CHAPTER 36 Systemic Disorders
Among patients who present special problems for anesthesiologists are children whose underlying conditions complicate anesthetic management and may be associated with an increased risk of morbidity. The number of rare diseases that may be encountered in infants and children is great, although only a few are mentioned here. Chosen for discussion are the diseases most commonly seen, those carrying an increased risk related to anesthetic management, and a few of unusual interest. Modifications to the understanding of mechanisms of coagulation are included, along with consideration of coagulopathic states, and there is a comprehensive review of the anesthetic implications of pediatric syndromes associated with genetic, metabolic, and dysmorphic features (Baum and O’Flaherty, 2006). A partial list of syndromes with possible anesthetic implications is included in Appendix D, which can be accessed online at www.expertconsult.com
Endocrine Disorders
Diabetes Mellitus
The endocrine condition most commonly dealt with in the perioperative period is the management of glucose homeostasis in children with diabetes mellitus. The prevalence of type 1 (insulin-dependent) diabetes in the United States has remained stable for the past 15 years at 1 in 400 to 600 school-aged children, whereas the incidence of type 2 diabetes is increasing, especially among American Indian, Black, and Hispanic children and adolescents (CDC, 2007c). Diabetes mellitus is the result of an absolute or functional deficiency of insulin production by the pancreas. In type 1 diabetes, this deficiency is caused by an autoimmune pathophysiologic process. Insulin deficiency results in abnormalities of glucose transport and storage and of lipid and protein synthesis. Over time, these metabolic derangements result in the vascular pathology that leads to end-stage complications of renal, cardiac, and eye disease—diseases that typically do not occur before adulthood. The anesthetic implications of type 1 diabetes in children differ from those in adults with the same disease, for whom the primary concern is the type and severity of end-organ disease.
Children with type 1 diabetes may be treated with various types of insulin on a daily basis to maintain tight glucose control with the aid of frequent blood-glucose monitoring. Since 1982, most newly approved insulin preparations have been produced using recombinant DNA technology with laboratory-cultivated bacteria or yeast. This process allows the bacteria or yeast cells to produce complete human insulin. Recombinant human insulin has mostly replaced animal-derived insulin (e.g., pork and beef insulin) in diabetes management (Plotnick and Henderson, 1998). Insulin products called insulin analogues are produced so that the structure differs slightly from human insulin (by one or two amino acids) to change onset and peak of action. An example of an analogue is human lispro, an ultra–short-acting insulin that is given only 15 minutes before a meal. Its peak and duration of action parallel the glucose rise that results from carbohydrate ingestion.
Another new insulin is glargine, which almost mimics an insulin pump, providing a continuous, 24-hour, low background level of insulin. The kinetics of some of the insulin preparations most commonly used in children are listed in Table 36-1. Some children’s diabetes may be managed with an external insulin pump, which provides a low, background, subcutaneous infusion of insulin and the ability to give small boluses before meals. Most children with diabetes administer insulin at least three times each day and check their blood sugar at least four times each day. Type 2 diabetes in children and adolescents may be controlled with diet and exercise, but these children also may be taking metformin. Metformin was recently shown to decrease gluconeogenesis by directly modulating copper-binding protein (CBP) in the liver much as insulin itself does, rather than by overcoming the liver’s decreased sensitivity to insulin (He et al., 2009).
Insulin is an anabolic hormone that promotes glycogen and triglyceride storage and protein synthesis. Present in small amounts even in the fasting state, it decreases glycogenolysis, gluconeogenesis, and lipolysis, with resultant ketogenesis and protein breakdown. Its complete absence at the time of surgery puts the patient in a state of starvation, in which caloric intake is greatly restricted and substrate demands (e.g., for healing) are at their highest. The risk of a catabolic state is increased by the release of stress hormones, including catecholamines, cortisol, and glucagon. Perioperative insulin administration is essential to control glucose and to promote an anabolic state, which is most conducive to speedy healing and metabolic homeostasis. Preoperative anesthesia evaluation for elective procedures, informed by contemporaneous endocrine assessment of adequacy of glucose control, should be completed 7 to 10 days before the scheduled date of surgery to allow adjustment of treatment regimen or delay of procedure if control is not optimal. Rhodes and colleagues (2005) published a comprehensive review of concerns and perioperative management of pediatric patients with diabetes; it features an extremely useful clinical practice guideline that incorporates both preoperative assessment and choice of preoperative insulin regimen.
