Chapter 11 Thoracic Aorta
There are four main lesions of the thoracic aorta that present for management: dissection, aneurysm, rupture, and aortic coarctation. The focus in this chapter is on the first two of these conditions; traumatic aortic rupture is covered in Chapter 25, and aortic coarctation is covered briefly in Chapter 15.
ANEURYSMS OF THE THORACIC AORTA
Etiology
The causes of thoracic aortic aneurysms are summarized in Table 11-1. Atherosclerotic degeneration is the most common cause of aneurysms involving the descending thoracic and thoracoabdominal aorta. Risk factors include increased age, smoking, and hypertension.
Degeneration |
Hypertension |
Atherosclerosis |
Cystic medial necrosis |
Genetic or congenital origin |
Marfan syndrome |
Ehlers-Danlos syndrome |
Bicuspid or unicuspid aortic valve |
Inflammation |
Takayasu arteritis |
Behçet arteritis |
Kawasaki disease |
Polyarteritis |
Infection |
Aortic stenosis |
Aneurysms of the proximal ascending aorta may result from chronic flow turbulence due to aortic valve stenosis (poststenotic dilatation). In addition, patients with bicuspid or unicuspid aortic valves are at increased risk for aortic aneurysm formation due to primary structural abnormalities of the aortic wall.1 Thoracic trauma, usually a sudden deceleration injury, is a rare cause of chronic aneurysm formation. This is usually caused by intimal disruption in the area of the aortic isthmus. A pseudoaneurysm (false aneurysm) is a collection of thrombus and connective tissue outside the aortic wall that occurs as a result of a contained rupture. Pseudoaneurysms typically occur secondary to infection, trauma, or previous aortic surgery.
Classification
Aneurysms of the thoracic aorta can be classified on the basis of their anatomic position. Ascending aortic aneurysms arise anywhere from the aortic valve to the innominate artery. Aortic arch aneurysms involve aorta bounded by the brachiocephalic vessels. Descending thoracic and thoracoabdominal aneurysms arise distal to the left subclavian artery. Thoracoabdominal aneurysms are further divided according to the Crawford classification (see Fig. 11-1).
Natural History
An aortic aneurysm is a slowly progressive but intrinsically lethal condition that eventually undergoes rupture or dissection. The primary determinant of adverse outcome is aneurysm size: a patient with a maximal aortic dimension of 6 cm has an annual risk for rupture, dissection, or death of 14%.2
The growth rate of aneurysms is not linear, tending to accelerate as the aneurysm increases in size (see Wall Stress and Aneurysm Growth in Chapter 1). Aneurysms grow faster in patients who smoke, in patients who are hypertensive, in aortas with dissections, and in the thoracic aorta rather than the abdominal aorta.
Surgical intervention is recommended when the maximal aortic diameter exceeds 5.5 cm in the ascending aorta and 6 to 6.5 cm in the descending aorta.2,3 These recommendations are based on the relative risks for rupture or dissection compared with the risks involved in surgery.
Aneurysms caused by Marfan syndrome display accelerated unpredictable growth patterns and have a tendency to rupture or dissect at a smaller size than do aneurysms in patients without Marfan syndrome. This is particularly the case if there is a family history of early complications. Surgery is recommended for patients with Marfan syndrome when the maximal diameter exceeds 5 cm in the ascending aorta and 6 cm in the descending aorta.2
Clinical Features and Investigations
The aneurysm may be apparent on a chest radiograph. With an ascending aneurysm, there may be a prominent right aortic border on the frontal projection and filling in of the upper substernal space on the lateral projection. With an arch aneurysm there may be widening of the mediastinum on the frontal projection. An enlarged descending thoracic aorta may be seen behind the heart on the frontal and lateral projections (Fig. 11-2).
Imaging of thoracic aortic aneurysms may be undertaken by echocardiography, computed tomography (CT), or magnetic resonance (MR) imaging. Echocardiography allows accurate assessment of aortic regurgitation, aortic leaflet morphology, and ventricular function, but it may not fully delineate the anatomic extent and relationships of the aneurysm. Echocardiography is particularly poor at imaging the distal ascending aorta and aortic arch. To image the aneurysm itself accurately, either a contrast CT scan (Fig. 11-3A) or MR imaging scan is required. For patients undergoing aneurysm surveillance, conventional axial CT scanning is sufficient. For operative planning, CT or MR imaging with three-dimensional reconstruction is extremely helpful (Fig. 11-3B).
Treatment of Thoracic Aneurysms
In patients with small aneurysms, regular surveillance by CT scanning is indicated; the frequency of scanning is determined by the size and growth pattern of the aneurysm. Medical treatment primarily involves control of hypertension. β Blockers are a good choice for blood pressure control because they reduce tension in the aneurysm wall and, unlike arteriolar vasodilators, do not increase shear forces in the aorta.4 Drug treatment of hyperlipidemia and diabetes may also be indicated. Smoking cessation, diet, and exercise are all important preventive strategies for atherosclerosis.
