Thermoregulation
Thermoregulation is the balance between heat production and heat loss involved in maintaining thermal equilibrium. Heat is produced by the body as a by-product of metabolic processes and muscular activity; thus a major function of the thermoregulatory system is dissipation of this heat.18 The thermoregulatory system must also respond appropriately to alterations in environmental temperature to preserve thermal equilibrium. Maintenance of thermal stability is particularly critical in the newborn in that exposure to cold environments and lowered body temperatures are closely correlated with survival, especially in very low–birth weight (VLBW) infants. Maternal temperature changes are also important in relation to fetal well-being and the potential adverse consequences of maternal hyperthermia. Regulation of body temperature is summarized in Figure 20-1 and Box 20-1 on page 658.
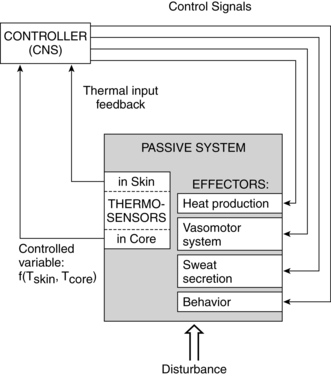
Maternal physiologic adaptations
Antepartum period
The amount of heat generated increases 30% to 35% during pregnancy because of the thermogenic effects of progesterone, alterations in maternal metabolism and basal metabolic rate (see Chapter 16), and maternal dissipation of heat generated by the fetus.30,91 As a result, many pregnant women develop an increased tolerance for cooler weather and decreased tolerance for heat. The additional heat is dissipated by peripheral vasodilation with a fourfold to sevenfold increase in cutaneous blood flow and increased activity of the sweat glands (see Chapter 14). Cutaneous vasodilation leads to skin warmth.
The maternal temperature usually increases by 0.5° C (0.3° F). Both core and skin temperature increase during pregnancy, with a slight decrease reported in late pregnancy. The core temperature peaks by midpregnancy.85 The decrease in core temperature in late pregnancy may be related to decreases in progesterone and physical activity (which generates heat) during this time. The rise in skin temperature is particularly evident in the hands and feet, probably due to arteriovenous shunting in these areas.129 In general, heat accumulation may be slower and heat dissipation faster in later pregnancy than before pregnancy or in early pregnancy. The increased plasma volume during pregnancy provides a greater area for heat storage and may enhance heat transfer from the fetus to the mother.85 The pregnant woman has decreased vasoconstriction in response to cold during pregnancy. This may alter her ability to conserve heat during cold stress.85
Body temperature increases with exercise due to heat generated by increased metabolic energy production. Some of this heat is dissipated by increased skin blood flow; the remainder is stored transiently, increasing the core temperature. Changes in temperature with exercise during pregnancy are moderate, as compared with changes in nonpregnant women, suggesting that the enhanced thermoregulatory capacity of the pregnant woman may help protect her against hyperthermia.85 The increased plasma volume in pregnancy may assist in maintaining uterine and placental blood flow during exercise and in maximizing heat transfer from the fetus and heat dissipation in the mother. Aerobic exercise in water is associated with minimal changes in either core or skin temperature.85,129 Exercise during pregnancy is discussed further in Chapters 9 and 10.
Intrapartum period
An increase in body temperature, averaging 1° C (1.7° F), may occur during labor as a result of physical activity with uterine contractions and the release of substances from the fetal-placental unit that may stimulate the maternal hypothalamic thermoregulatory center.87,91,104 However, Bartholomew and colleagues reported that 95% of 147 women had temperatures between 36.2° C and 37.8° C (97.2° F and 100.0° F) during labor.11 Diurnal variations were noted with a nadir at midday and peak in the evening.11
In some women the increase in temperature during labor is high enough to generate concern that the mother is infected. Although this may sometimes be the case, the most common cause for maternal fever during labor is use of epidural analgesia, not infection.1,11,134 Women with epidural analgesia are more likely to develop a fever during labor than women without this form of anesthesia.50,97,104,134 Epidural anesthesia in nonpregnant individuals is more likely to lead to a decreased rather than increased temperature. The fever seen with epidurals is usually characterized by a slow increase in temperature of approximately 0.07° C/hour, although the temperature may rise to greater than 37.5° C (99.5° F) or 38° C (100.4° F) in some women.50,104 The basis for the increased temperature in pregnant women during the intrapartum period may be due to decreased heat dissipation (due to a decreased sweating threshold, alterations in ventilation, and altered hypothalamic responses) and an increase in heat production, possibly due to increased shivering.1,5,104,134,136 The autonomic block with epidural analgesia inhibits peripheral vasoconstriction and sweating in the lower body. The impairment of sweating and behavioral responses may decrease heat loss.11,91 Maternal fever with epidural anesthesia is associated with an inflammatory state with increased cytokines, especially interleukin-6, but this state is rarely due to infection.5,97,104,136 Prophylactic administration of acetaminophen has not been found to suppress the fever associated with epidurals.69,104 Panzer and colleagues reported that women during labor do not show the same relationship between temperature, sweating, and shivering as nonpregnant individuals.91 In the parturient, shivering was not necessarily triggered by decreased temperature nor was sweating necessarily triggered by increased temperature; the woman might shiver and sweat simultaneously. Temperature increases can lead to an increased maternal heart rate, cardiac output, oxygen consumption, and catecholamine production.104
The laboring woman is also at risk for hypothermia during the intrapartum period due to vasodilation (limiting usual vasoconstrictive responses to cold); administration of anesthetics, narcotics, and other pharmacologic agents; blood loss, rapid fluid replacement, especially if cool fluids are used; or other events that increase maternal heat loss (e.g., cold drafts, wet drapes or towels). Prolonged exposure to a cool environment can aggravate heat loss. Hypothermia can result in shivering, hypotension, and hemodynamic and cardiorespiratory instability.91
Postpartum period
Maternal temperature is monitored closely during the postpartum period because elevations may indicate infection or dehydration. A transient postpartum chill or shivering is often experienced about 15 minutes after birth of the infant or delivery of the placenta. The cause of this chill is unknown and various causes have been proposed. This phenomenon may represent muscular exhaustion or result from disequilibrium between the internal and external thermal gradients secondary to muscular exertion during labor and delivery, sudden changes in intraabdominal pressure with emptying of the uterus, or small amniotic fluid emboli. Most women who experience early postpartum shivering are normothermic, suggesting that this phenomenon is nonthermoregulatory in origin.91 Shivering is seen in about 20% of women who did not receive neuraxial analgesia and more frequently in women after epidural analgesia.91
Transient maternal temperature elevations up to 38° C (100.4° F) occur in up to 6.5% of vaginally delivering women during the first 24 hours after delivery. In most women this resolves spontaneously and is secondary to noninfectious causes such as dehydration or to a transient bacterial endometritis.42 Maternal fever in the postpartum period may be a sign of puerperal infection, mastitis, endometritis, or urinary tract infection, although these infections are usually the cause of fever after 24 hours. Any temperature elevation merits close monitoring, especially with increasingly early discharge.3,30
Clinical implications for the pregnant woman and her fetus
The fetal ambient environmental temperature is the maternal temperature. Fetal temperature is linked to maternal temperature and the maternal-fetal thermal gradient (see section on Development of Thermoregulation in the Fetus), so that if maternal temperature rises, so will the fetal temperature. Maternal oral temperature has been reported to correlate with intrauterine temperature, with maternal oral temperature lower than intrauterine temperature by 0.8° C (1.3° F) (95% confidence interval, 0.7° C, 1° C [1.28° F, 1.7° F]).10 In this study a maternal “oral temperature greater than 37.2° C (99.0° F) detected an intrauterine temperature greater than 38° C (100.4° F) with a sensitivity of 81% and a specificity of 96%.”10
Maternal hyperthermia and fever
Maternal fever has three potential detrimental effects on the fetus: (1) hypoxia secondary to maternal and fetal tachycardia and altered hemodynamics; (2) teratogenesis; and (3) preterm labor from the fever per se, from underlying infection, or from associated hemodynamic alterations.30 Maternal intrapartum fever due to infection has been linked to a risk of cerebral palsy, cognitive defects, and neonatal seizures and encephalopathy, as well as later disorders such as schizophrenia and autism.58,80,95,104 Maternal hyperthermia increases maternal oxygen consumption and shifts the oxygen-hemoglobin dissociation curve to the right. Although this latter change increases the oxygen supply to the placenta, fetal oxygen uptake becomes more difficult because of the altered thermal gradient.57
Research regarding adverse fetal effects has focused primarily on maternal temperature elevations due to fever secondary to illness, exercise, and the use of saunas or hot tubs. Animal studies show specific effects of maternal hyperthermia, especially when the maternal core temperature increases to 2° C or more above baseline; the longer the temperature elevation is maintained or the higher the temperature, the greater the risk to the fetus.24 Some of the human studies have been retrospective and suggestive but inconclusive, while other have demonstrated a clear association.7,23,24,39,85,89,120 A meta-analysis of studies, including both retrospective case-controlled and prospective cohort studies, reported an overall odds ratio of neural tube defects (NTDs) when associated with maternal hyperthermia of 1.92 (95% confidence interval, 1.16, 2.29).89 Definitions of hyperthermia in the various studies ranged from temperature greater than 37.8° C (greater than 100.4° F) to temperature greater than 38.9° C (greater than 102.0° F). Most of the hyperthermia was due to maternal influenza or other febrile illness. Others have reported a twofold to fourfold increase in the risk for NTD with maternal first trimester hyperthermia for any reason.120