Preoperative Evaluation
The preoperative evaluation should include measurements of the hematocrit, electrolyte levels, and glucose levels. A hemoglobin (Hb) A1c level (i.e., glycosylated Hb assay), although a useful index of long-term glucose control, is unlikely to affect the anesthetic plan and is not a necessary preoperative test (Nathan et al., 1984). If glycohemoglobin results are available, it is important to remember that different laboratories have different ranges for Hb A1c in normal subjects. Even in the same laboratory, the normal range may change from time to time. It is therefore important to know the laboratory’s normal range to interpret results in patients with diabetes. The normal range of Hb A1c is 4.5% to 6.1%, but the normal range also varies with age (Rhodes et al., 2005; Custer and Rau, 2009).
Several systemic abnormalities may be present in the child with diabetes. Nineteen percent of children with diabetes have a vital capacity two standard deviations below the predicted mean value, suggesting the presence of restrictive lung disease (Buckingham et al., 1986). No apparent association exists between decreased vital capacity and duration of diabetes or presence of other diabetic complications. Abnormal lung elasticity and thickening of the alveolar basal laminae have been reported in children with diabetes (Schuyler et al., 1976; Vracko et al., 1980). Routine preoperative pulmonary function tests are not indicated in the asymptomatic child who has diabetes.
Decreased atlantooccipital joint mobility, resulting in difficult intubation, may be present in a subset of adolescents with a syndrome of diabetes mellitus, short stature, and tightness of small joints of the fingers, wrists, ankles, and elbows (Salzarulo and Taylor, 1986). Abnormal cross-linking of collagen by nonenzymatic glycosylation is the postulated cause of this syndrome (Chang et al., 1980).
Perioperative Management
Various regimens for managing insulin therapy perioperatively have been proposed, three of which are discussed in the following sections and in Table 36-2: a classic regimen, the subcutaneous infusion insulin pump, and intravenous (IV) insulin infusion. Essential to optimal management, regardless of regimen, is the scheduling of elective surgery for the child with diabetes as early as possible in the day (first case) to minimize time that the patient must fast. The fasting interval should be the same as that recommended for patients who do not have diabetes: no solid food or milk for 8 hours, and clear liquids are permissible until 2 hours before the scheduled time of surgery (Schreiner et al., 1990). Children with diabetes should be encouraged to continue taking clear liquids until 2 hours before surgery. If this is not possible, an IV fluid infusion should be started (described later). As recommended in adult patients with type 2 diabetes, metformin should be stopped 48 hours before surgery, based on reports of lactic acidosis in patients who remain on the drug and are in a fasting state perioperatively. Other orally administered medications (e.g., thiazolidinediones or sulfonylureas) may be continued through the day before surgery.
Regimen | Morning of Surgery Procedure |
Classic regimen | Start IV infusion of 5% dextrose in 0.45% saline or Ringer’s lactate solution at 1500 mL/m2/day. Administer half of usual morning insulin dose as regular insulin. Check blood glucose before induction, during and after anesthesia |
Continuous insulin infusion | Start IV infusion of 5% dextrose in 0.45% saline or Ringer’s lactate solution at 1500 mL/m2/day. Add 1-2 units of insulin per 100 mL of 5% dextrose Starting insulin dose = 0.02 units/kg/hr Check blood glucose before induction, and during and after anesthesia |
Insulin- and glucose-free regimen (for operative procedures of short duration) | Withhold morning insulin dose If indicated for procedure, give glucose-free solution (e.g., Ringer’s lactate) at maintenance rate. Check blood glucose before induction, and during and after anesthesia. |
Based on the blood-glucose level determined on arrival to the preoperative facility and before implementation of the regimens discussed in the following sections, glucose or insulin should be administered according to the scheme outlined in Table 36-3.
TABLE 36-3 Preoperative Glucose and Insulin Management for Diabetic Patients
Blood Glucose Level | Management |
<80 mg/dL | 2 mL/kg D10W followed by glucose infusion |
80-250 mg/dL | D5/0.45 NS or D10/0.45 NS solution at maintenance if insulin is to be administered; 0.9 NS if short case; no insulin |
>250 mg/dL | Administer rapid-acting (lispro) or short-acting (regular) insulin SC to reduce blood sugar; use correction factor from patient’s endocrine provider or 0.2 unit/kg SC |
>350 mg/dL | Consider canceling or postponing surgery, especially if ketonuria |
NS, Normal saline; SC, subcutaneously.