Preoperative Assessment
Aortic aneurysms and dissections typically occur in older patients with histories of smoking, hypertension, and chronic obstructive pulmonary disease. There is frequently coexisting coronary artery, carotid artery, and peripheral vascular disease. Furthermore, open repair of thoracic aortic aneurysms imposes a huge physiologic insult on patients. Thus, it is essential to fully investigate and optimize patients prior to surgery.
An echocardiogram should be performed on all patients; with particular emphasis on aortic valve and ventricular function. Patients over 40 years of age and those with risk factors for coronary artery disease should undergo coronary angiography.5
More than one third of patients undergoing surgery for thoracic aortic aneurysms have either a diagnosis of chronic obstructive pulmonary disease or a history of heavy smoking.6 These patients should have spirometry and arterial blood gases performed preoperatively. They are also likely to benefit from a period of smoking cessation, chest physiotherapy, and appropriate treatment of the infective and bronchospastic components of their respiratory diseases. The management of patients with obesity, renal failure, carotid disease, and diabetes is similar to that outlined in Chapter 9.
AORTIC DISSECTION
Intramural hematoma is due to a burst vessel in the media, causing a hematoma, which may then lead to a tear and communication with the aortic lumen. A penetrating atherosclerotic ulcer is ulceration within an atherosclerotic plaque that extends down to the level of the media. Both intramural hematoma and penetrating atherosclerotic ulcer have high incidences of dissection and rupture.7,8
Etiology
The causes of aortic dissection are similar to the causes of aortic aneurysm (see Table 11-1). Indeed, dissection, intramural hematoma, and atherosclerotic plaque rupture can all complicate an aortic aneurysm. An additional cause of aortic dissection is iatrogenic aortic injury due to surgical manipulations such as the placement of aortic cannulas during cardiopulmonary bypass.
Classification
Most aortic dissections begin in the ascending aorta and extend distally, although between 5% and 10% begin in the arch, and 30% to 35% begin in the first part of the descending aorta. There are two main classification systems, based on the extent of aortic involvement (Fig. 11-4). The Stanford classification is used here.9
Clinical Features and Investigations
Type A Dissection
Type A aortic dissections can result in the following complications:
Patients commonly present with shock due to the combined effects of pericardial tamponade and aortic regurgitation. In the absence of these complications, patients may be hypertensive. Patients may develop a major neurologic deficit, such as impaired consciousness, hemiplegia, paresthesia, or paraplegia. Pulses (brachial, carotid, femoral) may be weak, absent, or asymmetric. Four-limb blood pressure measurements may show marked variability. The chest radiograph may demonstrate widened mediastinum and loss of the aortic knuckle (Fig. 11-5).
Imaging
Patients who present with symptoms and signs consistent with aortic dissection require urgent diagnostic imaging. Options include transesophageal echocardiography (TEE), CT scanning, MR imaging, and aortography, all of which have a sensitivity and specificity greater than 90%.10 Intramural hematoma may be difficult to diagnose with any of these techniques. The preferred initial investigation varies according to the institution, but CT scanning is the most widely used modality.11 CT scanning has the advantage of being rapid (unlike MR imaging) and does not require sedation or general anesthesia (unlike TEE). Aortography is rarely indicated other than as part of a stenting procedure. CT scanning demonstrates the extent of the dissection (Fig. 11-6) and the presence of any pericardial blood but does not usually demonstrate the entry tears or any associated aortic regurgitation.
TEE is the most useful investigation for determining the severity of any associated aortic regurgitation (Fig. 11-7) and may provide information on the site of the entry tears. TEE is also useful for identifying pericardial effusions and myocardial ischemia. The administration of intravenous sedation for TEE in a patient with suspected dissection is hazardous because it may precipitate either hypotension (due to the effects of sedative drugs in a patient with pericardial tamponade) or hypertension (due to patient anxiety).