Maternal febrile illness is thought to pose the greatest hyperthermic risk; however, susceptibility may be modified by genotype.20 Elevated maternal temperature secondary to illness-induced fever during early pregnancy has been associated with increased risk of anencephaly and spina bifida (especially around the time of neural tube closure (22 to 28 days), microcephaly, and other central nervous system disorders; alterations in growth; cleft lip; and facial dysmorphogenesis in humans.10,23,51 In a prospective study, Chambers and colleagues reported a 10-fold increase in NTDs in women who had a temperature of 38.9° C (102° F) or greater lasting for more than 24 hours in the first month of pregnancy.23 Whether these disorders are primarily due to the elevated temperature, the underlying infection, or a combination of these events has been debated. Most human and animal studies suggest that the increased risk is correlated to the maternal fever and not the viral illness per se.39,89 Several investigators have reported increased cleft lip and palate, NTDs, and cardiovascular disorders in women with influenza accompanied by high temperature in the first trimester with a reduction in risk with treatment of the fever with antipyretics.16,120 Reductions in risk have also been reported with folic acid supplementation.16 Antifever medications and folic acid may reduce the risk of vascular disruption and apoptosis leading to congenital anomalies.16,39
Several studies have reported an increase in neural tube defects and oral clefts in infants born to women with an extended heat exposure secondary to sauna or hot tub use in the first trimester, suggesting that an elevated temperature may be the critical factor.23,24,120 Milunsky and colleagues found that the hot tub exposure, especially in the first two months of pregnancy (and the risk increased with number of exposures during this time), posed a greater risk than sauna use, with no risk from electric blanket use.88 Prospective studies from Finland of sauna use in pregnancy have not shown an increased risk. However, in these studies, maximal temperature was 38.1° C (100.6° F), below the value of 38.9° C (102° F) thought to be critical.85
Maternal exercise and temperature elevations
Maternal exercise is associated with increased heat production and body temperature increases that may alter the maternal-fetal thermal gradient and fetal heat dissipation. Uterine blood flow decreases during exercise, further altering the ability of the mother to dissipate fetal heat.57,118 However, most women seem to be able to tolerate moderate intensity exercise without significant changes in core temperature.118 Low-impact aerobic exercise (to 70% of maximal heart rate) has not been associated with hyperthermia.72,78 The ability of the mother to dissipate the heat generated by exercise may improve as pregnancy progresses.85,118 The risks from temperature changes during exercise in late pregnancy are related to decreased uterine blood flow, which can be potentiated by dehydration. Prolonged exercise or exercise in heat (increased ambient heat reduces the thermal gradient between the skin and environment) or high humidity (decreases evaporative heat loss) may result in a higher maternal temperature than exercise in a cool, dry environment or water environment (e.g., aerobic exercise in water).85,93,129 Physical conditioning before pregnancy can improve thermoregulatory capacity and may decrease the effect of heat stress.85,93
Summary
Alterations in thermal status during pregnancy increase the risk of alterations in fetal health and development. Ongoing assessment and monitoring of thermal status in the pregnant woman and neonate and initiation of appropriate interventions to maintain thermal stability can prevent or minimize these risks. Clinical recommendations related to maternal thermoregulation are summarized in Table 20-1.
Table 20-1
Clinical Recommendations Related to Clinical Practice in Pregnant Women
Counsel pregnant women regarding basis for heat intolerance during pregnancy and intervention strategies (p. 657).
Counsel pregnant women to avoid activities that may lead to hyperthermia (pp. 659-660).
Encourage adequate fluid intake before and during exercise (p. 660).
Discourage prolonged exercise especially in a hot, humid environment (p. 660).
Maintain adequate ambient temperature in the delivery area (p. 658).
Protect from cold drafts during delivery (p. 658).
Avoid infusing cold solutions (p. 658).
Avoid contact of maternal skin with wet drapes and towels (p. 658).
Monitor maternal temperature and assess thermoregulatory status during the intrapartum and postpartum periods (pp. 657-659).
Evaluate women with elevated temperatures for signs of infection (pp. 657-659).
Development of thermoregulation in the fetus
Because fetal temperature is linked to maternal temperature and the maternal-fetal thermal gradient, the fetus cannot control its temperature independently. Under normal resting conditions, the temperature of the fetus is approximately 0.5° C (0.9° F) higher (range, 0.3° C to 1° C [0.5° F to 1.7° F]) than that of the mother, or about 37.6° C to 37.8° C (99.7° F to 100.0° F).13,14,47,77,87,94,100 About half of this difference is due to placental and uterine heat production.94 In animal studies, this difference between maternal and fetal temperatures is seen by the time the fetus achieves one third of its body weight, which would be around the beginning of the third trimester in the human fetus.87 The fetus produces about 5 calories (20.9 J) of heat per every millimeter of oxygen used.94 Fetal metabolic rate varies to match oxygen availability (adaptive hypometabolism), so that if PO2 decreases, fetal oxygen consumption also decreases and if fetal PO2 increases, so does fetal oxygen consumption.94 Any increase in fetal oxygen consumption increases heat production.118
Fetal core and skin temperature and the temperature of amniotic fluid are all similar. The fetal temperature must be higher than the maternal temperature to maintain a gradient to offload heat from the fetus to the mother. Fetal heat dissipation is influenced by fetal and placental metabolic activity, thermal diffusion capacity of heat exchange sites within the placenta, and rates of blood flow in the umbilical cord, placenta, and intervillous spaces.77,85
Heat generated by fetal metabolism is dissipated by the amniotic fluid to the uterine wall (conductive pathway) or via umbilical cord and placenta to maternal blood in the intervillous spaces (convective pathway). The majority of heat is transferred via the convective pathway through the placenta via the umbilical circulation, with only 10% to 20% dissipated via amniotic fluid.47 The large placental surface area, thin membrane barrier, and high blood flow rate enhance thermal exchange. Transfer of heat is facilitated by the maternal-fetal temperature gradient. Therefore if the mother has an elevated temperature (from exercise, illness, or exposure to hot environments such as a sauna), this gradient may be reduced or reversed, leading to an increase in the fetal temperature (see section on Maternal Hyperthermia and Fever).25 Changes in fetal temperature lag behind maternal changes because amniotic fluid provides some insulation.57
Fetal heat production and loss mechanisms are suppressed in utero. Fetal temperature is “heat-clamped” to the maternal system, preventing the fetus from independent thermoregulation before birth.94 Fetal responses to cooling are minimal and primarily involve shivering-like muscle contractions and endocrine response. Cooling of the fetus does not activate nonshivering thermogenesis (NST). The inability to initiate NST is thought to be linked to placental inhibitors of an uncoupling protein essential for brown adipose tissue (BAT) metabolism that are present in fetal blood. Both BAT and the uncoupling protein (UCP-1) that regulates its metabolism increase from 25 to 26 weeks’ gestation to term (see Brown Adipose Tissue Metabolism).32,56 The placental inhibitors, primarily prostaglandin E2 and adenosine, are an advantage to the fetus in promoting accumulation of BAT. The rapid decrease in these inhibitors with clamping of the umbilical cord at birth promotes BAT metabolism in the newborn to maintain thermal stability with transition to extrauterine life.94 Fetal cord occlusion thus leads to an increase in body temperature, although brain temperature tends to remain constant.32,56,94
The fetal-maternal temperature gradient is sustained during labor, although it may widen with prolonged labor, during the latent phase or with infection or prolonged rupture of the membranes, or decrease with compression of the umbilical cord (decreasing blood flow from the fetus and thus the ability of the fetus to dissipate heat).69,94,100 If the maternal temperature rises in labor, as often occurs with epidural analgesia (see Intrapartum Period), the fetal temperature will also increase.100,104 In the second and third trimesters, increased fetal temperature leads to increased fetal heart rate but not to other thermoregulatory responses. Maternal fever in labor, especially temperature greater than 38° C (100.4° F), in noninfected women has been associated with lower Apgar scores, hypotonia, hypoxia, and an increased need for resuscitation and oxygen at birth.10,77
Neonatal physiology
Thermoregulation is a critical physiologic function in the neonate that is closely linked to the infant’s survival and health status.62 An understanding of transitional events and neonatal physiologic adaptations is essential for provision of an appropriate environment to maintain thermal stability. Heat losses are greater and more rapid and can easily exceed heat production in both term and preterm neonates if the infants are left unclothed in an environment comfortable for an adult. This is because of the infant’s larger surface area–to–body mass ratio, decreased insulating subcutaneous fat, increased skin permeability to water, and small radius of curvature of exchange surfaces.33 Newborns (even most preterm infants) have a relatively well-developed thermoregulatory capacity. Their major thermoregulatory limitation is a narrow control range that makes them more vulnerable to alterations in the thermal environment.62
Transitional events
With birth the fetus moves from the warm, moist intrauterine environment to the colder, drier extrauterine environment. The infant’s temperature falls after birth, triggering cold-induced metabolic responses and heat production.14,18,103 Because fetal thermoregulation is linked to the mother, thermoregulatory processes are suppressed in the fetus. These processes, especially initiation of nonshivering thermogenesis (NST), must be activated rapidly after birth if the infant is to survive the transition to extrauterine life. Stimulation of cutaneous cold receptors and spinal cord and hypothalamic thermal receptors at birth activates the sympathetic nervous system. This leads to the release of norepinephrine and a twofold to threefold increase in metabolic rate, oxygen consumption, and heat production.47,94 Occlusion of the umbilical cord, which removes placental factors that suppress NST, increases a brown adipose tissue (BAT)-specific uncoupling protein with a rapid increase in BAT metabolism (see Brown Adipose Tissue Metabolism) and heat production.94,99 Thermal transition at birth and BAT metabolism are interrelated with changes in thyroid function (see Chapter 19).
