Chapter 91 The High-Risk Infant
Neonates at risk should be identified as early as possible to decrease neonatal morbidity and mortality (Chapter 87). The term high-risk infant designates an infant who should be under close observation by experienced physicians and nurses. Factors that define infants as being high-risk are listed in Table 91-1. Approximately 9% of all births require special or neonatal intensive care. Usually needed for only a few days, such observation may last from a few hours to several months. Some institutions find it advantageous to provide a special or transitional care nursery for high-risk infants, often within the labor and delivery suite. This facility should be equipped and staffed like a neonatal intensive care area.
DEMOGRAPHIC SOCIAL FACTORS
PAST MEDICAL HISTORY
PREVIOUS PREGNANCY
PRESENT PREGNANCY
LABOR AND DELIVERY
NEONATE
For many infants who are born prematurely, are small for gestational age (SGA), have significant perinatal asphyxia, are breech, or are born with life-threatening congenital anomalies, there are no previously identified risk factors. For any given duration of gestation, the lower the birthweight, the higher the neonatal mortality; for any given birthweight, the shorter the gestational duration, the higher the neonatal mortality (Fig. 91-1). The highest risk of neonatal mortality occurs in infants who weigh <1,000 g at birth and whose gestation was <28 wk. The lowest risk of neonatal mortality occurs in infants with a birthweight of 3,000-4,000 g and a gestational age of 38-42 wk. As birthweight increases from 500 to 3,000 g, a logarithmic decrease in neonatal mortality occurs; for every week of increase in gestational age from the 25th to the 37th wk, the neonatal mortality rate decreases by approximately half. Nevertheless, approximately 40% of all perinatal deaths occur after 37 wk of gestation in infants weighing 2,500 g or more; many of these deaths take place in the period immediately before birth and are more readily preventable than those of smaller and more immature infants. Neonatal mortality rates rise sharply for infants weighing over 4,000 g at birth and for those whose gestational period is 42 wk or longer. Because neonatal mortality largely depends on birthweight and gestational age, Figure 91-1 can be used to help identify high-risk infants quickly. This analysis is based on total live births and therefore describes the mortality risk only at birth. Because most neonatal mortality occurs within the 1st hours and days after birth, the outlook improves dramatically with increasing postnatal survival.
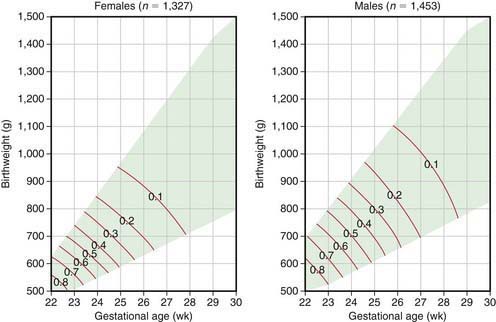
(From Lemons JA, Bauers CR, Oh W, et al: Very low birthweight outcomes of the National Institute of Child Health and Human Development Neonatal Research Network, January 1995 through December 1996, Pediatrics 107:2001; available at www.pediatrics.org.cgi/content/full/107/1/el.)
91.1 Multiple Gestation Pregnancies
Monozygotic Versus Dizygotic Twins
Examination of the Placenta
In the fetal transfusion syndrome, an artery from one twin acutely or chronically delivers blood that is drained into the vein of the other. The latter becomes plethoric and large, and the former is anemic and small. Generally, with chronicity, 5 g/dL hemoglobin and 20% body weight differences can be noted in this syndrome. Maternal hydramnios in a twin pregnancy suggests fetal transfusion syndrome. Anticipating this possibility by preparing to transfuse the donor twin or bleed the recipient twin may be lifesaving. Death of the donor twin in utero may result in generalized fibrin thrombi in the smaller arterioles of the recipient twin, possibly as the result of transfusion of thromboplastin-rich blood from the macerating donor fetus. Disseminated intravascular coagulation may develop in the surviving twin. Table 91-2 lists the more frequent changes associated with a large uncompensated arteriovenous shunt from the placenta of one twin to that of the other. Treatment of this highly lethal problem includes maternal digoxin, aggressive amnioreduction for polyhydramnios, selective twin termination, and Nd:YAG laser or fetoscopic ablation of the anastomosis.