Management
Type A Dissection
Patients with type A dissection should be taken to the operating room for immediate surgical repair because they have a mortality rate of 1% to 2% per hour after the onset of symptoms.12 Without surgery, more than 25% of patients with type A dissections die within 24 hours, and 58% die within 30 days. Surgical mortality is approximately 10% at 24 hours, increasing to 26% at 30 days.12 Patients with pericardial tamponade, coronary artery involvement, or involvement of the cerebral vessels are at greatest perioperative risk. Iatrogenic dissections during cardiac surgery have a mortality rate of 35%.13 Intramural hematoma and ulcerating atherosclerotic plaque in the ascending aorta should be managed as aortic dissection.7,8
Type B Dissection
Type B dissections should be managed medically unless complications occur. Uncomplicated type B dissections have a mortality rate of 10% at 30 days.12 Once this diagnosis has been made, patients should be admitted to the intensive care unit. Management involves pain relief and aggressive control of hypertension. A target blood pressure of 100 to 110 mmHg systolic is reasonable. This may be achieved by an intravenous β blocker (e.g., metoprolol) and, if necessary, addition of a vasodilator such as nitroglycerin. Once blood pressure is under effective control by means of intravenous therapy, oral treatment is instituted, usually including a β blocker and either an ACE inhibitor or a calcium channel blocker.
Surgical intervention for type B dissection is reserved for patients who develop complications, such as an enlarging or extending dissection or a contained leak into the mediastinum. Persistent or recurrent pain is highly suggestive of expansion of the dissection and indicates the need for urgent imaging of the aorta. Peripheral or mesenteric ischemia may also require surgical intervention. In many circumstances the complications arising from a type B dissection can be managed with endovascular stent grafting, percutaneous fenestration of a dissection flap, or both (see later material). Up to 40% of patients with type B dissections develop complications. Thus, regular CT scans, initially 3 to 6 monthly, are indicated following discharge.
AORTIC SYNDROMES: ENDOVASCULAR STENT GRAFTING
Endovascular stent grafting was first described for the treatment of abdominal aortic aneurysms.14 Subsequently, the technique has been applied to the thoracic aorta for the treatment of aneurysms, pseudoaneurysms, complicated type B dissections, and traumatic ruptures.15–21 Both acute and chronic aortic syndromes may be managed with endovascular stent grafting. Endovascular stent grafting may also be combined with open repair for lesions involving the ascending aorta and aortic arch. In many centers, endovascular stent grafting is now the preferred technique for the management of aortic syndromes involving the descending thoracic aorta. In the United States, adoption of the technique has been limited, until recently, by the lack of a device approved for use in the thoracic aorta.
Endovascular stent grafting involves placement of one or more self-expanding prostheses (Fig. 11-8) within the aorta under radiographic guidance. The device is usually inserted percutaneously via the femoral or, occasionally, the iliac artery. If endovascular stent grafting is used in combination with an open procedure, the graft may be placed under direct vision at the time of surgery, with the proximal aorta open during hypothermic circulatory arrest. The suitability of a patient for stent graft placement is determined by the location and morphology of the lesion, the tortuosity of the abdominal and thoracic aorta, and the size of the vascular access artery. Proximal and distal “landing” sites for the graft must be a minimum of 20 mm long, free from disease and, ideally, not overlying a critical branch artery. In many cases, exclusion of the left subclavian artery is not associated with arterial insufficiency of the left upper limb. If the planned landing site covers a critical branch artery (e.g., the left common carotid artery), a revascularization procedure (e.g., connection of the left and right common carotid arteries) must precede stent deployment. Where possible, endovascular stents should avoid covering the aorta between the levels of the T10 and L2 vertebrae. The celiac trunk arises from the aorta at the T10 level. The artery of Adamkiewicz is a large intercostal artery that supplies the anterior spinal artery; interruption of flow down this artery may result in paraplegia (see subsequent material). This artery has a variable origin but usually arises between T8 and L2.
With a type B dissection, placement of the stent graft across the primary entry tear is sufficient to control flow and allow thrombus formation in the false lumen; it is not necessary to cover the entire length of the dissection. If the true lumen is compressed by an enlarging false lumen then the stent graft may improve flow within the true lumen.22 Occasionally, branch arteries are supplied exclusively from the false lumen. In this situation, a fenestration may be created between the true and false lumens to improve end-organ perfusion.
The primary advantage of aortic stent graft placement is the avoidance of an open surgical repair. To date, there have been no large randomized, controlled trials comparing endovascular stent grafting with open repair for thoracic aortic syndromes. Data from numerous case series indicate that endovascular stent grafting is feasible and has a low, but not negligible, incidence of serious morbidity and mortality rates. In one small case-controlled study, a reduction in 30-day mortality rates and a reduced hospital cost was observed in patients treated with stent graft repair of descending aortic pathology compared to patients undergoing open repair.23 However, the long-term durability of endovascular stent grafting of the thoracic aorta is not known, and there are additional costs associated with long-term CT surveillance following stent grafting. One difficulty with research in this area is that the devices are rapidly evolving. Thus, when long-term follow-up studies are reported, they may not be applicable to devices in current use.