Newborns lose heat rapidly after birth, especially through evaporative losses (0.58 kcal/mL [2.4 J/mL] of water loss) from their moist body surface, as well as via convection to cooler room air and radiation to cooler room walls. A newborn’s temperature may fall 0.2° C to 1° C/min (0.5° F to 1.7° F/min) if thermal interventions are not initiated.25,47,62 Rutter estimated that in a cool room, the body temperature of a 1000-g preterm infant falls 1° C (1.7° F) every 5 minutes.100 Initial temperatures of infants born by cesarean birth are on average 0.3° C (0.5° F) lower than infants born vaginally, perhaps due to slightly lower levels of sympathetic activity, catecholamines, and thyroid activity.94 Interventions in the delivery room to reduce evaporative and other losses support transition, reduce cold stress, and have been associated with a higher PO2 at 1 hour of age, lower mortality, and decreased morbidity.62 Since much of the heat loss immediately after birth is due to evaporative losses, infants should be quickly dried and wrapped in a warm, dry towel and either given to the mother for skin-to-skin contact or placed under a radiant warmer or in a prewarmed incubator.47,48 A polyethylene bag or wrap can be used with VLBW infants to reduce heat loss after birth (see Methods of Promoting Thermal Stability).14,29,64 Heat loss in VLBW infants immediately after birth can also be reduced by maintaining adequate ambient temperatures and humidity in the delivery and stabilization room.122 Bissinger and Annibale recommend 50% humidity and room temperatures of 78° F to 80° F (25.5° C to 26.6° C) for infants less than or equal to 28 weeks’ gestation (less than or equal to 1000 g) and greater than or equal to 72° F (22.2° C) with a goal of 75° F (23.8° C) for infants 29 to 32 weeks’ gestation (1001-1500 g).14 Other interventions to reduce evaporative, radiant, conductive, and convective losses are listed in Table 20-2.
Table 20-2
Prevention of Heat Loss and Overheating in the Neonate
MECHANISM | SOURCES OF HEAT LOSS OR OVERHEATING | INTERVENTIONS |
Conduction | Cool mattress, blanket, scale, table, x-ray plate, or clothing |
Maintain the room temperature at levels adequate to provide a safe thermal environment for infants according to their gestational ages.
Transport the infant in an enclosed, warmed incubator through internal hallways and between external environments (e.g., ambulance to nursery).
Open incubator portholes only when necessary and for brief periods.
Swaddle with warm blankets (unless under radiant warmer).
Use polyethylene bags or wraps with VLBW infants from birth; use transparent plastic across the infant between the radiant warmer side guards; use caps with adequate insulation quality or hooded blankets.
Dry the infant, especially the head, immediately after birth with a warm blanket or towel.
Use caps with adequate insulation quality or hooded blankets.
Use polyethylene bags or wraps with VLBW infants from birth.
Replace wet blankets with dry, warm ones and place in a warm environment.
Delay the initial bath until the infant’s temperature has stabilized; then give a sponge bath.
Bathe the infant in a warm, draft-free environment, place on warmed towels and dry immediately; bathe under a radiant warmer.
Term newborns can increase their metabolic rate by up to 200% to 300%.18,47 This response is delayed in larger preterm infants, who do not approximate term values until 2 to 3 weeks of age; further delay is seen in VLBW infants.18,35 VLBW infants may only have a maximal increase in metabolic activity of 25% with cold stress.25 In extremely low–birth weight (ELBW) infants, an increase in thermal maturational skills has been reported at 28 weeks’ postmenstrual age, possibly due to decreased evaporative losses.35
Most healthy infants increase their temperatures by 2 to 3 hours after birth. Thermal stabilization may be affected by timing of the first bath, thermal status at time of bathing, and method of bathing.19,86,131 VLBW infants are more likely than term infants to have admission temperatures of less than 36° C (96.8° F).14,69
Heat exchange
Heat exchange occurs between the environment and the infant’s skin and respiratory tract. Heat transfer involves four mechanisms: evaporation, radiation, conduction, and convection. All four mechanisms operate at the body surface; in the respiratory tract, evaporation and convection are the main mechanisms of heat exchange.103 The degree of heat exchange depends on these mechanisms as well as other factors such as body surface area, position, body movements, and body shape.103 Equations are available to calculate heat exchange at the infant’s body surface via evaporation, radiation, conduction, and convection as well as heat exchange between the respiratory tract and the environment.103
Heat transfer
Heat transfer through the internal gradient is increased in neonates because of their thinner layer of subcutaneous fat (i.e., less insulation) and a larger surface area–to–body mass ratio, especially in preterm infants. The subcutaneous layer of insulating fat accounts for only 16% of body fat in infants compared with 30% to 35% in adults.34 The body mass of the neonate is about 5% of adult mass, whereas the surface area is 15%. In term infants, the surface area–to–body mass ratio may be three times, and in preterm infants five times, greater than that of adults.101 This ratio is even higher in ELBW infants. For example, the surface area–to–body mass ratio of a 500-g infant may be six times greater than that of an adult and twice as great as that of a 1500-g infant.55
The external gradient involves transfer of heat from the body surface to the environment. The rate of heat loss is directly proportional to the magnitude of the difference between skin temperature and the environmental temperature and can be expressed as follows: heat loss = h (skin temperature − environmental temperature) × (surface area), where h is the thermal transfer coefficient (the rate at which heat leaves the body surface) and is influenced by body size, tissue conductance, skin blood flow, and vasoactivity.18,103 Heat loss per unit of body mass is inversely proportional to body size.18 As noted above, the mechanisms by which heat is transferred from the body surface are conduction, convection, radiation, and evaporation (see Prevention of Excessive Heat Loss or Heat Gain).
Heat transfer by the external gradient is also increased in the neonate because of increased surface area and an increased thermal transfer coefficient.103 In terms of heat loss, the amount of exposed surface area is most critical; thus an infant who is not in an incubator or radiant warmer will lose less heat if he or she is clothed or swaddled. Factors that increase the thermal transfer coefficient (and thus heat loss), such as decreased skin thickness and altered conductance, are present in the neonate. The threshold for heat production in the newborn is more closely linked to skin temperature than in the adult. As a result, cold responses, especially in preterm infants, are related primarily to skin rather than core temperature changes.18
Heat production and conservation
Heat production is a result of metabolic processes that generate energy by oxidative metabolism of glucose, fats, and proteins. The amount of metabolic heat produced varies with activity, feeding (calorigenic or specific dynamic action), state (greater heat is produced in awake infants than in sleeping infants and in active versus quiet (deep) sleep), health status, and environmental temperature.18,26,35 Organs that generate the greatest amount of metabolic energy are the brain, heart, and liver. To maintain a constant body temperature, heat production must equal heat loss from the body surface over a given time. Basal heat production to maintain this stability is generated by body metabolic processes. In the event of cold stress, heat above basal needs can be generated by physical or chemical mechanisms. Because of their surface area–to–body mass ratio (which is an important determinant of heat loss), heat production in infants is low relative to heat loss.100 In the term infant, heat production at rest (estimated by oxygen consumption) in a thermoneutral environment is similar to that of adults per unit of weight, but approximately half that of the adult per unit of surface area. This is further decreased in preterm infants, who have an even greater surface area–to–body mass ratio and are less likely to spontaneously lie in a flexed position.100 Prone positioning in low–birth weight (LBW) infants has been reported to increase central and peripheral body temperature in spite of the lower metabolic rates seen in this position.4 In term infants the maximal heat production increases from 20% to 75% of adult values over the first postbirth week; this change takes longer in preterm infants.103
Physical mechanisms to generate include involuntary (shivering) and voluntary muscular activity. Shivering is the most important mechanism for the generation of additional heat in adults. The neonate uses physical methods (shivering and increased muscular activity) to some extent to generate additional heat. Shivering, which is controlled via the somatomotor system, is not as important in the neonate as in the adult, and the shivering threshold is probably at a lower body temperature.101 Shivering in neonates is primarily seen as a late event associated with decreased spinal cord temperature after prolonged cold exposure. The cervical spinal region is protected from cold stress and preferentially receives heat generated by NST through metabolism of BAT in the intrascapular area. If NST is blocked or the infant is unable to generate adequate heat to compensate for severe or prolonged cold stress, the temperature of the spinal cord eventually decreases.18
Infants, primarily term or late preterm infants, produce some heat by increasing muscular activity with restlessness, hyperactivity, or crying. This increases heat production in skeletal muscles with breakdown of glycogen and glucose oxidation.13,25 Infants may try to conserve heat by postural changes such as flexion that reduce the surface area and heat loss through the internal gradient. The ability to produce heat by physical methods can be markedly reduced or obliterated with the use of anesthetics, muscle relaxants, sedatives, or tight restraints and in infants with brain injury.18,34,47,103
Heat can be generated by chemical mechanisms or NST through changes in the metabolic rate and, primarily in neonates, by BAT metabolism. These changes are mediated by the sympathetic nervous system. Both infants and adults can generate heat by increasing their metabolic rate above basal levels. An adult can increase heat production by 10% to 15% by NST; in the neonate, this increase can be 100% or more.53 NST is mediated by epinephrine in the adult and by norepinephrine in the neonate.21,119 This results in activation of an adipose tissue lipase and splitting of triglycerides into glycerol and nonesterified fatty acids (NEFA), which are oxidized to produce heat, esterified to form triglycerides, or released into the circulation.21,115,119 NST is triggered when the mean skin temperature falls to 35° C to 36° C (95° F to 96.8° F).115
NST is the major mechanism through which the infant produces heat above basal needs. Increasing the metabolic rate may lead to further problems in immature or compromised neonates, because any increase in metabolic rate increases oxygen consumption. Stressed infants may be unable to provide enough oxygen; oxygen debt with lactic acidosis from anaerobic metabolism and finally exhaustion can result. The ability to generate heat by NST is limited in ELBW infants.65
Thermal receptors in the skin are important mediators of the hypothalamic thermal center’s response to temperature changes or cold stress. Stimulation of these receptors initially leads to heat-conserving responses with peripheral vasoconstriction.64 This may result in acrocyanosis in the neonate. In the infant, thermal receptors are most prominent and sensitive over the trigeminal area of the face. For example, cooling the face of an infant who is normothermic causes a rise in metabolic rate. Conversely, warming the facial skin (i.e., use of warmed oxygen in an oxygen hood) when the infant’s body is cold may suppress the usual increase in metabolic rate and other heat-generating mechanisms and can be dangerous.103 A recent study of ELBW found that these infants did not demonstrate peripheral vasoconstriction the first 12 hours after birth even with a low body temperature.65 Other studies have found minimal or no peripheral vasoconstriction with poor vasomotor control in infants weighing less than 1000 g, increasing their risk of thermoregulatory problems.65
Brown adipose tissue metabolism
The neonate relies primarily on BAT metabolism for NST. Large amounts of BAT are found in human and animal newborns, hibernators, and in adult animals after cold acclimatization.21,34,90 Small amounts of BAT remain in human adults, although it is found primarily in the cervical-supraclavicular area and often interspersed within white adipose tissue.21,32 Active BAT is seen more frequently in adult females than adult males.133
The major function of BAT is heat production. In the newborn, BAT is found in the midscapular region; nape of the neck; around the neck muscles extending under the clavicles into the axillae; in the mediastinum; and around the trachea, esophagus, heart, lungs, liver, and intercostal and mammary arteries; abdominal aorta; kidneys; and adrenal glands. The largest deposits of BAT are around the kidneys and adrenals, with smaller amounts around the great vessels, extending to the neck and from the thoracic cavity to the axillae and clavicles.34 In children and adults the BAT is less widely dispersed as in the newborn and has a lower lipid content.32 The total amount of heat produced by BAT metabolism in the neonate is unknown, but it may account for nearly 100% of the infant’s needs.