Classic Regimen
On the morning of surgery, one half of the usual dose of long-acting insulin (e.g., Neutral Protamine Hagedorn [NPH]) is administered subcutaneously after establishing an IV infusion of 5% glucose-containing solution at a rate of 100 mg/kg per hour of glucose (Table 36-2). Plasma-glucose concentrations should be maintained between 100 and 180 mg/dL. This target range is chosen because mild to moderate hyperglycemia (without ketosis) usually does not present a serious problem to the child, whereas hypoglycemia has devastating consequences. Hyperglycemia greater than 250 mg/dL should be avoided because of associated mental status changes, diuresis, and subsequent dehydration, which can occur because of the hyperosmolar state. Hyperglycemia has been associated with poorer outcomes in patients at risk for central nervous system (CNS) ischemia, including those undergoing cardiopulmonary bypass (Lanier et al., 1987; Lanier, 1991). Hyperglycemia has also been shown to impair wound healing and has adverse effects on neutrophil function in vitro. (Marhoffer et al., 1992; Delamaire et al., 1997). When the classic regimen is employed, supplemental subcutaneous doses of short-acting insulin can be given on a sliding scale postoperatively to maintain the desired plasma glucose level. This regimen should be restricted to patients who are scheduled for short surgical procedures, after which they are expected to resume eating promptly.
Subcutaneous Infusion Insulin Pump
Increasing numbers of pediatric patients with type I diabetes are being managed with an external insulin pump that is capable of subcutaneous administration of both continuous and bolus doses of insulin. Such pumps afford excellent control, with changes in administration coordinated with eating, exercise, and stress. At this time, the proliferation of pumps from multiple manufacturers precludes the easy acquisition of knowledge and familiarity with their use in the perioperative setting. Some clinicians and institutions allow continued use of insulin pumps for short, uncomplicated procedures (e.g., less than 2 hours), whereas most recommend transition to a continuous insulin infusion, as described in the next section (Glister and Vigersky, 2003; Rhodes et al., 2005).
Intravenous Insulin Infusion
If a long procedure or a prolonged period of postoperative fasting is anticipated, the continuous IV infusion of glucose and insulin may provide the best control. On the morning of surgery, a glucose infusion is begun at a maintenance rate of 100 mg/kg per hour, with an insulin infusion of 0.02 to 0.05 unit/kg per hour “piggy-backed” into the glucose infusion. The glucose infusion can be D5 or D10 in half-normal saline with 10 to 20 mEq/L of potassium chloride. These infusions should be started 2 hours before surgery to minimize the duration of fasting and decrease the risk of the development of a catabolic state. Insulin is absorbed by IV bags and tubing. When the insulin solution is prepared, the first portion of the solution should be run through the tubing and discarded to saturate the sites in the tubing that bind insulin (Kaufman et al., 1996). Blood glucose levels should be checked hourly for the first few hours, and adjustments of 0.01 unit/kg per hour in the insulin rate should be made to keep the blood sugar in the acceptable range of 100 to 180 mg/dL. This continuous-infusion regimen has been shown to yield better control of glucose concentrations than the regimen in which intermittent subcutaneous insulin is administered (Kaufman et al., 1996). The administration of intermittent large IV-insulin doses has no role, as it can result in large swings in glucose concentration (high and low) and a greater chance of lipolysis and ketogenesis. Patients with insulin pumps should have them turned off in the perioperative period, and pumps should be replaced by the continuous-infusion regimen, as most anesthesiologists are not familiar with the details of operation of such pumps. Fifty-percent dextrose solution should be available for administration in case of the development of hypoglycemia; 0.1 g/kg of dextrose raises the blood-glucose level by approximately 30 mg/dL.
Alternative Procedure (Insulin- and Glucose-free Regimen)
For extremely brief procedures, after which prompt resumption of oral intake is expected, an alternative protocol involves the administration of no insulin or glucose before or during surgery. When oral intake is established postoperatively, 40% to 60% of the usual daily insulin dose is given (Stevens and Roizen, 1987). Myringotomy with tube placement is an example of a procedure for which this regimen would be appropriate. The surgical procedure should be performed as the first case on the morning schedule to avoid prolonged fasting and excessive delay in insulin administration.
