The acute respiratory distress syndrome
Background and definitions
The acute respiratory distress syndrome (ARDS) is an inflammatory lung condition with associated noncardiogenic pulmonary edema and impairment of gas exchange. ARDS is a major cause of respiratory failure in patients in the intensive care unit (ICU). In the perioperative period, patients who are undergoing major surgical procedures, who are seriously ill, or who aspirate are susceptible to developing ARDS. In 2012, ARDS was redefined according to the Berlin definition (Table 227-1) in an effort to overcome some of the inadequacies of the previously used American-European Consensus definition, which dated from 1994 (Table 227-2). Compared with the prior definition, the Berlin definition defines “acute,” clarifies the methods to exclude hydrostatic edema, adds minimal ventilator-setting requirements, drops the term “acute lung injury,” and classifies ARDS into three categories of severity.
Table 227-1
Berlin Definition of Acute Respiratory Distress Syndrome
Feature | Description |
Timing | Onset within 1 week of a known clinical insult or new or worsening respiratory symptoms |
Chest imaging* | Bilateral opacities—not fully explained by effusions, lobar/lung collapse, or nodules |
Origin of edema | Respiratory failure not fully explained by cardiac failure or fluid overload; need objective assessment (e.g., echocardiography) to exclude hydrostatic edema if no risk factor is present |
Oxygenation† | |
Mild | 200 mm Hg < PaO2/FIO2 ≤ 300 mm Hg with PEEP or CPAP ≥ 5 cm H2O‡ |
Moderate | 100 mm Hg < PaO2/FIO2 ≤ 200 mm Hg with PEEP ≥ 5 cm H2O |
Severe | PaO2/FIO2 ≤ 100 mm Hg with PEEP ≥ 5 cm H2O |
*Chest radiograph or computed tomography scan.
†If altitude is greater than 1000 m, the correction factor should be calculated as follows: (PaO2/FIO2) × (Barometric pressure/760).
‡This may be delivered noninvasively in the mild ARDS group.
Modified from ARDS Definition Task Force. Acute respiratory distress syndrome: The Berlin definition. JAMA. 2012;307:2526-2533.
Table 227-2
American-European Consensus Conference Definitions for Acute Lung Injury and Acute Respiratory Distress Syndrome*
Feature | ALI | ARDS |
Timing | Acute | Acute |
Findings on chest radiograph | Bilateral diffuse infiltrates | Bilateral diffuse infiltrates |
PCOP (mm Hg) | ≤18 | ≤18 |
PaO2/FIO2 | ≤300 | ≤200 |
*The original intent with these definitions was to designate acute respiratory distress syndrome (ARDS) as a subset of acute lung injury (ALI). In practice, however, many clinicians used the term ALI exclusively to indicate those patients with ALI but without ARDS (i.e., a PaO2/FIO2 ratio of 201-300). The Berlin definition dropped the “ALI” category. (Although superseded by the Berlin definition, these criteria were used as a basis for interventional and epidemiologic studies between 1994 and 2012.)
Ventilator-associated lung injury is a major concern (Figure 227-1). Inspired gas flows preferentially to relatively uninvolved alveoli, potentially causing overdistention and lung injury due to volutrauma and barotrauma. Constant opening and closing of derecruited lung units can lead to shear stress (atelectrauma). These physical forces can lead to an increase in the injurious inflammatory response (biotrauma). Laboratory investigations have suggested that a “safe zone” exists on the pulmonary pressure-volume curve defined by lower and upper “inflection points” in which ventilation should occur. At the lower end of the pressure-volume curve, lung units are susceptible to derecruitment and atelectasis, and, at the upper end of the pressure-volume curve, overdistention leads to lung injury (Figure 227-2).
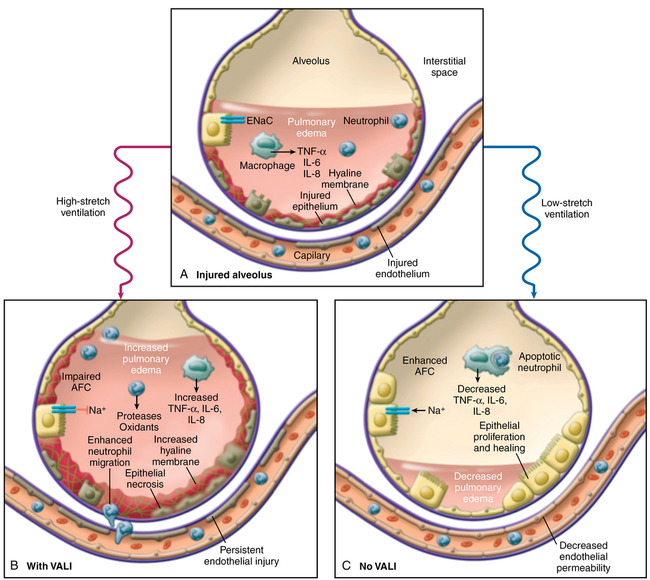
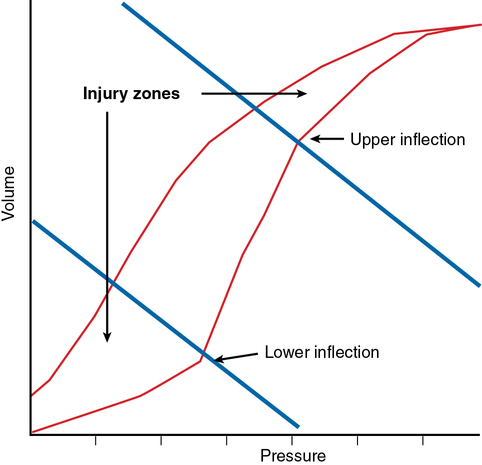
Treatment
A variety of techniques have been used in patients with ARDS to allow adequate oxygenation and ventilation while attempting to minimize ventilator-induced lung injury. Selected major clinical trials of therapeutic interventions for ARDS are listed in Table 227-3.