BAT cells begin to differentiate by 25 to 26 weeks’ gestation and immature brown adipocytes are seen by at least 29 weeks.32 BAT increases in the third trimester. BAT stores continue to increase in the early weeks after birth and can double during this time.32 The term newborn has an estimated 30 g of BAT accounting for approximately 1% of the infant’s weight and accounting for one tenth of the adipose tissue in these infants.32,119 BAT stores are lower in preterm and minimal in VLBW infants.
In appearance and composition, BAT in the infant is markedly different from white adipose tissue (Table 20-3).18,21,34,90,119 These characteristics promote rapid metabolism, heat production, and heat transfer to the peripheral circulation. The lipolysis rate in BAT is three times higher than in white adipose tissue.21 The unique structure of BAT gives it the ability to generate more energy than others tissue in the body.
Table 20-3
Characteristics of Brown Adipose Tissue and Their Significance
CHARACTERISTIC | SIGNIFICANCE |
Many small fat vacuoles | Large fat-to-cytoplasm ratio, enhancing the rapidity of fat use |
Many mitochondria | Production of energy (i.e., adenosine triphosphate [ATP]) for rapid metabolic turnover and heat production |
Glycogen stores | Source of glucose for production of ATP and energy |
Abundant blood supply | Brings nutrients to cell and transports heat produced to other areas of the body |
Abundant sympathetic nerve supply | Metabolism of brown adipose tissue mediated by norepinephrine |
Heat production within the BAT cell is regulated by uncoupling protein-1 (UCP-1), sometimes called thermogenin, located along the inner membrane of brown adipocyte mitochondria (Figure 20-2). UCP-1 permits protons to pass across the mitochondrial membrane without the need to conserve energy. UCP-1 uncouples oxidative phosphorylation and adenosine triphosphate (ATP) synthesis via oxidation of fatty acids. As a result, more energy is used for heat and less is conserved for ATP regeneration.21,28,82,90,101,113,115 UCP-1 increases from 29.4 ± 3 pmol/mg at 25 weeks’ gestation to 62.5 ± 10.2 pmol/mg at 40 weeks with a marked increase seen at 32 weeks’ gestation that marks the ability to use NST.56,64 This gestational age is also the time when levels of enzymes to convert T4 to T3 also increase.56,64 Thus NST is immature in infants of less than 32 weeks’ gestation, and especially in ELBW infants, due to low levels of BAT, decreased UCP-1, and decreased thyroid hormones.64
UCP-1 is also expressed in the smooth muscle of the uterus, digestive tract, and male reproductive tract, although at much lower levels than in BAT, and may have a role in smooth muscle relaxation.28 There has been increased interest in the roles of UCP-1 and BAT in recent years since BAT is thought to play a role in energy balance.28,32,82,96 BAT in adults is associated with low total adipose tissue content and may have a role in weight control. UCP-1 is involved in diet-induced thermogenesis and body weight regulation.28 Thus there has been increased interest and research recently in BAT related to the prevention and treatment of obesity and age-related disorders.32,76,82,96,133
Activation of UCP-1 is controlled by the hypothalamus. The sympathetic nervous system and hormonal mediators control BAT metabolism. Changes in temperature are transmitted from peripheral cutaneous receptors to the posterior hypothalamus (see Figure 20-1). The sympathetic nervous system is stimulated to release norepinephrine within BAT stores and to stimulate catecholamine release from the adrenal medulla.74,94,112
Norepinephrine released at the surface of brown adipocytes interacts with α1-, β1-, and, primarily, β3-adrenergic receptors to increase cyclic adenosine monophosphate (cAMP). The cAMP increases lipase activity, allowing rapid hydrolysis of triglycerides and phospholipids within the brown adipocytes. The free fatty acids released activate UCP-1, which further increases heat production.94 This process is enhanced by triiodothyronine (T3) and thyroxine (T4), which up-regulate UCP-1, as well as by cortisol and prolactin.82,94,113 A thyroid hormone surge occurs with birth (see Chapter 19). The thyroid gland is stimulated by the pituitary release of thyroid-stimulating hormone to produce T4, which is then converted to T3. Thyroid hormones enhance the effect of norepinephrine on the BAT cells and may also act directly on UCP-1.18,34,94,112,113 Leptin may also play a role in neonatal thermoregulation. Leptin (see Chapter 16) is primarily found in white adipose tissue, but small amounts are seen in BAT, increasing from 35 weeks’ gestation to term.21,112 Leptin is regulated by the sympathetic nervous system, cortisol, and thyroid hormones. Before birth, placental prostaglandin E2 and adenosine block the catecholamine-induced increase in cAMP that is needed to initiate NST. Occlusion of the umbilical cord with cord clamping removes this inhibition. Recent studies have found that signaling molecules of the fibroblast growth factor and bone morphogenetic protein families are also involved in BAT adipogenesis.32,82
Heat dissipation and loss
The neonate can dissipate heat by peripheral vasodilation or by sweating. As the infant’s core temperature rises above 37.3° C (99.1° F), cutaneous blood flow and skin thermal conductance increase along with heat loss through the external gradient.121
Sweating increases evaporative loss. Each milliliter of water evaporated results in 0.58 cal (2.4 J) of heat loss.103 Term infants can increase evaporative losses up to 100% but are less effective at sweating than adults. Although the density of sweat glands is six times greater in the neonate, the capacity of these glands is only about one third of adult values.45 Sweat appears first on the infant’s forehead, generally beginning after 35 to 40 minutes of exposure to an ambient temperature above 37° C (98.6° F). By 70 to 75 minutes, evaporative water losses increase four times. In the term small-for-gestational-age (SGA) infant, the onset of sweating is slower (55 to 60 minutes), but evaporative losses increase more rapidly.
Sweating is also altered in infants with central nervous system dysfunction and in preterm infants. Infants of mothers on opiates such as heroin and methadone may have early maturation of sweating.100 In preterm newborns over 30 weeks’ gestation, the onset of sweating is delayed. SGA infants demonstrate thermoregulatory potential similar to that in infants of comparable gestation but are limited by their body size. In preterm infants the maximal rate of sweating is less than that of either term or SGA infants. Sweating is minimal or nonexistent in infants of less than 30 weeks’ gestation because of nonfunctional or immature sweat glands.45,103 However these infants have significant transepidermal water and heat losses due to their immature skin (see Chapter 14).115,137 These losses are significantly increased in infants cared for in a radiant warmer or receiving phototherapy (see Chapter 18). Neonates also lose heat by radiation, conduction, and convection (discussed in the next section).