The most serious perioperative complication that can occur in the diabetic child is hypoglycemia. Common signs of low blood-glucose levels include tachycardia, tearing, diaphoresis, and hypertension. In the anesthetized patient, these signs may be misinterpreted as the result of inadequate anesthesia. Because the clinical signs of hypoglycemia are masked by sedation or anesthesia, frequent (every hour) measurement of the serum-glucose level is critical for the prevention of hypoglycemia, independent of the glucose-insulin regimen chosen. Glucose test strips, with or without the use of a reflectance photometer, provide quick, convenient, and reliable bedside blood-sugar measurements to guide therapy. Blood-glucose determinations performed with reflectance photometers provide results that are generally within 10% of clinical laboratory glucose determinations done on the same specimen (Chen et al., 2003). Visual evaluation of blood-glucose strips is less accurate (Arslanian et al., 1994). Postoperative insulin administration is determined by the time of resumption of oral or enteral feeding and by the postoperative blood-glucose concentration. The endocrinologist and surgeon should be active partners in the choice of an appropriate insulin regimen, because they are responsible for monitoring glucose homeostasis after the patient leaves the recovery room. For day-surgery patients, contingency planning for insulin management and mechanisms for follow-up care and consultation should be clearly defined for members of the care team and family.
Perioperative Management of Diabetic Ketoacidosis
Occasionally, patients with diabetes require surgery for trauma or infection while they are in a state of ketoacidosis. Diabetic ketoacidosis includes hyperglycemia (plasma-glucose concentration greater than 300 mg/dL) with glucosuria, ketonemia (ketones strongly positive at greater than 1:2 dilution of serum), ketonuria, and acidemia (pH lower than 7.30, serum bicarbonate lower than 15 mEq/L, or both). It is common for intraabdominal catastrophes with infection (e.g., appendicitis) to precipitate ketoacidosis. Foster and McGarry (1983) have succinctly summarized the pathophysiology of diabetic ketoacidosis. The initiating event is usually cessation of insulin therapy or onset of stress that renders the usual dose of insulin inadequate. Glucagon, catecholamines, cortisol, and growth hormone levels increase. A catabolic state is produced as substrates are mobilized, resulting in hepatic production of glucose and ketone bodies, which causes hyperglycemia and ketoacidosis. Subclinical brain swelling nearly always occurs during diabetic ketoacidosis therapy, although most patients remain asymptomatic (Krane et al., 1985). Fatalities from cerebral edema do occur, and some studies suggest that high rates of fluid administration early in treatment (more than 50 mL/kg in the first 4 hours) greatly increase the risk of herniation (Mahoney et al., 1999). Studies using 4 L/m2 for the first 24 hours followed by 1 to 1.5 times maintenance resulted in clearance of ketoacidosis equal to that in patients who were given more fluid, but a low but persistent incidence (0.35% to 0.5%) of symptomatic cerebral edema remained (Felner and White, 2001). The best methods to prevent the development of this devastating complication are administration of isotonic fluid only and frequent monitoring of serum osmolality (by direct measurement or calculation) to ensure that elevated osmolality is reduced gradually. Insulin therapy should be tailored to decrease the blood glucose concentration at a rate not greater than 100 mg/dL per hour. To prevent a more rapid decrease in blood glucose concentration, 5% dextrose and if necessary, 10% dextrose, should be added to the rehydration solution to slow the rate of fall, rather than decreasing the rate of insulin infusion (Arslanian et al., 1994). Fortunately, the anesthesiologist is rarely called on to administer anesthesia during this severe metabolic derangement. If an anesthetic is required during diabetic ketoacidosis, preoperative attention should be directed toward the correction of hypovolemia and hypokalemia, along with beginning an insulin infusion. Invasive hemodynamic monitoring may be indicated preoperatively to optimize the patient’s fluid and electrolyte balance and to monitor the patient’s hemodynamic status accurately. Surgery should not be delayed inordinately because it may be impossible to correct the metabolic derangements before the underlying source of infection or organ dysfunction is corrected. For patients with signs of cerebral edema, monitoring of intracranial pressure may be necessary.
Diabetes Insipidus
Diabetes insipidus (DI) is a clinical syndrome of hypotonic polyuria in the face of elevated plasma osmolality that results from inadequate production of, or inadequate response to, antidiuretic hormone (ADH). Central DI results from inadequate production or release of ADH from the posterior pituitary gland. ADH is synonymous with arginine vasopressin. Nephrogenic DI (also referred to as vasopressin-resistant DI) is characterized by partial or complete renal tubular unresponsiveness to endogenous ADH or exogenously administered arginine vasopressin. Congenital nephrogenic DI is caused by mutations in either the vasopressin receptor or aquaporin-2 gene. Inheritance is X-linked in the former and autosomal recessive or dominant in the latter (Sasaki, 2004). A combination of hydrochlorothiazide and a nonsteroidal antiinflammatory drug (NSAID) has been effective for the treatment of nephrogenic DI. Both cyclooxygenase-1 (COX-1; i.e., tolmetin and indomethacin) and cyclooxygenase-2 (COX-2; i.e., rofecoxib) drugs have been effective (Jakobsson and Berg, 1994; Pattaragarn and Alon, 2003). Toxic drug effects may also lead to acquired nephrogenic DI. Anesthetic implications of nephrogenic DI have been reviewed by Cramolini (1993) and Malhotra and Roizen (1987).