Table 227-3
Selected Major Clinical Trials in Acute Respiratory Distress Syndrome
Intervention | Study | No. of Patients | Result |
Lung-protective strategy | ARDSNet Investigators, 2000 (ARDSNet ARMA trial) | 861 | Ventilation with 6 mL/kg IBW decreased mortality rate (versus 12 mL/kg) |
High PEEP | Brower RG, et al, 2004 (ARDSNet ALVEOLI trial) | 549 | No difference in mortality rate with high (versus low) PEEP strategy |
Fluid strategy | Wiedemann HP, et al, 2006 (ARDSNet FACTT) | 1000 | More ventilator-free days with fluid-conservative strategy |
PAC versus CVC | Wheeler AP, et al, 2006 (ARDSNet FACTT) | 1000 | No improvement in survival or organ function but more complications with PAC-guided therapy |
Neuromuscular blockade | Papazian L, et al, 2010 (ACURASYS study) | 340 | Decrease in mortality rate with 48 hours of neuromuscular blockade in severe ARDS |
Methylprednisolone | Steinberg KP, et al, 2006 (ARDSNet LASRS study) | 180 | No difference in mortality rate with steroid administration in late-phase ARDS |
Prone position | Taccone P, et al, 2009 (PRONE-SUPINE II Study Group) | 342 | No difference in mortality rate |
Extracorporeal membrane oxygenation | Peek GJ, et al, 2009 (CESAR study) | 180 | Decrease in mortality rate but results not conclusive |
Inhaled nitric oxide | Taylor RW, et al, 2004 | 385 | No difference in mortality rate |
β2-Agonist | Gao Smith F, et al, 2012 (BALTI-2 trial) | 324 | Increase in mortality with intravenous β2-agonist |
ω-3 fatty acid, γ-linoleic acid, and antioxidant supplementation | Rice TW, et al, 2011 (OMEGA study) | 272 | No difference in ventilator-free days or mortality rate |
HFOV | Ferguson ND, et al, 2013 (OSCILLATE trial) | 548 | HFOV does not reduce, and may increase, in-hospital mortality rate |
Other therapeutic interventions
The use of corticosteroids has not been demonstrated to be advantageous in either early-phase or late-phase ARDS. Despite initial enthusiasm for the use of corticosteroids in the fibroproliferative stage of ARDS, the ARDSNet Late Steroid Rescue Study failed to show an outcome benefit. Steroids are indicated, however, in certain circumstances (e.g., ARDS associated with “peri-engraft syndrome” in patients after hematopoietic stem cell transplantation). Immunomodulatory nutrition formulations, β-adrenergic receptor agonists, and novel surfactant preparations have also been investigated as adjunctive therapies for ARDS, but, so far, the results have been disappointing (see Table 227-3).
Mortality and morbidity
The mortality rate associated with ARDS has decreased over the past decade. Recent clinical trials in ARDS have demonstrated mortality rates of approximately 25% to 45%, with lower mortality rates reported in young patients who developed ARDS following polytrauma and higher mortality rates in older patients admitted to medical ICUs (Figure 227-3). As alluded to previously, although some patients die as a result of hypoxemia, most deaths are due to sepsis, multiple organ failure, and the underlying disease rather than to ARDS itself. Initial indices of oxygenation and ventilation do not predict outcome, although failure to improve after 1 week of therapy and persistently high minute ventilation requirements are signs of poor prognosis. Advanced age and the presence of comorbid conditions also increase the chance of death.
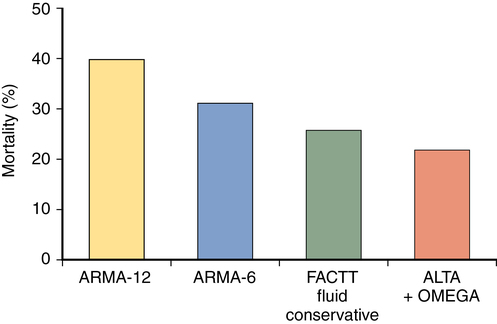