Clinical implications for neonatal care
Prevention of cold stress and hypothermia is critical for the intact survival of the neonate. Lowered body temperatures are inversely correlated with survival, especially in VLBW infants.30,45,62,69,103 Exposure to cool environments and subsequent cold stress often result in physiologic changes that significantly alter the infant’s health status, especially in VLBW and ELBW infants. Introduction of the intensive care environment into the delivery room may improve outcomes for these infants.132 Major components of neonatal care include maintenance of infant thermoregulatory processes, provision of an appropriate thermal environment, and prevention of heat loss, hypothermia, and cold stress.
Neutral thermal environment
Body temperature and oxygen consumption are closely related. As the body temperature falls, the amount of oxygen needed for survival increases rapidly. Oxygen consumption is minimal in two thermal regions: the neutral thermal environment (NTE) and with severe hypothermia (Figure 20-3). The NTE (thermoneutrality) is an idealized setting defined as a range of ambient temperatures within which the body temperature is normal, metabolic rate is minimal, and thermoregulation is achieved by basal nonevaporative physical processes alone.18,25,33 Within the thermal neutral range, the person is in thermal equilibrium with the environment (Figure 20-4). Because all newborns lose fluid through their skin continuously, evaporative loss is always present. Darnell proposes the following clinical definition of thermoneutrality for infants: “that environment (usually a range of air temperatures in an incubator or abdominal skin temperature under a radiant warmer) in which the infant, when quiet or asleep, is not required to increase heat production above ‘resting’ levels to maintain body temperature.”33 Figure 20-3 illustrates the relationships between thermoneutrality, body temperature, and metabolism.
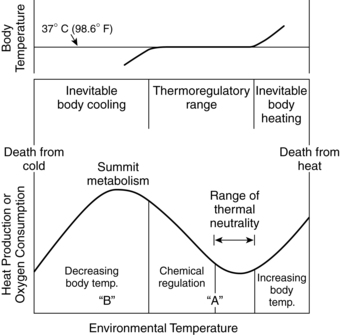
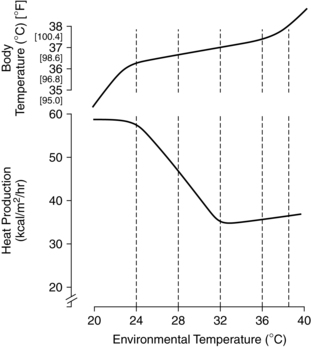
In the thermoneutral state an infant is neither gaining nor losing heat, oxygen consumption is minimal, and the core-to-skin temperature gradient is small. A normal skin or core temperature does not necessarily mean that the infant is in a thermal neutral zone, in that an infant may be maintaining that temperature by increasing metabolic rate and BAT metabolism. Thus body temperature is not a very sensitive indicator of neutral thermal environment stability.13,25,100 Swaddling or clothing widens the lower range.100
Thermoneutrality is generally achieved at environmental temperatures of 25° C to 30° C (77° F to 86° F) in the adult; at 32° C to 33.5° C (89.6° F to 92.3° F) in the unclothed term infant and at 24° C to 27° C (75.2° F to 80.6° F) when clothed; at 34° C to 35° C (93.2° F to 95° F) in the unclothed preterm infant at 30 weeks (1500 g) and at 28° C to 34° C (82.4° F to 93.2° F) when clothed; and at higher temperatures in more immature infants.18,100,119 Environmental conditions that do not tax an adult may require increased metabolic work by the neonate. The thermoneutral range is narrowest for infants of the lowest birth weights.100 Guidelines for determining neutral thermal zones for infants of varying ages and weights are available.62,103 These guidelines were developed for healthy infants at set environmental conditions and must be used in conjunction with evaluation of the temperature of the incubator walls and external environment; humidity; air velocity; posture; clothing; infant weight; health status and activity level; and, especially in the first week, gestational age.100,103 These guidelines are not appropriate for ELBW infants because these infants require higher neutral temperatures because of increased evaporative losses. The most immature infants may require environmental temperatures equal to or higher than skin and core temperatures.103
Preterm infants cared for in environments outside the thermoneutral zone may not grow as well as infants with similar caloric intakes cared for in a thermoneutral environment. VLBW infants may respond to temperatures outside the thermoneutral zone by a change in body temperature unaccompanied by an increase in oxygen consumption. The very high transepidermal water losses in these infants seem to be the major factor in determining their appropriate thermal environment. Use of a neutral thermal environment in the early weeks after birth is associated with smaller feeding and caloric requirements.62,115
Prevention of excessive heat loss or heat gain
The four mechanisms by which heat is transferred to and from the body surface are conduction, convection, radiation, and evaporation. Examples of each mechanism in the neonate and appropriate interventions are listed in Table 20-2.
Conduction
Conduction involves transfer of heat from the body core to the surface through body tissue and from the body surface to objects in contact with the body (mattress, clothing, and scales). The rate at which heat is transferred is directly proportional to the size of the temperature gradient.47 Conductive loss can be minimized through insulation such as blankets or clothing or through skin-to-skin contact and is increased by placing the infant on conductive surfaces such as metal.48 The mattress used in most incubators and radiant warmers has a low conductivity. Room temperature blankets and mattresses can be associated with significant heat loss, especially in the preterm infant, and must be warmed before use.14,100
Convection
With convective losses, heat is dissipated from the interior of the body to the skin surface through the blood, conducted from the body surface to surrounding air (boundary layer), and carried away by diffusion to moving air particles (drafts, colder room or outside air) at the skin surface. Natural (free) or passive convection involves movement of heat molecules into the boundary layer near the skin and then gradually away from the infant.14,47 Forced convection occurs when a mass of air is physically moving over the infant, conveying heat away from the body.14,25,47,73 Transfer is dependent on ambient temperature, air flow velocity (usually at velocities of greater than or equal to 0.27 m/sec), and relative humidity (which determines the air’s thermal density).47 In LBW infants, convective losses are increased by the shorter radius of the curvature of the body surface.121 Heat can be lost by convective means through transfer of warmed inspired air to colder exterior air (or unheated oxygen) through exhalation. During resuscitation, use of cool oxygen (which removes warm carbon dioxide from the lungs) can decrease body temperature.62 Measurements of air temperature should be made close to the infant’s skin surface, because air temperature within an incubator may vary.
Convective losses are increased at higher air-flow velocities.73,103 This is known as the wind-chill factor and may be induced by incubator air circulation. Environments with higher air temperatures and minimal air circulation can reduce convective loss by up to two thirds.119 Heat can be gained by convection if ambient temperature is higher than the infant’s skin temperature. Incubators use this principle to warm infants by circulating warm air around the infant.
Convective heat losses can be minimized by swaddling or using caps on infants in cribs, use of polyethylene bags or wraps with VLBW infants after birth (see Methods of Promoting Thermal Stability), warming oxygen, and placing infants away from drafts or air vents (see Table 20-2).14,29,64 Warming of oxygen from a face mask is important because of the sensitivity of the thermal receptors in the trigeminal area. If the infant’s environment is well heated below the neck but cool around the face, the infant will react as if cold stressed. This can lead to metabolic alterations and hyperthermia.103 If the infant’s face is warmer than the body, apnea may result.
Evaporation
Evaporative heat loss occurs as moisture on the body surface or respiratory tract mucosa vaporizes. These losses depend on air speed and relative humidity. Evaporative loss is the major source of heat loss immediately after delivery, in the first few weeks, and during bathing, accounting for up to 25% of the total heat loss. A wet newborn in the delivery room loses heat and lowers his or her skin temperature at a rate of 0.3° C/min (0.5° F/min) and rectal temperature by 0.1° C/min (0.2° F/min). This change is equivalent to a temperature loss of 3° C (5° F) over 10 minutes.115
Evaporative losses are correlated with both gestational age and postbirth age.103 For example, when cared for in an ambient humidity of 50%, evaporative heat exchange is up to 5 W/m2 in term infants versus 50 W/m2 in preterm infants.103 Transepidermal water loss (TEWL) is up to six times higher per unit of surface area in VLBW infants than in term infants and up to 15 times higher in infants at 25 weeks’ gestation.100,101 TEWL is lower in small-for-gestational-age than appropriate-for-gestational-age infants at similar gestations.103 In immature infants, higher evaporative loss due to poor skin resistance to water passage from a lack of skin keratinization within the stratum corneum (see Chapter 14) constitutes a significant portion of overall heat loss (Figure 20-5).14,44,47 The stratum corneum normally provides the greatest resistance to diffusion of water from the skin. In the term newborn the keratin in the stratum corneum creates resistance to water diffusion that is 1000 times greater than the resistance of the dermis.81 In VLBW infants, evaporative losses in the first days exceed all other sources of heat loss and often exceed heat production.47,73,115 Preterm infants lose heat via evaporation at a rate of 0.58 kcal/mL (2.4 J/mL).47 VLBW infants may lose up to 120 mL/kg/day through skin water loss. This represents a loss of up to 72 kcal/kg/day, a significant portion of the infant’s total caloric intake.47,62 Evaporative losses in preterm infants of 26 weeks’ gestation or less may be greater than 180 to 200 mL/kg/day.14,47,103 Evaporative losses decrease with increasing post-birth age.