The causes of DI are outlined in Box 36-1. This discussion focuses on central DI and its clinical manifestations, which are polyuria and polydipsia. The urine is hypotonic relative to the plasma. The urine osmolality is usually less than 200 mOsm/L, and urine specific gravity is less than 1.005 (Custer and Rau, 2009). When the patient has had inadequate access to water, severe dehydration and hypernatremia ensue, because a large volume of dilute urine is continually produced.
Box 36-1 Causes of Diabetes Insipidus
Patients with preexisting DI may need incidental surgery. They are usually taking maintenance doses of vasopressin, which for relatively short, uncomplicated, elective procedures, should be continued through the perioperative period (Wise-Faberowski et al., 2004). Desmopressin (1-desamino-8-d-arginine vasopressin, or DDAVP), a longer-acting (8 to 20 hours) vasopressin analogue, has a decreased vasopressor effect relative to its antidiuretic effect (Hays, 1990). DDAVP is usually given intranasally (2.5 to 10 mcg once or twice daily) or orally (25 to 200 mcg once or twice daily) to prevent diuresis (Lee et al., 1976). DDAVP also may be given subcutaneously or intravenously (1 to 2 mcg twice daily). An algorithm for the management of DI, whether preexisting or developing intraoperatively or postoperatively, is presented in Figure 36-1.
The most common situation encountered by the anesthesiologist, however, is the new onset of DI intraoperatively or postoperatively in patients who are having surgery for pituitary or hypothalamic tumors, most commonly craniopharyngiomas; 70% to 90% of these patients develop DI (Lehrnbecher et al., 1998; Ghirardello et al., 2006;). Perioperative DI may present in one of four ways:
If any degree of DI is going to occur, the onset is most commonly within 18 hours after the operation. Recommendations for therapy are reviewed by Wise-Faberowski and colleagues (2004).
The goal of perioperative management of DI is to maintain normal fluid and electrolyte balance, urine output, and hemodynamic stability. Urine output may be prodigious (10 to 20 mL/kg per hour). Care must be taken to differentiate polyuria caused by DI (urine specific gravity of less than 1.005) from diuresis caused by mannitol administration, hyperglycemia (urine specific gravity that is usually greater than 1.015), or simple excessive administration of crystalloid (urine specific gravity of greater than 1.005). Patients with partial ADH deficiency usually do not require supplemental aqueous vasopressin perioperatively, because large quantities of ADH are produced in response to surgical stress (Malhotra and Roizen, 1987). However, serum osmolality should be measured often, and aqueous vasopressin should be given if the plasma osmolality exceeds 300 mOsm/L (Wise-Faberowski et al., 2004).
If central DI is present preoperatively and the planned surgery is prolonged, an infusion of aqueous vasopressin is begun preoperatively and continued intraoperatively. The recommendations for adults include a bolus of 100 milliunits of aqueous vasopressin followed by a continuous infusion of 100 to 200 mU/hr. This should be accompanied by the intraoperative administration of isotonic saline at two thirds of the maintenance rate, with additional fluid given for blood loss replacement and for maintaining hemodynamic stability (Malhotra and Roizen, 1987). Hypotonic fluids should be avoided, as hyponatremia may result. For the pediatric population, an infusion is begun at 0.5 mU/kg per hour and increased in 0.5 mU/kg per hour increments until a urine osmolality twice that of plasma and a urine output of less than 2 mL/kg per hour are achieved. It is rarely necessary to use more than 10 mU/kg per hour (Weigle, 1987). Side effects from vasopressin administration are minimal at doses used for antidiuresis; at larger doses, generalized vasoconstriction can occur and has resulted in tissue ischemia and myocardial infarction.
DDAVP, rather than aqueous vasopressin, may also be used for treatment of perioperative DI because of its potent antidiuretic effect with minimal pressor activity or other side effects. In the perioperative period, it may be given intravenously until intranasal administration can be started or resumed. The suggested IV dose is 0.5 to 4 mcg, with a single dose having a duration of action of 8 to 12 hours (Muglia and Majzoub, 2008). It is important to note that this dose of DDAVP is one fortieth to one fourth of that used to prevent bleeding in patients with von Willebrand’s disease (vWD). The ease of intermittent dosing with DDAVP with low incidence of side effects must be balanced against the ability to titrate the continuous vasopressin infusion cited earlier. The long half-life of DDAVP (6 to 24 hours) in combination with intraoperative fluid administration may incur an increased chance of hyponatremia. In either case, careful monitoring of fluid balance is essential.
