13 The renal system
Acetonuria: The appearance of acetone in the urine. Acetonuria is present when excessive fats are consumed or when an inadequate amount of carbohydrates is metabolized.
Albuminuria: The presence of protein in the urine, also called proteinuria. Albumin is the most common protein found in the urine. This condition usually indicates a malfunction in glomerular filtration.
Anuria: Lack of urine production, less than 50 mL/day.
Azotemia: The presence of nitrogenous products in the blood, usually because of decreased kidney function.
Cystitis: An inflammation of the bladder.
Dysuria: Painful or difficult urination.
Enuresis: Involuntary discharge of urine.
Glomerular Filtration Rate: Volume of fluid filtered through the kidney.
Glycosuria: The presence of glucose in the urine.
Hematuria: The presence of blood in the urine.
Nephritis: Inflammation of the kidney.
Nephrosis: Degeneration of the kidney without the occurrence of inflammation.
Oliguria: A decreased urine formation of less than 500 mL/day or 0.5 mL/kg/h in an adult.
Pyelonephritis: An inflammation of the renal pelvis and calices.
Stricture: An abnormal narrowing; in the urinary tract, a narrowing of the ureter or urethra.
Uremia: The presence of nitrogenous substances in the blood.
Urinary Incontinence: The inability to retain urine in the bladder.
Anatomy of the kidneys
The kidneys are two bean-shaped organs in the retroperitoneal spaces at the level of the twelfth thoracic to third lumbar vertebrae. The right kidney is slightly lower than the left. Each kidney weighs approximately 150 g. The notched portion of the kidney is called the hilum, which is where the ureter, the renal vein, and the renal artery enter the kidney (Fig. 13-1). The kidney is divided into an outer cortex and an inner medulla.
Blood is supplied to each kidney by a renal artery arising from each side of the abdominal aorta. The rate of blood flow through both kidneys of a man who weighs 70 kg is approximately 1200 mL/min, or approximately 21% of the cardiac output. As the renal artery enters the kidney at the hilum, it divides into the interlobar arteries. Branches from the interlobar arteries divide into afferent arteries that supply the capillaries of the nephrons. The capillaries form the efferent arterioles and divide to form the peritubular capillaries that help to supply the nephron. (Figs. 13-2 and 13-3).
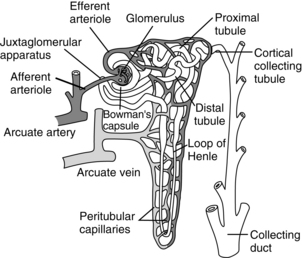
FIG. 13-2 Functional nephron.
(From Hall J: Guyton and Hall textbook of medical physiology, ed 12, Philadelphia, 2011, Saunders.)
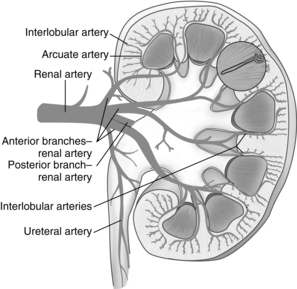
FIG. 13-3 Arterial supply to kidney. Soon after entering the hilum of the kidney, the renal artery divides into several anterior and posterior branches. Branches divide into interlobar arteries, which give off arcuate arteries that course between cortex and medulla.
The nephron is the functional unit of the kidney. Together the kidneys contain approximately 2.4 million closely packed nephrons. Each nephron consists of a glomerulus, a proximal convoluted tubule, a loop of Henle, a distal convoluted tubule, and collecting ducts. The blood enters the afferent arteriole and goes into the glomerulus located in the cortex. The glomerulus is a compact network of capillaries encased in a double-layered capsule, the Bowman capsule. Filtered blood flows out of the glomerulus into the efferent arteriole. The portion of the blood that is filtered drains into the proximal convoluted tubule. The renal tubules begin in the Bowman capsule. The pressure gradient, caused by renal artery blood flow, forces fluid to leave the glomerulus and enter the Bowman capsule. The filtrate flows into the proximal convoluted tubule, which is still in the cortex of the kidney, and then into the loop of Henle. The proximal loop of Henle is thick walled, but becomes thin at the distal segment in the medulla of the kidney. The filtrate then flows into the distal convoluted tubule, located in the cortex of the kidney, and passes into the collecting ducts. The collecting ducts traverse the cortex to the medulla, where they merge into the renal pelvis by way of the renal calyces. In the collecting ducts, the filtrate is termed urine.
Kidney physiology
Urine is formed by processes of filtration, reabsorption, and secretion. Filtration occurs as the blood passes through the glomerulus. The force of filtration is a pressure gradient that pushes fluid through the glomerular membrane. Approximately 180 L of water every 24 hours along with other substances is filtered out of plasma by the glomeruli (Table 13-1). Blood cells and heavy particles including proteins are retained in the blood because they are too large to pass through the glomerular epithelium. The presence of red blood cells or protein in the urine usually indicates a pathologic process in the kidney.
Regulation of kidney function
Countercurrent mechanism
The countercurrent mechanism is used by the kidneys to concentrate urine. The vasa recta are special capillaries that, with the loop of Henle, form the countercurrent mechanism. Fluids flow in opposite directions between the ascending and descending loops and sections of the vasa recta. The osmolality or weight of particles in solution of the interstitial fluid increases deeper into the medulla. The increase in osmolality results in active transport of particles or solutes into the interstitial fluid. The countercurrent mechanism is useful when the body needs to excrete a large amount of waste products yet reabsorb the normal amount of solutes and when the water in the body needs to be conserved, while waste products are eliminated.
Hormonal control
The juxtaglomerular complex is a group of cells, located just before the glomerulus and in close proximity to the distal tubule, which contain granules of inactive renin. Renin is released in response to reduced arterial blood pressure entering the afferent arteriole of the kidney or a low concentration of sodium in the distal tubule. The released renin acts as an enzyme to convert angiotensinogen to angiotensin I. Angiotensin I is converted to angiotension II by the angiotensin-converting enzyme, which leads to angiotensin II. Angiotensin II stimulates the adrenal cortex to release aldosterone. Aldosterone signals the kidney tubules to increase the reabsorption of sodium and retention of water. Because the renin-angiotensin system causes this reabsorption of water and sodium, it plays a role in the control of arterial blood pressure and is important in the conservation of sodium and control of fluid volume in hypotensive states (Fig. 13-4).