Evaporative losses in VLBW infants can be reduced by altering the environment. The degree of TEWL depends on the humidity, so infants in environments with greater humidity will lose less water and fewer calories. For example, TEWL at 85% to 95% humidity is approximately 10% that at 50% humidity.81,103 Evaporative insensible water loss (IWL) increases with activity and tachypnea, under radiant warmers, or with phototherapy (see Chapter 18).55 Rapid maturation of the skin occurs in the first few weeks after birth in infants of all gestational ages, reducing the degree of TEWL (see Chapter 14). Even at 4 weeks of age, TEWL is still twice as high in preterm versus term infants.101 The term and older preterm infant can increase evaporative losses via the skin by sweating in response to a warm environment.100
Evaporative losses may be minimized by drying infants immediately after delivery or bathing, swaddling them in warm blankets, warming soaks and solutions, and warming and humidifying oxygen (see Table 20-2). Use of polyethylene wrappings, caps, and bags with preterm infants in the delivery room and intensive care nursery can also reduce evaporative losses (see Methods of Promoting Thermal Stability).22,83,92,103,127,135 Use of topical petroleum emollients to reduce evaporative losses has been associated with an increased risk of bacterial infection and is not recommended.40,103 Evaporative water losses in infants under radiant warmers can be reduced by stretching a layer of plastic wrap or bubble wrap around the infant or across the infant between the side guards of the radiant warmer, which can reduce IWL up to 75%.47,100
In term and preterm infants, the evaporative heat loss is inversely proportional to the partial pressure of water vapor.63,73 The neutral thermal environment temperature has been calculated to be reduced approximately 0.5° C (0.8° F) for each 1 mm of increase in water vapor pressure.115 Increasing relative humidity reduces evaporative losses; at a relative humidity of 100%, evaporative loss is nonexistent.121 Incubator humidity levels may need to be increased to reduce evaporative losses in VLBW infants. These infants may have subnormal temperatures despite incubator temperatures above their body temperatures. The elevated air temperature reduces convective and radiant losses but does little to reduce the extremely high transepidermal evaporative loss in these infants. Vapor pressure increases with higher temperatures, so as long as the infant’s skin is warmer than the environment, evaporative losses can occur even with 100% humidity.103
Radiation
A major form of heat loss in infants in incubators is radiation. Radiation involves the transfer of radiant energy from the body surface (through absorbance and emission of infrared rays) to surrounding cooler or warmer surfaces (walls, windows, heat lamps, lightbulbs) not in contact with the infant. The rate of transfer depends on the temperature gradient, surface absorption, and geometry (the amount and angle of the infant’s surface area facing the object).47,73,103 Radiant heat losses are independent of ambient temperature, air speed, and other heat loss mechanisms.119 The infant can also gain heat by radiation, which is the principle of radiant warmers in which the (cooler) infant is placed under the warm radiant heat source.
Regulating incubator temperature only does not prevent heat loss by radiation. Thus infants can get cold in a room containing air warmer than the infant if the walls and windows are cold. Infants in warm incubators can be cold stressed if they radiate body heat to cooler incubator walls or cooler windows and walls or to the cool outside air during transport. The amount of radiant loss is related to the temperatures of the window and walls rather than the temperature of the air in the incubator. Conversely, a baby in a cool incubator can get overheated if the incubator walls or room windows or walls are too hot.47,62,103 Heat loss by radiation is reduced in double wall versus single wall incubators. Loss of heat by radiation is also influenced by the infant’s position and amount of exposed radiating surface area.25
Incubators tend to act as greenhouses by trapping heat. The acrylic walls of the incubator are opaque to infrared rays. The walls allow short light waves to enter the incubator and subsequently the infant’s body. The infant converts the short waves to heat and reemits them as longer infrared rays. Because these rays cannot escape from the incubator, they heat the incubator and then the infant (“greenhouse effect”). Infants in incubators can become hyperthermic even if they are not subjected to obvious heating and without a change in incubator temperature.119 Radiant heat losses can be reduced by placing vulnerable infants away from the cooler exterior walls and windows, use of thermal shades, and use of double-walled incubators. Overheating of infants by radiation can be prevented by placing infants away from windows and walls that are warmer than the infant.47,62,103
Monitoring temperature
Neonatal temperature is usually monitored by skin or axillary measurements, and by manual or servocontrol methods. Although rectal temperature provides an approximation of core temperature, it is not used, because of the risks associated with taking rectal temperatures, including trauma, perforation, and cross-contamination with repeated insertions. Axillary temperatures are noninvasive and approximate core temperature. The optimal length of time to obtain an accurate assessment of temperature with axillary measurement using a mercury glass thermometer carefully placed in the axilla is 3 to 5 minutes in most studies.75,110
In a cold environment, core temperature may not indicate thermal stability. In a cold-stressed infant, the core temperature may be within normal limits because the infant has successfully compensated by increasing nonshivering thermogenesis. By the time the core temperature falls, the infant may be significantly compromised and difficult to rewarm.103 Skin temperature measurement is used with preterm and other neonates at risk for thermoregulatory problems. Skin temperature changes provide an early indication of cold or heat stress in that an early mechanism to preserve body heat is peripheral vasoconstriction detected by measuring skin temperature.62
Thermocouples or thermistors must be carefully placed because skin temperature can vary widely. The best area for probe placement is not known, although sites usually recommended in textbooks are the skin surface over the liver, between the umbilicus and pubis, or the back in a prone infant. Commonly suggested sites to avoid are over areas of BAT, poorly vasoreactive areas, and excoriated or bruised areas or near transcutaneous gas monitoring transducers.15 However, there have been few probe placement site studies to determine either the best areas or which areas to avoid. Differences have been reported between various sites used for probe placement, with lower temperatures in sites not exposed to radiant heat.15,75 Lying on the probe may raise temperature by increasing skin insulation and lead to variable skin temperatures with repositioning.15
Disadvantages of skin temperature include accidental displacement of the probe, risk of skin irritation, misleading values with rapid temperature changes, and artifacts altering accuracy of measurement.3,15,47 Artifacts that can alter readings include inadvertent insulation of the probe and the underlying skin; partial loss of skin contact with the probe; radiant or convective heating or cooling of the probe; increased probe temperature if covered with clothing or blankets or if the infant is lying on the probe (producing falsely high readings); and alteration in evaporative loss in skin covered with probes. This may occur with use of probe covers so that the temperature of the skin under the probe may be different from the skin temperature at other sites.3,100,102,103,124 Insulated probes provide different information from exposed probes.103,124 Insulated probes may result in probe temperatures greater than skin temperatures in either a radiant warmer or incubator and have been reported to alter incubator servocontrol with lower incubator temperatures and a higher skin-to-environment temperature gradient.100,103,124 Therefore it is important to follow the manufacturer’s directions regarding the types of probes and probe covers.
Servocontrol
Servocontrol methods are used to maintain an infant’s temperature within specified ranges. Servocontrol systems regulate both skin and air temperatures. A change in air temperature results in a change in the wall temperature and in heat exchange via convection and radiation.103 Infant skin servocontrol using proportional control units are standard in current convective incubators.47 Air and skin servocontrol produce different thermal environments (Figure 20-6).6,102,124,125 Air servocontrol tends to provide a more stable thermal environment with greater infant temperature variability, whereas skin servocontrol leads to greater variability in air temperature but is more effective in maintaining an NTE.6,116,124,125 With infant skin servocontrol, the temperature from a skin probe is electronically monitored and used to control heater output decisions in either a convectively heated incubator or radiant warmer. Considerations related to use of skin probes and probe artifacts are discussed in the preceding section. Risks of servocontrol include hyperthermia, if the probe becomes detached or is left in the manual mode, and failure to detect early signs of sepsis because alterations in body temperature are masked.
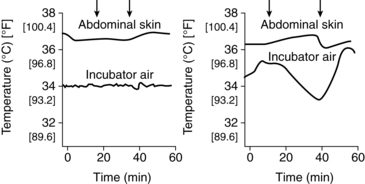
Servocontrol may be affected by high evaporative water losses. The servocontrol system is based on the assumption that the temperature of the skin under the probe represents that of the surrounding skin; however, attaching the probe to the skin reduces evaporative losses at the site where temperature is being monitored. Thus the servocontrol system may record skin temperatures that are higher than those of the rest of the infant’s body and fail to keep the infant warm.6,47,103 Increasing humidity may reduce these differences. Opening of the portholes may lead to overdamping of the servocontrol system, with undershooting or overshooting of the air temperature for up to 1 hour later.47 This problem has been reduced with the newer combination convection incubator–radiant warmer devices.
Methods of promoting thermal stability
A major consideration in monitoring thermal status and promoting thermal stability in the neonate involves issues related to the various types of equipment that are currently available. Other methods of supporting thermoregulation are summarized in Table 20-2.
High-risk neonates are generally cared for in convectively heated incubators; open, radiantly heated beds; or hybrid devices. The hybrid devices combine these two methods with an incubator mode in which plastic walls enclose the infants and a radiant warmer mode in which the radiant warmer is turned on and the plastic walls retract. Each of these pieces of equipment and methods has inherent advantages and disadvantages, as do adjuncts to these beds such as humidity and heat shields, thermal blankets, polyethylene skin wraps, and warmed mattresses.2,14,29,61,70,83,117 There is no consistent evidence supporting either closed, convectively warmed incubators or open, radiant warmers as more effective in reducing morbidity or mortality; each has its advantages and disadvantages, especially for VLBW infants.43
Convective incubators
Convective incubators operate by circulation of warmed air. Air entering the incubator is filtered, providing a barrier against airborne pathogenic organisms from the environment. Air leaving the incubator is not filtered, so nearby personnel or infants in open warmers and cribs are not protected from airborne organisms in the incubator air. In double-walled incubators, walls are warmed by a layer of warm water or by computer-controlled, electrically conductive plastic panels, thus decreasing heat loss, and reducing radiant losses and oxygen consumption.62,71
Up to 60% to 70% of total heat loss in infants cared for in convectively heated incubators is by radiation.25,121 Because Plexiglas is relatively opaque to radiant waves, infants radiate their body heat primarily to the inner side of the incubator wall. Radiant losses are reduced with double-walled incubators, which place a second layer of Plexiglas between the infant and the outer incubator wall. The infant radiates heat to the inner wall, which is surrounded on both sides by warmed incubator air. Increasing the ambient temperature of the room and placing the infant away from windows or exterior walls can also reduce radiant losses in infants in incubators. Further alterations in radiant losses occur when infants are clothed or incubators are covered with blankets or quilts to reduce light and noise levels. As a result of extremely high transepidermal evaporative losses, VLBW infants may have subnormal temperatures even though incubator temperatures are above their body temperatures and double-walled incubators are used.