Table 91-2 CHARACTERISTIC CHANGES IN MONOCHORIONIC TWINS WITH UNCOMPENSATED PLACENTAL ARTERIOVENOUS SHUNTS
TWIN ON | |
---|---|
ARTERIAL SIDE—DONOR | VENOUS SIDE—RECIPIENT |
Prematurity | Prematurity |
Oligohydramnios | Polyhydramnios |
Small premature | Hydrops |
Malnourished | Large premature |
Pale | Well nourished |
Anemic | Plethoric |
Hypovolemia | Polycythemic |
Hypoglycemia | Hypervolemic |
Microcardia | Cardiac hypertrophy |
Glomeruli small or normal | Myocardial dysfunction |
Arterioles thin walled | Tricuspid valve regurgitation |
Right ventricular outflow obstruction | |
Glomeruli large | |
Arterioles thick walled |
Prognosis
Most twins are born prematurely, and maternal complications of pregnancy are more common than with single pregnancies. The risk for twins is most often associated with twin-twin transfusion, assisted reproductive technology, and early-onset discordant growth. Although monochorionic twins have a significantly higher perinatal mortality, there is no significant difference between the neonatal mortality rates of twin births and single births in comparable weight and gestational age groups (Fig. 91-2). Because most twins are premature, their overall mortality is higher than that of single-birth infants. The perinatal mortality of twins is about four times that of singletons. Monoamnionic twins have an increased likelihood of entangling the cords, which may lead to asphyxia. Theoretically, the 2nd twin is more subject to anoxia than the 1st because the placenta may separate after birth of the 1st twin and before birth of the 2nd. In addition, delivery of the 2nd twin may be difficult because it may be in an abnormal presentation (breech, entangled), uterine tone may be decreased, or the cervix may begin to close after the 1st twin’s birth. The mortality for multiple gestations with four or more fetuses is excessively high for each fetus. Because of this poor prognosis, selective fetal reduction (with transabdominal intrathoracic fetal injection of KCl) to two to three fetuses has been offered as a treatment option. Monozygotic twins have an increased risk of one twin dying in utero. The surviving twin has a greater risk for cerebral palsy and other neurodevelopmental sequelae.
Airas U, Heinonen S. Clinical significance of true umbilical knots: a population-based analysis. Am J Perinatol. 2002;19:127-132.
American Academy of Pediatrics Committee on Fetus and Newborn. Hospital discharge of the high-risk neonate. Pediatrics. 2008;122:1119-1126.
Anderson AN, Pinborg A, Loft A. Neonatal outcome in singletons conceived after ART. Lancet. 2008;372:694-695.
Fraga MF, Ballestar E, Paz MF, et al. Epigenetic differences arise during the lifetime of monozygotic twins. PNAS. 2005;102:10606-10609.
Garite TJ, Clark RH, Elliott JP, et al. Twins and triplets: the effect of plurality and growth on neonatal outcome compared with singleton infants. Am J Obstet Gynecol. 2004;191:700-707.
Hansen M, Colvin L, Petterson B, et al. Twins born following assisted reproductive technology: perinatal outcome and admission to hospital. Hum Reprod. 2009;9:2321-2331.
Mari G, Roberts A, Detti L, et al. Perinatal morbidity and mortality rates in severe twin-twin transfusion syndrome: results of the International Amnioreduction Registry. Am J Obstet Gynecol. 2001;185:708-715.
Ortibus E, Lopriore E, Deprest J, et al. The pregnancy and long-term neurodevelopment outcome of monochorionic diamniotic twin gestations: a multicenter prospective cohort study from the first trimester onward. Am J Obstet Gynecol. 2009;200:e1-494.e8.
Pearn J. Bioethical issues in caring for conjoined twins and their parents. Lancet. 2001;357:1968-1971.
Steer P. Perinatal death in twins. BMJ. 2007;334:545-546.
Strömberg B, Dahiquist G, Ericson A, et al. Neurological sequelae in children born after in-vitro fertilisation: a population-based study. Lancet. 2002;359:461-465.
91.2 Prematurity and Intrauterine Growth Restriction
Definitions
Liveborn infants delivered before 37 wk from the 1st day of the last menstrual period are termed premature by the World Health Organization. LBW (birthweight of 2,500 g or less) is due to prematurity, poor intrauterine growth (IUGR, also referred to as SGA), or both. Prematurity and IUGR are associated with increased neonatal morbidity and mortality. Ideally, definitions of LBW for individual populations should be based on data that are as genetically and environmentally homogeneous as possible. As previously mentioned, Figure 91-1 presents variations in mortality based on birthweight, gestational age, and gender.