The anesthesiologist may occasionally encounter children who are receiving nightly nasal DDAVP for the treatment of enuresis. A review of its use reveals a negligible incidence of water intoxication (and no permanent effect on enuresis when treatment is stopped) (van Kerrebroeck, 2002). Given the known duration of action, DDAVP administered the night before outpatient surgery should not affect the urine output on the day of surgery.
Syndrome of Inappropriate Antidiuretic Hormone Secretion
Just as central DI is caused by ADH deficiency, syndrome of inappropriate ADH secretion (SIADH) is caused by an excess production of ADH that is inappropriate with respect to the state of the intravascular volume. The most common causes of SIADH are listed in Box 36-2. The hallmark of SIADH is hyponatremia in the face of high urine osmolality and sodium levels. A comparison of the urine and serum electrolyte status seen in DI and SIADH is presented in Table 36-4. The treatment for mild cases of SIADH is fluid restriction (50% to 60% of maintenance fluid requirement) or insensible loss (400 mL/m2 per day), plus one half to three fourths of the urine output. If hyponatremia is severe enough to cause coma or seizures, treatment with hypertonic saline (3%) solution may be indicated, but caution should be employed because the administration of hypertonic saline may cause circulatory overload because the intravascular volume is already increased. A too-rapid rise of osmolarity (more than 20 mOsm/kg or more than 10 mmol/L of sodium in 24 hours) carries a risk of central pontine myelinolysis, a condition that can result in death (Laureno and Karp, 1997). This syndrome is thought to be caused by the sudden shrinkage of brain cells in response to rapidly increasing extracellular osmolality.
Laboratory Test | Diabetes Insipidus | SIADH |
Urine specific gravity | ≤1.005 | ≥1.005 |
Urine osmolality | 50-200 mOsm/L | >200 mOsm/L |
Serum osmolality | >280 mOsm/L | <280 mOsm/L |
Serum sodium | High (usually >148 mEq/L) | Low (usually <132 mEq/L) |
Urine sodium | <20 mmol/L | >20 mmol/L |
Adrenal Insufficiency
Adrenal Insufficiency as a Result of Primary Abnormalities of the Hypothalamic-Pituitary-Adrenal Axis
Adrenal insufficiency is an uncommon disease in children, but when it occurs it is associated with significant implications for the anesthesiologist. The causes of adrenal insufficiency are listed in Box 36-3. Adrenal insufficiency may include glucocorticoid deficiency with or without mineralocorticoid deficiency (Box 36-4). Isolated hypoaldosteronism is rare. In the perioperative period, children with congenital adrenal insufficiency require glucocorticoid and mineralocorticoid replacement.
Box 36-3 Causes of Adrenal Insufficiency
Chronic deficits in adrenal function result in the classic findings of Addison disease, including hyperpigmentation, weakness, and hyponatremia. The hyperpigmentation results from high levels of adrenocorticotropic hormone (ACTH) and unopposed melanophore-stimulating hormone caused by cortisol insufficiency. The additional presence of aldosterone insufficiency may produce hyponatremia, hyperkalemia, hypotension, and a small cardiac silhouette that results from hypovolemia (Keon and Templeton, 1993).
Perioperative Steroid Management
The preoperative recognition of adrenal insufficiency and appropriate preoperative therapy minimize the likelihood of significant perioperative complications. Ninety percent of patients with congenital adrenal hyperplasia with adrenal insufficiency have 21-hydroxylase deficiency (Migeon and Donohoue, 1994). Virilization of the external genitalia occurs in female patients, and they often require surgical revision of their external genitalia. An abnormal genital pigmentation occurs in male patients, but this finding may be subtle. Infants with undiagnosed congenital adrenal hyperplasia may undergo exploratory laparotomy for acute abdomen because of nausea and vomiting. It is important to be attuned to the signs and symptoms in the history, physical examination, and laboratory evaluation that point to this diagnosis to prevent or treat shock, which may occur because of failure to administer steroid replacement.