A major disadvantage of convective incubators is temperature fluctuations that occur with opening and closing of the portholes or hood. When the portholes are open, the temperature of the air in the incubator falls rapidly and recovers slowly.137 Laminar flow incubators minimize temperature fluctuations with the opening of portholes. The drop in air temperature when opening the incubator is less in double-walled than single-walled incubators, providing a more stable thermal environment during caregiving. The inner wall in a double-walled incubator acts as a heat reservoir, while in some models warm air is redirected across the front of the incubator when it is opened.102 The air thermometer or thermistor in an incubator should be near the infant’s body and protected from being covered with bedding, clothing, or cool air flow from a resuscitation bag left in the incubator.25
Convective losses can also occur in incubators due to either natural convection or forced air convection. Natural convection is the thermal gradient between the skin surface and the surrounding air. Warm air rises from the infant’s skin, carrying heat and moisture. This air then cools and falls back to the infant’s surface, especially over the curvature of the body. Infants positioned in flexion have less exposed surface area and less heat loss than infants in an extended posture.25,47 Forced convection usually occurs at air velocities equal to or greater than 0.2 m/sec.103 Lower air velocities (especially less than 0.1 m/sec) minimize these losses, as can swaddling or clothing and humidity. In incubators currently in use, the primary route of convective loss is through natural convection, because the air velocities near the infant’s body are minimal.47,102
Air in the incubator is usually humidified by active methods. Active methods use a modular integral or external humidifier, so water vapor is continuously added to the circulating air via vaporization. This method generally uses servocontrol, and the amount of humidity can be individualized. Advantages of active humidification include reduction in infection risk, easier cleaning, and reduced recovery time after incubator doors are opened.81
Radiant warmers
Radiant warmers maintain the infant’s temperature using a proportional servocontrol system that maintains skin temperature at a constant level. The optimal skin temperature is not known and may vary from infant to infant.102 Radiant heat is produced in the infrared spectrum and penetrates below the skin surface, where epidermal cells with a high water content absorb the radiant energy and convert it to heat. This heat is transferred to deeper tissues by conduction and circulating blood. The heat source is usually about 80 to 90 cm from the infant’s surface.47,100
Radiant warmers are convenient to use, allow direct access to the infant, decrease radiant losses, and eliminate temperature fluctuations with opening of the portholes. Evaporative and convective—and possibly radiant—losses may increase, however, with a risk of dehydration.13,43,103 Evaporative losses are primarily increased due to the lower humidity and can be reduced by use of plastic wrap or blankets. IWL in infants in radiant warmers is increased 50% to 200%.53 These losses may counteract the reduction in radiant losses in VLBW infants. Further IWL occurs if infants are simultaneously being treated with phototherapy (see Chapter 18). Although it may be possible to compensate for variations in evaporative and convective heat losses, the increased evaporative water losses are more difficult to manage.47 Radiant warmers have been associated with an increase in oxygen consumption and metabolic rate, although the increase has not been statistically significant in most trials.100,115 Other issues in use of radiant warmers include those associated with servocontrol and probe use, discussed earlier.
Convective losses can be reduced by using the sides on the warming bed or by stretching a layer of plastic wrap across the infant between the radiant warmer’s side guards or on a special frame. Plastic wrap does not block radiant heat waves as does Plexiglas; thus heat shields made from this material are not recommended for use with radiant warmers.47,115 Plastic wrap also reduces IWL; plastic shields do not have a similar effect.47
Skin-to-skin care
At birth, term infants who are dried and covered with a warm blanket and placed skin to skin against their mother or who experience skin-to-skin contact later maintain their temperature as well as infants cared for in standard heating units.13,46,48 Studies of skin-to-skin (kangaroo) care with stable preterm infants demonstrate that most infants maintain adequate thermal control during this type of holding, especially if the infant is wearing a hat and is covered with a blanket. In some infants, both skin and rectal temperature transiently increase during kangaroo care.12,17,27,74,102,115 Somewhat less thermal stability has been reported in infants less than 26 weeks’ gestation.17 Others have found no significant differences in skin temperature before, during, and after skin-to-skin care in clinically stable ELBW infants if transfer techniques were standardized to prevent heat loss.79,130 During kangaroo care, the parent’s clothing around the sides and back of the infant forms a “pouch” that provides insulation and reduces non evaporative losses (and possibly evaporative losses).115 ELBW infants in particular require careful temperature monitoring during kangaroo care to avoid both heat stress and cooling.
Use of head coverings, clothing, blankets, and polyethylene wraps
Clothing and head coverings can provide thermal insulation, reducing radiant and convective losses, and can, depending on the fabric, also reduce evaporative loss.70,102 In the neonate the head accounts for one fifth of the total body surface area. Brain heat production is estimated to account for 55% of total metabolic heat production in the newborn.98 Thus the neonate’s head is poorly insulated and accounts for a significant proportion of total heat loss. Head coverings are often used to reduce heat loss from this area, and may be especially useful immediately after birth and after a bath when the infant’s hair is wet.103 Head coverings or caps made of stockinette, which is a poor insulator, are relatively ineffective in reducing heat loss.98 More effective materials include caps made of insulated fabrics, wool, or polyolefin; lined with Gamgee; or insulated with a plastic liner.98 Head coverings have been found to decrease heat loss after delivery and in neonates cared for in cribs and incubators.98,123 These coverings are most effective in a cool environment and are less effective in a thermoneutral environment or with use of infant servocontrol.102 Head coverings and clothing can interfere with radiant heat loss and gain and are not appropriate for infants under radiant warmers. There has been little research on the use of hats with VLBW infants.
Clothing and blankets alter the thermal environment by both widening and lowering the neutral thermal range.100 Insulation from swaddling with blankets decreases evaporative loss by reducing the amount of exposed surface area and by changing airflow patterns and skin emissivity. In addition, swaddling and nesting maintain flexion, which reduces exposed surface area and thus convective and radiant losses.47 Swaddled infants in servocontrolled double-walled incubators have been reported to have higher abdominal skin temperatures and require lower incubator temperatures.111 Swaddling appears to have a greater effect on skin than core temperature. Others have found that nested infants have closer approximation of skin and rectal temperatures.75
Polyethylene wraps and bags are recommended for use with preterm infants to reduce heat loss after birth and in the early days postbirth.22,29,83,92,103,127 These wraps enhance radiant heat gain and reduce evaporative losses, IWL, and TEWL by creating a microenvironment under the wrap.14,29 Unwrapping the infant disrupts this microenvironment, so it should be minimized.14
Neonatal hypothermia and cold stress
Alterations in health status or maturity can compromise the ability of the newborn to efficiently and effectively regulate heat production and respond to cold stress. Infants at risk and the basis for this risk are summarized in Table 20-4.
Table 20-4
Infants at Risk for Problems in Thermoregulation
Inability to increase oxygen consumption or minute ventilation further
Inability to increase metabolic rate and reduce metabolic response to cold
Impairment of BAT metabolism by hypoxia (impaired with PO2 of 45 to 55 torr; essentially ceases at values below 30 torr)114
Inadequate caloric intake to meet metabolic demands
Increased risk of metabolic acidosis
Increased temperature losses through evaporation of water from lungs
ATP, Adenosine triphosphate; BAT, brown adipose tissue.
Compiled from references 18,34,47,62,103.
Signs of hypothermia are often absent or nonspecific in neonates. Clinical findings may include lethargy, restlessness, pallor, cool skin, tachypnea, grunting, poor feeding, or decreased weight gain.103,119 Hypothermia may be a sign of neonatal sepsis since the newborns have an altered response to pyrogens and as a result may not increase their temperature and run a fever with infection.13,103 The neonatal response to pyrogens is not well understood.13 Hypothermia in ELBW infants has been associated with abnormal heart rates (either less than 25th percentile or greater than 75th percentile of the individual infant’s baseline heart rate) with a correlation between heart rate and abdominal temperature reported.66
“Cold stress occurs when an exposed infant loses more heat than he or she produces.”64 Cold stress can interfere with interpretation of pH, because pH is temperature-dependent.100 Cold stress may delay transition from fetal to neonatal circulation.14,64 With severe cold stress the infant may develop peripheral edema and sclerema. The initial temperature decrease is peripheral with stimulation of cutaneous receptors and initiation of NST. If the heat generated by this method is insufficient, the core temperature will drop. ELBW infants are at increased risk of cold stress due to poor vasomotor control with decreased peripheral vasoconstrictive responses, lack of both white and brown adipose tissue, thin skin, increased surface area/weight, and inefficient brown adipose tissue (BAT) metabolism due to decreased BAT, UCP-1, and thyroid hormones.64
The hypothermic or cold-stressed neonate tries to compensate by conserving heat and increasing heat production. These compensatory mechanisms can lead to physiologic alterations that may set off a series of adverse metabolic events (Figure 20-7). If uninterrupted, this chain of events can result in hypoxemia, metabolic acidosis, glycogen depletion, hypoglycemia, and altered surfactant production.52 Physiologic effects of hypothermia and their consequences are summarized in Table 20-5.