Postoperative Care Following Endovascular Stent Grafting
After uncomplicated endovascular stent graft repair, patients are typically awakened from anesthesia and transferred to a high-dependency environment for 12 to 24 hours of monitoring. Slight to moderate back pain and transient postimplantation syndrome, with low-grade fever, leukocytosis, and raised C-reactive protein are common.18
A number of serious early and late complications have been reported following endovascular stent grafting (Table 11-2). Postoperative renal failure may develop for a number of reasons, including preexisting renal dysfunction, exposure to radiographic contrast agents, intraoperative hemodynamic instability and, rarely, stent occlusion of a renal artery. Urine output should be measured hourly for 24 hours postoperatively. In patients with preexisting renal impairment, the administration of N-acetylcysteine prior to the procedure should be considered (Chapter 33).
Table 11-2 Complications of Endovascular Aortic Stent Grafting
Immediate | Delayed |
---|---|
Renal failure | Endoleak |
Bowel ischemia | Stent graft migration or embolization |
Lower extremity ischemia | Stent graft erosion into the esophagus |
Rupture | Stent graft infection |
Paraplegia | Expansion or rupture of the aneurysm or dissection |
Stroke | |
Endoleak | Lower limb or bowel ischemia |
Myocardial infarction | |
Vascular access site complications (hematoma, pseudoaneurysm, infection, distal ischemia) |
Modified from Gowda RM, Misra D, Tranbaugh RF et al: Endovascular stent grafting of descending thoracic aortic aneurysms. Chest 124:714-719, 2000.
Bowel or hepatic ischemia may occur due to the stent’s occlusion of the arterial blood supply or an embolus involving the celiac trunk or superior mesenteric artery. The gradual onset of severe abdominal pain in the absence of signs of peritonism indicates the need for urgent abdominal imaging by CT or MR imaging scan to exclude mesenteric vascular insufficiency. Once signs of peritonism, acidosis, and shock have developed, bowel infarction is likely to have already developed.
Spinal cord ischemia is rare but can occur. In one small series, 3 of 53 patients undergoing endovascular stent grafting of the descending thoracic aorta developed spinal cord ischemia and 2 of them were irreversibly paralyzed.24 The onset of neurologic signs commonly occurs hours or days after the procedure.24,25 Previous or concomitant abdominal aortic aneurysm repair and the use of long or multiple stent grafts that extend into the T8 through L2 region increase the risk for paraplegia.15,26 In patients at high risk for paraplegia, cerebrospinal (CSF) fluid drainage (see subsequent material) should be considered prior to the procedure or as a rescue maneuver if symptoms develop postoperatively.24,26,27
A complication that is specific to endovascular stent grafting is endoleak, in which there is persisting flow into the aneurysm or the false lumen of a dissection. Endoleaks may occur at the proximal or distal attachment site (type I), in a retrograde manner via side branches (type II), or through defects in the graft itself (type III). Leaks at the junction of two sequential grafts can also occur. An endoleak may be diagnosed at the time of stent deployment or during the early or late postoperative period. Endoleak may cause pain due to expansion of the aneurysm or dissection, but in many cases symptoms are minimal and the problem is identified on CT scanning at the time of stent deployment or at routine follow-up (Fig. 11-9). If an endoleak is identified it may be left and followed up with serial CT scans or a repair may be attempted. For type I endoleak, molding the stents by using a balloon catheter or deploying additional stents may be undertaken; for a type II endoleak, angiographic coiling of feeder vessels may be performed. Aneurysm expansion is an indication to intervene for an endoleak.
AORTIC SYNDROMES: OPEN SURGICAL REPAIR
Ascending Aorta and Aortic Arch
For aortic syndromes involving the ascending aorta and aortic arch, open repair is typically performed through a midline sternotomy with the use of cardiopulmonary bypass (CPB). Modifications to the CPB technique described in Chapter 9 may be required. In the presence of aneurysm or dissection, it may not be possible to position the arterial bypass cannula in the proximal ascending aorta, in which case the distal ascending aorta, right axillary artery, or femoral artery is used.
For a type A dissection it is necessary to replace only that part of the aorta that contains the entry tears, not the entire length of the dissection. If an aneurysm or the entry tear of a type A dissection extends into the aortic arch, it is not possible to place a cross-clamp above the pathology without compromising blood supply to the brain. In this situation, surgical repair of the aortic arch is performed using hypothermic circulatory arrest. On CPB, the patient is cooled to a state of deep hypothermia (<20°C), the heart-lung machine is turned off, and the patient is exsanguinated into the venous reservoir. Circulatory arrest provides a bloodless surgical field that is not obstructed by clamps and cannulas. The main danger in hypothermic circulatory arrest is neurologic injury, the incidence of which depends on many factors, but primarily on the duration of circulatory arrest and the patient’s age.28,29 Although there is no absolutely safe period of circulatory arrest, the incidence of neurologic injury increases substantially beyond 30 minutes at a temperature of 20°C.28 In an attempt to reduce the incidence of neurologic injury, techniques of selective cerebral perfusion have been developed, in which cold, oxygenated blood is perfused (usually) antegrade via the carotid arteries during the period of circulatory arrest. One technique for antegrade cerebral perfusion, which relies on an intact circle of Willis, involves placing the arterial bypass cannula in the right axillary artery. During circulatory arrest, blood is perfused down the arterial cannula at low flow rates (10 ml/kg/min) with the innominate artery clamped, thus directing flow up the right common carotid artery. Excellent neurologic outcome has been achieved with this technique.30 If selective cerebral perfusion is used, some surgeons limit systemic cooling to 20° to 25°C.