Mineralocorticoid deficiency can be managed by administering saline solution and avoiding potassium in IV fluids. Mineralocorticoid secretion rates in children are similar to those in adults, and the replacement dose is independent of age and weight. Desoxycorticosterone acetate may be administered intramuscularly in a dose of 1 mg/day. The intramuscular injection may be replaced by a single daily oral dose of 9-fluorocortisol (Florinef, 0.05 to 0.1 mg) when it is clear that an oral medication can be tolerated and absorbed. When cortisol is administered perioperatively, it has sufficient mineralocorticoid activity to obviate the need for any other replacement; 20 mg of hydrocortisone has mineralocorticoid activity equivalent to 0.1 mg 9a-fluorocortisol (Miller et al., 2008).
Glucocorticoid deficiency is treated with cortisol (hydrocortisone) replacement. The importance of cortisol replacement for patients with known adrenal insufficiency should not be underestimated, although vastly excessive doses are unwarranted. In the normal individual, the adrenal gland secretes 12 ± 2 mg of cortisol per square meter of body surface area every 24 hours (Kenny and Preeyasombat, 1966). The normal replacement dose prescribed for unstressed children is 25 mg/m2 per day; the dose is double the normal production because of factors of bioavailability and half-life (Migeon and Donohoue, 1994). In response to stress (e.g., fever, acute illness, surgery, and anesthesia), the normal adrenal gland secretes 3 to 15 times this amount. Consequently, in the past, the recommendations for “stress” steroid coverage in the perioperative period ranged from 36 to 180 mg/m2 per day.
More important than just the dose of steroid to be given, consideration should be devoted to the type of glucocorticoid administered, its half-life, the route of administration, and the timing of doses. The equivalencies for steroid preparations in terms of their relative glucocorticoid and mineralocorticoid effects are presented in Table 36-5. The most commonly cited recommendation for perioperative steroid coverage is hydrocortisone hemisuccinate (Solu-Cortef), given intravenously as 2 mg/kg immediately preoperatively and every 6 hours on the day of surgery, with reductions in the postoperative period depending on the degree of stress. Some practitioners feel that the half-life of hydrocortisone is so short that a 6-hour dosing interval may lead to periods of inadequate “coverage.” These practitioners recommend a preinduction dose of 25 mg/m2 of hydrocortisone given intravenously, followed by a continuous infusion of 50 mg/m2 administered during the estimated period of anesthesia. Postoperatively, 50 mg/m2 by continuous infusion is administered over the remainder of the first 24 hours. The total dose for the first 24 hours is 125 mg/m2, or 10 times normal physiologic production (Migeon and Donohoue, 1994). The first bolus dose must be administered before induction of anesthesia rather than waiting for an IV cannula to be placed after inhalational induction because of the stress associated with anesthetic induction itself. In the postoperative period, the steroid dose is tapered to a level commensurate with the residual stress. It is replaced with the child’s usual oral preparation when the child clearly can tolerate and absorb oral medication.
Hypothalamic-Pituitary-Adrenal Axis Suppression Caused by Exogenous Steroid Therapy
In addition to the diseases discussed previously, suppression of the hypothalamic-pituitary-adrenal (HPA) axis can also occur after exogenous steroid usage, such as that administered for the treatment of inflammatory conditions (e.g., Crohn disease and asthma) or autoimmune disease (e.g., lupus and juvenile rheumatoid arthritis). Nearly 60 years ago, it was reported that two patients developed irreversible shock perioperatively after glucocorticoid administration was stopped preoperatively (Fraser et al., 1952; Lewis et al., 1953). Both patients were found to have adrenal atrophy and hemorrhage at autopsy. These two cases led to suggestions for “stress” steroid coverage in the perioperative period. HPA suppression places steroid-dependent children at increased risk for complications in the perioperative period, because they may be unable to respond to stress with an appropriate increase in the adrenal secretion of glucocorticoid. Dosages of cortisol or its equivalent that exceed 15 mg/m2 per day for more than 2 to 4 weeks invariably produce HPA suppression. A study in children with relatively short-term exposure to prednisolone or dexamethasone (5 and 3 weeks, respectively) for treatment of acute lymphoblastic leukemia showed that recovery of normal adrenal function (in response to ACTH stimulation) had a very wide range, occurring between 2 weeks and 8 months (Petersen et al., 2003).