Incidence
There is an increasing percentage of deaths in children <5 yr of age that occur in the neonatal period. Approximately 57% of deaths in this age group occur within the 1st mo of life, of which approximately 36% are attributable to premature birth. In 2008, 8.2% of liveborn neonates in the USA weighed <2,500 g; the rate for blacks was almost twice that for whites. Over the past 2 decades, the LBW rate has increased primarily because of an increased number of preterm births. Women whose 1st births are delivered before term are at increased risk for recurrent preterm delivery. Approximately 30% of LBW infants in the USA have IUGR and are born after 37 wk. At LBW rates >10%, the contribution of IUGR increases and that of prematurity decreases. In developing countries, approximately 70% of LBW infants have IUGR. Infants with IUGR have greater morbidity and mortality than do appropriately grown, gestational age–matched infants (see Fig. 91-1). Although U.S. infant mortality rates have fallen since 1971, the ethnic disparity between black infants and white or Hispanic infants remains unchanged. Black infants have higher neonatal mortality rates and comprise a larger percentage of low birthweight births in the USA.
The incidence of preterm births in the USA continues to rise (Fig. 91-3) and is due in part to multiple gestation pregnancies. In single births, the overall incidence has been stable, but premature births due to medically indicated deliveries have increased, whereas premature births due to spontaneous preterm birth or ruptured membranes have declined (Fig. 91-4).
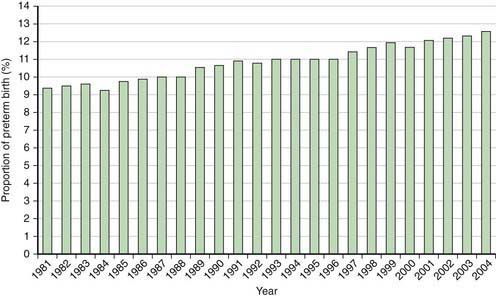
Figure 91-3 Percentage of all births classified as preterm in the USA, 1981-2004.
(From Martin JA, Kochanek KD, Strobino DM, et al: Annual summary of vital statistics—2003, Pediatrics 115:619–634, 2005.)
Factors Related to Premature Birth and Low Birthweight
It is difficult to separate completely the factors associated with prematurity from those associated with IUGR (Chapters 88 and 89). A strong positive correlation exists between both preterm birth and IUGR and low socioeconomic status. Families of low socioeconomic status have higher rates of maternal undernutrition, anemia, and illness; inadequate prenatal care; drug misuse; obstetric complications; and maternal history of reproductive inefficiency (abortions, stillbirths, premature or LBW infants). Other associated factors, such as single-parent families, teenage pregnancies, short interpregnancy interval, and mothers who have borne more than four previous children, are also encountered more frequently in such families. Systematic differences in fetal growth have also been described in association with maternal size, birth order, sibling weight, social class, maternal smoking, and other factors. The degree to which the variance in birthweight among various populations is due to environmental (extrafetal) rather than genetic differences in growth potential is difficult to determine.
The etiology of preterm birth is multifactorial and involves a complex interaction between fetal, placental, uterine, and maternal factors (Table 91-3).
Table 91-3 IDENTIFIABLE CAUSES OF PRETERM BIRTH
FETAL
PLACENTAL
UTERINE
MATERNAL
OTHER
IUGR is associated with medical conditions that interfere with the circulation and efficiency of the placenta, with the development or growth of the fetus, or with the general health and nutrition of the mother (Table 91-4). Many factors are common to both prematurely born and LBW infants with IUGR. IUGR is associated with decreased insulin production or insulin (or insulin-like growth factor [IGF]) action at the receptor level. Infants with IGF-I receptor defects, pancreatic hypoplasia, or transient neonatal diabetes have IUGR. Genetic mutations affecting the glucose-sensing mechanisms of the pancreatic islet cells that result in decreased insulin release (loss of function of the glucose-sensing glucokinase gene) give rise to IUGR.
Table 91-4 FACTORS OFTEN ASSOCIATED WITH INTRAUTERINE GROWTH RESTRICTION
FETAL