Descending Thoracic Aorta
A number of surgical strategies are used. The most simple is the “clamp-and-sew” technique, in which the proximal thoracic aorta is cross-clamped and the distal aorta repaired (Fig. 11-10A). Some surgeons have achieved good results with this approach.31 However, cross-clamping the descending thoracic aorta in the absence of CPB or distal aortic perfusion is associated with a number of adverse hemodynamic and metabolic effects. Proximal to the clamp (the heart and the head) there is marked hypertension, increased left ventricular afterload, and the potential for myocardial ischemia. Distal to the clamp (the abdominal organs and spinal cord) there is end-organ hypoperfusion with the potential for paraplegia, mesenteric ischemia, and renal failure. Unclamping may be associated with profound hypotension and acidosis. Outcomes of the clamp-and-sew technique are critically dependent on the aortic cross-clamp time.
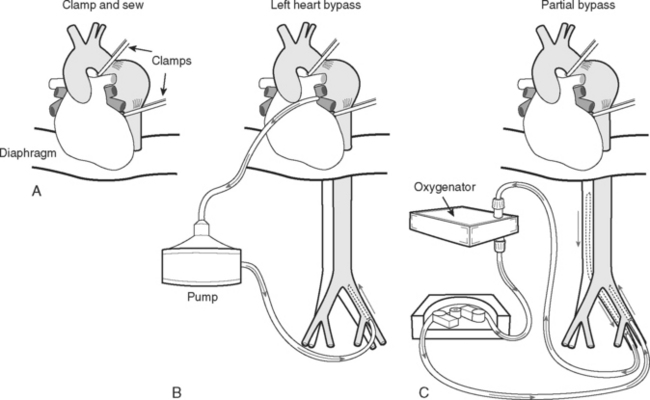
Figure 11.10 Repair techniques for aneurysms involving the descending thoracic aorta. See text for details.
Three techniques that are used to decompress the proximal aorta and maintain perfusion to the distal aorta during aortic cross clamping are (1) passive aortoaortic shunting across the clamped area using a Gott shunt; (2) left heart (atriofemoral) bypass; and (3) partial (femoral-femoral) bypass. With left heart bypass (see Fig. 11-10B), oxygenated blood is drained from the left atrium and is returned to the femoral artery. A simple heparin bonded circuit consisting of a heat exchanger and a constrained vortex pump is typically used. Full heparinization is not required. With partial bypass (see Fig. 11-10C), deoxygenated blood is drained from the femoral vein, passes through a heat exchanger and a membrane oxygenator, and is returned to the femoral artery. Both techniques require ventilation and normal cardiac function throughout the period of bypass support. The use of a heat exchanger in the circuits allows the use of modest hypothermia (30° to 34°C), potentially providing some neurologic protection. Distal aortic perfusion with left heart or partial bypass stabilizes hemodynamics, minimizes acidosis, and maintains perfusion to the abdominal organs but has not unequivocally been shown to reduce the incidence of paraplegia. Techniques that involve aortic cross-clamping are often combined with lumbar cerebrospinal fluid (CSF) drainage (see subsequent material). CSF drainage reduces CSF pressure and therefore helps to maintain spinal cord perfusion pressure during aortic cross-clamping. Two recent metaanalyses have demonstrated a reduction in adverse neurologic events with CSF drainage compared to without drainage.32,33
An alternative strategy for the repair of aneurysms of the descending thoracic and thoracoabdominal aorta is the use of full CPB and hypothermic circulatory arrest. CPB is initiated with bypass cannulas placed in the femoral artery and vein, and the patient is cooled to 15° to 18°C. The proximal aortic anastomosis is completed under circulatory arrest and a second arterial cannula is placed proximally in the graft. The aorta is then repaired by means of sequential repositioning of two aortic cross-clamps, while maintaining perfusion to the upper and lower body via the two arterial cannulas. This technique is associated with an acceptable mortality rate and a low incidence of paralysis, stroke, and visceral end-organ dysfunction, particularly renal failure.34
Postoperative Care Following Open Aortic Repair
Postoperative complications following open surgical repair of thoracic pathology are determined by the site of the surgery, the nature and extent of the operation, and the patient’s comorbid state. The mortality rate in patients undergoing surgery on the thoracic aorta is about 10%35 but increases to more than 30% in emergency surgery.36
Cardiovascular Function
Both high and low cardiac-output states are seen following aortic surgery. High cardiac output may occur due to the development of the systemic inflammatory response syndrome. Low cardiac output may occur for several reasons. Postoperative myocardial stunning is common as the result of the effects of prolonged hypothermic CPB and difficulties with myocardial protection (see Chapter 9). Patients with thoracic aortic disease often have left ventricular hypertrophy and aortic regurgitation, both of which can create problems with myocardial protection. Rarely, myocardial ischemia can arise due to anatomic problems related to reimplantation of the coronary arteries following aortic root replacement (see Chapter 10). Right ventricular dysfunction may occur due to gas emboli in the anteriorly placed right coronary artery (usually with transient effects). Pericardial tamponade is not uncommon following aortic root surgery, particularly when there is marked coagulopathy.
