especially with surgery on the motor and speech areas. Any ascending vein to the superior sagittal sinus draining from the central or postcentral sulci should be left intact to avoid significant morbidity.4,27
Preoperative Care and Anesthesia
It is our practice to reduce the doses of antiepileptic medications the week before surgery so that the epileptogenic cortex is as active as possible during surgery.13 Some epilepsy centers do not use this strategy, particularly in situations in which they will be carrying out awake craniotomies. When resecting noneloquent cortical areas, general anesthesia can be used.27 However, when intraoperative electrocorticography (ECoG) is required, the use of drugs that depress cortical electrical activity, such as benzodiazepines and barbiturates, should be avoided. In addition, when functional mapping of speech and sensory areas is performed, the patient should be conscious and cooperative during the procedure. In this situation, local and total intravenous anesthesia with analgesic drugs (fentanyl and droperidol or propofol) should be used.14,49 Local anesthesia alone has the disadvantages of taking more time to create a complete block and limiting the range of head positions that can be used. Furthermore, it cannot be used with uncooperative patients and young children. Constant supervision by a specially trained anesthesia team is essential.4,27
Intraoperative Electrocorticography
Sufficient brain exposure via craniotomy is essential during ECoG. ECoG is performed to further delineate the extent of the epileptogenic zone. The intention is to identify regions with primary epileptic neurons by identifying brain sites that have interictal ECoG spikes. In our experience, there is a clear relationship among the site of interictal discharges, the site of ictal onset, and the tissue that must be removed to control seizures. This ECoG hallmark is used to determine what part of the brain should be resected.4,15,50
ECoG also provides prognostic information by indicating areas with residual discharges after cortical resection. Patients with no interictal discharges on postresection recordings are more likely to be free of seizures than those with persisting discharges.4,51,51 For “standard” temporal lobectomy surgery, the value of ECoG is not as clear.52
Cortical Stimulation (Functional Mapping)
The location of the central sulcus is determined by electrical stimulation of the precentral and postcentral gyri after preliminary identification by monitoring for the SSEP phase reversal.48 The suspected site of the motor and sensory cortex is stimulated and mapped with a motor response detected by the anesthetist, measurement by electromyographic electrodes, or a report of sensory change by the patient.15,47,51 In practice, the best way to identify the postcentral gyrus is to induce sensory responses in the tongue area located at the bottom of the postcentral gyrus.27,53
The frontal, parietal, and temporal language areas in the dominant hemisphere are stimulated while the patient carries out simple verbal tasks such as naming objects shown on picture cards. A language critical area is identified if the patient is unable to speak (speech arrest) when the site is being stimulated or if the patient can speak but is unable to name objects.14,15,47,51