Table 20-5
Consequences of Neonatal Hypothermia
PHYSIOLOGIC EFFECT | PHYSIOLOGIC CONSEQUENCE |
Peripheral vasoconstriction | |
Increased metabolic rate | |
Increased requirements for oxygen, glucose, and calories | Exhaustion of supplies with subsequent development of hypoglycemia, hypoxia, aggravation of respiratory distress syndrome, or weight loss |
Increased production of ketone bodies and accumulation of lactic acid from anaerobic metabolism | |
Norepinephrine release | |
Elevation in plasma nonesterified fatty acids | |
Dissociation of albumin and bilirubin because of acidosis |
Use of hypothermia for neuroprotection
Mild induced hypothermia has been investigated in recent years as a neuroprotective strategy to reduce secondary reperfusion injuries in infants who are at risk for post-asphyxial hypoxic-ischemic encephalopathy.8,9,36,37,38,54,59,105,106,108,109 The phases of cerebral injury (see Figure 6-4) are described in Chapters 6 and 15. Hypothermia is initiated during the latent stage to prevent or reduce the secondary phase changes. Both selective cooling of the head with mild systemic hypothermia (34° C to 35° C [93.2° F to 95° F]) and whole-body cooling have been studied in several ongoing multinational trials.8,49,54,106–108 The cooling generally begins within 6 hours after birth and continues for 48 to 72 hours. A recent meta analysis of 13 trials (n = 1440 infants) confirmed beneficial effects in terms of improved survival and outcome with no significant adverse effects up to 18 to 24 months of age, although even with cooling, mortality and morbidity remained high.106 In this analysis there was a reduction in the combined outcomes of mortality and neurodevelopmental disability and with neurodevelopmental disability in both systemic hypothermia and selective head cooling with decreases in cognitive delay, psychomotor delay, and cerebral palsy also reported in infants with systemic hypothermia.106 Long-term safety and efficacy have not been established, and the ideal cooling temperatures, method of cooling, and duration of cooling are still unclear.105
Hyperthermia in the neonate
Although discussions regarding alterations in neonatal thermoregulation often focus primarily on hypothermia, the neonate is also at risk for hyperthermia (heat stress). Hyperthermia increases metabolic demands, so even a slight increase in temperature can result in a significant increase in oxygen consumption, especially in preterm infants. Other consequences of hyperthermia include increased heart, respiratory, and metabolic rates; increased IWL; dehydration; peripheral vasodilation with a risk of decreased blood pressure; alteration in weight gain; and the risk of hypoxia and metabolic acidosis.25,62,103 Neonatal hyperthermia (i.e., temperature above 37.5° C to 37.8° C [99.5° F to 100.0° F]) is primarily due to overheating and less often to hypermetabolism. Hyperthermia also results from dehydration, drugs, or alterations in hypothalamic control mechanisms secondary to birth trauma. Maternal and neonatal fever is associated with maternal epidural anesthesia.1,47,104,134
Overheating because of increased environmental temperatures or radiant gains from exposure to sunlight or heat lamps is associated with peripheral vasodilation, a “flushed” appearance especially prominent in extremities, warm extremities, increased activity, irritability, increased IWL, extended posture, and sweating (in older preterm or term infants). Skin temperatures are higher than core temperatures. Foot temperature is no more than 2° C to 3° C (3.3° F to 5.0° F) cooler than abdominal skin temperature.100,103 Infants, particularly VLBW infants, are at risk for thermal injury from environmental sources (see Table 20-4).
The neonate is more vulnerable to overheating than are older individuals because the infant has a lower capacity for normothermic heat storage, a larger surface-to-volume ratio, and narrower control range than an adult does.62 At environmental temperatures above the neutral thermal zone, thermal equilibrium is reestablished by increasing evaporative losses.18 The adult responds by increasing skin water losses and sweating. The neonate’s initial response is to increase respiratory water loss (via tachypnea). Infants more than 30 weeks’ gestation can sweat if the environmental temperature exceeds their threshold.45
Hyperthermia secondary to hypermetabolism can result from sepsis, cardiac problems, drug withdrawal, or other metabolic alterations. Clinical signs include peripheral vasoconstriction; pale, cool extremities; and a core body temperature that is higher than skin temperature.13,103 Foot temperature is more than 3° C (5.0° F) cooler than abdominal skin temperature.100,103 Fever is less commonly seen with infections in neonates due to an immature, weak, or absent response to fever-producing substances such as bacterial endotoxins.13 Regardless of the cause, hyperthermia can lead to heat stroke, dehydration, and brain damage in the neonate.
Maturational changes during infancy and childhood
Nonshivering thermogenesis (NST) is thought to be the major mechanism for thermoregulation in infancy, especially during the first 3 to 6 months.18,32,76 With increasing age, NST becomes less prominent and the infant begins to rely more on physical methods of heat generation such as activity and shivering.18 Brown adipose tissue (BAT) is greater in children than adults and plays an important role in thermoregulation until at least 2 years.32 After that time, BAT in children and adults continues to play a role in energy balance and may have a role in weight control and prevention of obesity.32,76 After 10 years of age, BAT becomes less widely distributed as more peripherally situated deposits disappear.32
Much of the BAT is gradually replaced by white adipose tissue. This change is correlated with the switch in the major thermoregulatory mechanism from NST to shivering.119 Sweating becomes more efficient as a method of heat dissipation. The threshold body temperature for sweating decreases and maximal sweat production increases.121 Infants continue to have a decreased hypothalamic response to pyrogens for the first 2 to 3 months.84
Differences in thermoregulation may persist in preterm infants after discharge with smaller preterm infants more likely to be underheated at home.126 Infants increase their temperature more easily with heat exposure and are less able to lose the heat via sweating.128 By 3 months of age the thermal balance has shifted to favor heat conservation. Mass-to-surface differences and metabolic rate, however, continue to increase so that net heat loss per unit of surface area in a 3-month-old is 50% higher than at birth.60 Children have a greater surface area–to–body mass ratio than adults. “In a warm environment this allows them to rely more on dry heat loss and less on evaporative cooling. However, in extreme conditions, hot or cold, the greater surface area–to–body mass ratio results in a higher rate of heat absorption or heat loss, respectively.”41
Higher mean temperatures predominate in infants. Average temperatures at 18 months of age are 37.7° C (99.8° F), with marked daily variations seen in some children. Body temperatures gradually decrease from 2 years to puberty, stabilizing at 13 to 14 years of age in girls and 16 to 17 years in boys.67
Summary
Thermal stability is one of the most critical processes during the transition to extrauterine life. Thermoregulation is essential for survival and has priority over most other metabolic demands. The infant who does not achieve thermal stability after birth or who is cold stressed in the neonatal period is more likely to experience health problems, poor growth, and increased morbidity and mortality. Ongoing assessment and monitoring of thermal status in the neonate and initiation of appropriate interventions to maintain thermal stability can prevent or minimize these risks. Clinical recommendations related to neonatal thermoregulation are summarized in Table 20-6.
Table 20-6
Recommendations for Clinical Practice Related to Thermoregulation in Neonates
Know the mechanisms of heat production and dissipation in the neonate (pp. 663-669).
Initiate interventions to conserve body heat and reduce neonatal heat loss during transition (pp. 661-662 and Table 20-2).
Recognize the usual values for neonatal temperature (pp. 661-662).
Avoid overheating or cooling the trigeminal area of the neonate’s face (pp. 664, 668).
Recognize and monitor for events that increase heat loss by conduction, convection, evaporation, and radiation (pp. 667-669 and Table 20-2).
Institute interventions to reduce heat loss in term and preterm infants by conduction, convection, evaporation, and radiation (pp. 667-669 and Table 20-2).
Provide term and preterm neonates with an appropriate thermal environment (pp. 666-669).
Know the methods for monitoring thermal status and their advantages and limitations (pp. 669-670).
Understand the differences between and implications of core versus skin temperature (pp. 669-670).
Know the advantages and limitations of different types of infant incubators and radiant warmers (pp. 670-671).
Use infant incubators and radiant warmers appropriately to maintain neonatal thermal status and reduce heat loss (pp. 670-671).
Monitor insensible water loss in infants cared for in radiant warmers (p. 671).
Provide opportunities for skin-to-skin care (pp. 671-672).
Use head coverings made of appropriate materials to reduce heat loss in infants in incubators and cribs (p. 672).
Recognize infants at risk for hypothermia and cold stress (p. 672 and Table 20-4).
Monitor neonates for signs of hypothermia and cold stress (p. 672).
Monitor hypothermic or cold-stressed infants for adverse physiologic effects (p. 672, Figure 20-7, and Table 20-5).
Recognize infants at risk for and signs of hyperthermia and thermal injury (pp. 673-674).
Monitor hyperthermic infants for adverse physiologic effects (pp. 673-674).
Use polyethylene wraps or bags with very low birthweight infants (pp. 661, 668-669, 672).