Because of the high incidence and multiple causes of hemodynamic instability in this group of patients, routine use of a pulmonary artery catheter and early use of echocardiography is appropriate. An intraaortic balloon pump is contraindicated after surgery on the descending thoracic aorta.
Respiratory Function
Respiratory failure is common following thoracoabdominal aortic surgery and is associated with a high mortality rate.6 Severe contusion of the left lung is common following procedures on the descending thoracic aorta because of the effects of surgical handling, pulmonary collapse during one-lung ventilation, and heparinization. Postoperatively the chest radiograph may demonstrate a white-out appearance that persists for some days. Lung compliance and gas exchange are often impaired and patients may require prolonged mechanical ventilation. During the initial postoperative period there may be considerable bleeding into the tracheobronchial tree from the left lung. If, at the end of surgery, endobronchial bleeding is a significant problem, the double-lumen tube may be left in place for 24 hours to protect the right lung from being soiled by blood.
Bleeding
Aortic surgery involves extensive suture lines that are exposed to systemic arterial pressure. Surgery is typically prolonged and commonly involves the use of hypothermia and circulatory arrest. Thus, patients are at high risk for both surgical and nonsurgical bleeding during the early postoperative period. Thrombocytopenia, coagulopathy, and fibrinolysis may require aggressive treatment. Blood component therapy should be guided by coagulation tests and thromboelastography (see Fig. 17-1). Warming a patient also helps to control bleeding, but hyperthermia should be avoided (see subsequent material).
Renal Failure
Postoperative renal impairment is common. In one large series of patients undergoing thoracoabdominal aortic operations, renal impairment (serum creatinine >3 mg/dl or 0.26 mmol/l) occurred in 18% of patients, with dialysis being required in 9% of cases.37
Brain Injury
Permanent brain injury (focal or global deficit) occurs in up to 10% of patients following thoracic aortic surgery that involves hypothermic circulatory arrest;29 increased age and the duration of circulatory arrest are the two main risk factors. The presence of extensive aortic atheroma, even of the descending aorta, is associated with an increased risk for embolic stroke.
Hyperthermia exacerbates neurologic injury and should be avoided in all patients after cardiac surgery but particularly in patients at high risk for brain or spinal cord injury. Following arrival in the ICU, patients should be rewarmed slowly and incompletely (to 36°C). For patients at very high risk for brain injury (e.g., following a prolonged period of circulatory arrest), 12 to 24 hours of deliberate postoperative hypothermia (<34°C) may be considered. The risk for neurologic injury must be weighed against the increased risk for bleeding associated with hypothermia. Neurologic outcome may also be improved by control of the blood glucose level (see Chapter 36).
Spinal Cord Ischemia
The incidence of lower-limb neurologic deficits following surgery on descending thoracic aortic aneurysms (Crawford type I or II) is as high as 30%.32 Some of these deficits are minor and the patient might recover, but the risk for permanent paralysis is a very real concern. Paraplegia or paraparesis typically presents as an anterior spinal artery syndrome, including loss of motor function and pinprick sensation but preservation of vibration and position sense.
As noted earlier, lumbar CSF drainage appears to reduce the risk for paralysis and may also reverse any new-onset paralysis that develops during the postoperative period.38 CSF drains are normally inserted at the L3/4 or L4/5 disk spaces. Some anesthesiologists prefer to insert the catheters the night before surgery to allow time for any epidural bleeding to settle. CSF drainage should continue for at least 24 hours following surgery, aiming to keep the CSF pressure less than 10 to 12 mmHg. If there are no signs of paralysis, the catheter can be capped at 24 hours and the patient observed for a further 24 hours before the catheter is removed. If there are signs of lower-limb weakness, the CSF should be drained to a lower target pressure in an attempt to reverse the weakness. If there is evidence of paralysis but CSF drainage has not been performed, the insertion of a catheter and drainage of CSF should be considered. Spinal cord perfusion pressure is also dependent on systemic arterial blood pressure; therefore hypotension should be avoided. If possible, patients should be awakened early so their neurologic status can be assessed.
The incidence of serious side effects with lumbar CSF drainage is low—less than 1%.32,39 The most important side effects are meningitis and hematoma (subdural or epidural). While the catheter is in situ, daily samples of the CSF should be sent for microbiologic culture. If meningitis is suspected, the catheter should be removed and antibiotic therapy started. Staphylococcus aureus is the most likely infective pathogen. It is prudent to avoid removing the catheter while the patient is coagulopathic or if the CSF is heavily blood stained.
Analgesia
Extensive left thoracotomy for descending thoracic or thoracoabdominal aortic pathology is associated with severe pain that can contribute to postoperative respiratory insufficiency and myocardial ischemia. Excellent analgesia can be obtained with a thoracic epidural analgesia, but this technique can potentially mask the signs of paraplegia. If epidural analgesia is used, the catheter should be inserted prior to surgery while the patient is awake and hemostasis is normal. Provision of analgesia by means of an epidural opioid (see Chapter 12) instead of a local anesthetic facilitates neurologic assessment. Alternatives to epidural analgesia include a paravertebral block and intravenous opioids. Once the patient is awake, intravenous opioids may be administered by a patient-controlled analgesia system.
1 Bauer M, Pasic M, Meyer R, et al. Morphometric analysis of aortic media in patients with bicuspid and tricuspid aortic valve. Ann Thorac Surg. 2002;74:58-62.
2 Elefteriades JA. Natural history of thoracic aortic aneurysms: indications for surgery, and surgical versus nonsurgical risks. Ann Thorac Surg. 2002;74:S1877-S1880.
3 Cambria RA, Gloviczki P, Stanson AW, et al. Outcome and expansion rate of 57 thoracoabdominal aortic aneurysms managed nonoperatively. Am J Surg. 1995;170:213-217.
4 White KC, Kavanaugh JF, Wang DM, et al. Hemodynamics and wall shear rate in the abdominal aorta of dogs: effects of vasoactive agents. Circ Res. 1994;75:637-649.
5 Gibbons RJ, Balady GJ, Bricker JT, et al. ACC/AHA 2002 guideline update for exercise testing: summary article: a report of the American College of Cardiology/American Heart Association Task Force on Practice Guidelines (Committee to Update the 1997 Exercise Testing Guidelines). Circulation. 2002;106:1883-1892.
6 Money SR, Rice K, Crockett D, et al. Risk of respiratory failure after repair of thoracoabdominal aortic aneurysms. Am J Surg. 1994;168:152-155.
7 Eggebrecht H, Baumgart D, Schmermund A, et al. Penetrating atherosclerotic ulcer of the aorta: treatment by endovascular stent-graft placement. Curr Opin Cardiol. 2003;18:431-435.
8 Nienaber CA, Richartz BM, Rehders T, et al. Aortic intramural haematoma: natural history and predictive factors for complications. Heart. 2004;90:372-374.
9 Daily PO, Trueblood HW, Stinson EB, et al. Management of acute aortic dissections. Ann Thorac Surg. 1970;10:237-247.
10 Nienaber CA, von Kodolitsch Y, Nicolas V, et al. The diagnosis of thoracic aortic dissection by noninvasive imaging procedures. N Engl J Med. 1993;328:1-9.
11 Moore AG, Eagle KA, Bruckman D, et al. Choice of computed tomography, transesophageal echocardiography, magnetic resonance imaging, and aortography in acute aortic dissection: International Registry of Acute Aortic Dissection (IRAD). Am J Cardiol. 2002;89:1235-1238.
12 Hagan PG, Nienaber CA, Isselbacher EM, et al. The International Registry of Acute Aortic Dissection (IRAD): new insights into an old disease. JAMA. 2002;283:897-903.
13 Januzzi JL, Sabatine MS, Eagle KA, et al. Iatrogenic aortic dissection. Am J Cardiol. 2002;89:623-626.
14 Parodi JC, Palmaz JC, Barone HD. Transfemoral intraluminal graft implantation for abdominal aortic aneurysms. Ann Vasc Surg. 1991;5:491-499.
15 Bortone AS, de Cillis E, D’Agostino D, et al. Endovascular treatment of thoracic aortic disease: four years of experience. Circulation. 2004;110:II262-II267.
16 Destrieux-Garnier L, Haulon S, Willoteaux S, et al. Midterm results of endoluminal stent grafting of the thoracic aorta. Vascular. 2004;12:179-185.
17 Mitchell RS, Dake MD, Sembra CP, et al. Endovascular stent-graft repair of thoracic aortic aneurysms. J Thorac Cardiovasc Surg. 1996;111:1054-1062.
18 Nienaber CA, Fattori R, Lund G, et al. Nonsurgical reconstruction of thoracic aortic dissection by stent-graft placement. N Engl J Med. 1999;340:1539-1545.
19 Dake MD, Kato N, Mitchell RS, et al. Endovascular stent-graft placement for the treatment of acute aortic dissection. N Engl J Med. 1999;340:1546-1552.
20 Ramaiah V, Rodriguez-Lopez J, Diethrich EB. Endografting of the thoracic aorta. J Card Surg. 2003;18:444-454.
21 Neuhauser B, Czermak B, Jaschke W, et al. Stent-graft repair for acute traumatic thoracic aortic rupture. Am Surg. 2004;70:1039-1044.
22 Nienaber CA, Eagle KA. Aortic dissection: new frontiers in diagnosis and management: Part II: therapeutic management and follow-up. Circulation. 2003;108:772-778.
23 Brandt M, Hussel K, Walluscheck KP, et al. Stent-graft repair versus open surgery for the descending aorta: a case-control study. J Endovasc Ther. 2004;11:535-538.
24 Gravereaux EC, Faries PL, Burks JA, et al. Risk of spinal cord ischemia after endograft repair of thoracic aortic aneurysms. J Vasc Surg. 2001;34:997-1003.
25 Bhama JK, Lin PH, Voloyiannis T, et al. Delayed neurologic deficit after endovascular abdominal aortic aneurysm repair. J Vasc Surg. 2003;37:690-692.
26 Gowda RM, Misra D, Tranbaugh RF, et al. Endovascular stent grafting of descending thoracic aortic aneurysms. Chest. 2003;124:714-719.
27 Tiesenhausen K, Amann W, Koch G, et al. Cerebrospinal fluid drainage to reverse paraplegia after endovascular thoracic aortic aneurysm repair. J Endovasc Ther. 2000;7:132-135.
28 Svensson LG, Crawford ES, Hess KR, et al. Deep hypothermia with circulatory arrest: determinants of stroke and early mortality in 656 patients. J Thorac Cardiovasc Surg. 1993;106:19-28.
29 Ergin MA, Galla JD, Lansman L, et al. Hypothermic circulatory arrest in operations on the thoracic aorta: determinants of operative mortality and neurologic outcome. J Thorac Cardiovasc Surg. 1994;107:788-797.
30 Di Eusanio M, Schepens MA, Morshuis WJ, et al. Brain protection using antegrade selective cerebral perfusion: a multicenter study. Ann Thorac Surg. 2003;76:1181-1188.
31 Crawford ES, Crawford JL, Safi HJ, et al. Thoracoabdominal aortic aneurysms: preoperative and intraoperative factors determining immediate and long-term results of operations in 605 patients. J Vasc Surg. 1986;3:389-404.
32 Cina CS, Abouzahr L, Arena GO, et al. Cerebrospinal fluid drainage to prevent paraplegia during thoracic and thoracoabdominal aortic aneurysm surgery: a systematic review and meta-analysis. J Vasc Surg. 2004;40:36-44.
33 Khan SN, Stansby G. Cerebrospinal fluid drainage for thoracic and thoracoabdominal aortic aneurysm surgery. Cochrane Database Syst Rev. 2004:CD 003635.
34 Kouchoukos NT, Masetti P, Rokkas CK, et al. Hypothermic cardiopulmonary bypass and circulatory arrest for operations on the descending thoracic and thoracoabdominal aorta. Ann Thorac Surg. 2002;74:S1885-S1887.
35 Barmettler H, Immer FF, Berdat PA, et al. Risk-stratification in thoracic aortic surgery: should the EuroSCORE be modified ? Eur J Cardiothorac Surg. 2004;25:691-694.
36 Svensson LG. Natural history of aneurysms of the descending and thoracoabdominal aorta. J Card Surg. 1997;12:279-284.
37 Svensson LG, Crawford ES, Hess KR, et al. Experience with 1509 patients undergoing thoracoabdominal aortic operations. J Vasc Surg. 1993;17:357-368.
38 Khong B, Yang H, Doobay B, Skala R. Reversal of paraparesis after thoracic aneurysm repair by cerebrospinal fluid drainage. Can J Anaesthes. 2000;47:925-992.
39 Cheung AT, Pochettino A, Guvakov DV, et al. Safety of lumbar drains in thoracic aortic operations performed with extracorporeal circulation. Ann Thorac Surg. 2003;76:1190-1196.