Although high dosages, prolonged therapy, and short duration between discontinuance of therapy and the surgical procedure increase the likelihood of HPA suppression, no practical test is available that unequivocally identifies patients who will need intraoperative steroids. Metyrapone depresses the production of cortisol by the adrenal glands and can be used to test the capacity of the pituitary gland to respond to decreased plasma cortisol concentrations by increasing ACTH secretion (Haynes, 1990). However, this test takes 3 days, is expensive, and has the risk of inducing adrenal insufficiency. Similarly, an ACTH-stimulation test can be performed at great expense to test adrenal responsiveness. However, even if cost and time were not issues, a study has shown a poor correlation between tests that indicate normal HPA function and dose or duration of glucocorticoid therapy or basal cortisol levels (Schlaghecke et al., 1992). Clinically significant events rarely occur during the perioperative period in unsupplemented patients who were receiving steroid medications for diseases other than adrenal insufficiency. Nevertheless, the potential for symptomatic adrenal insufficiency, although rare, coupled with the low risk of steroid-induced complications for short-term administration, suggests that steroids should be given in uncertain cases. If steroid therapy has been discontinued within the previous year, Donohoue (2005) makes the following recommendations:
HPA suppression also can result from modes of steroid administration other than oral, including topical, nasal spray, and inhalers. Although adrenal suppression is rarely symptomatic with these modes of administration, some drugs, especially fluticasone propionate, in high doses have been associated with growth failure and adrenal suppression (Duplantier et al., 1998). With surgical stress, patients with adrenal suppression may become symptomatic, as has been reported for other kinds of stress. The patients reported by Drake et al. (2002) all had been taking fluticasone and had hypoglycemia at times of stress from intercurrent illness. Numerous cases of acute adrenal crisis have been reported in children receiving inhaled corticosteroids (ICS) for prolonged periods (Randell et al., 2003). Anesthesiologists should have a high index of suspicion of adrenal suppression if an asthmatic child on inhaled steroids develops hypotension or hypoglycemia in the perioperative period.
The dose administered should also be proportional to the perceived degree of surgical stress. For brief procedures, such as upper endoscopy, a single preoperative dose of steroids is suggested (50 mg/m2 of hydrocortisone); for more complicated cases, such as appendectomy or major intraabdominal operations, 100 mg/m2 is administered as a continuous infusion or divided into 4 doses per day. This dose is usually continued for 1 to 3 days after more complex surgical procedures (Krasner, 1999). The dose is tapered postoperatively and replaced with the patient’s usual oral steroid preparation and dose when the child is able to tolerate oral medications. The dose that these patients commonly take for the underlying disease often exceeds even maximum “stress” doses described for congenitally adrenal insufficient patients, and treatment required for the underlying disease may limit further tapering of the steroid dose. A small study of adults comparing “stress steroids” with saline showed no adverse effects in patients who continued their usual steroid dose for their underlying disease (Glowniak and Loriaux, 1997).
Thyroid Disorders
Hypothyroidism
Hypothyroidism occurs because of abnormally low production of thyroid hormone. It may be caused by primary thyroid dysfunction or result from pituitary failure with decreased production of thyroid-stimulating hormone (TSH). Normal values for routinely performed thyroid function tests are presented in Table 36-6, and the interpretation of these test results with regard to diagnosis is presented in Table 36-7.
Test | Age | Normal |
T4 RIA (mcg/dL) | 1-3 days | 11-21.5 |
1-4 weeks | 8.2-16.6 | |
1-12 months | 7.2-15.6 | |
1-5 years | 7.3-15 | |
6-10 years | 6.4-13.3 | |
11-15 years | 5.6-11.7 | |
16-20 years | 4.2-11.8 | |
T3 RU | 25%-35%* | |
T index | 1.25-4.20† | |
Free T4 (ng/dL) | 1-10 days | 0.6-2 |
>10 days | 0.7-1.7 | |
T3 RIA (ng/dL) | 1-3 days | 100-380 |
1-4 weeks | 99-310 | |
1-12 months | 102-264 | |
1-5 years | 105-269 | |
6-10 years | 94-241 | |
11-15 years | 83-213 | |
16-20 years | 80-210 | |
TSH RIA (mIU/mL) | 1-3 days | <2.5-13.3 |
1-4 weeks | 0.6-10 | |
1 month-15 years | 0.6-6.3 | |
16-20 years | 0.2-7.6 | |
TBG (mg/dL) | 1-3 days | — |
1-4 weeks | 0.5-4.5 | |
1-12 months | 1.6-3.6 | |
1-5 years | 1.3-2.8 | |
6-20 years | 1.4-2.6 | |
Reverse T3‡ (mg/dL) | Newborns | 90-250 |
Adults | 10-50 |
RIA, Radioimmunoassay; RU, resin uptake; T3, triiodothyronine; T4, thyroxine; TBG, thyroid-binding globulin; TSH, thyroid-stimulating hormone.
* Measures thyroid hormone binding, not T3.
Modified from Johnson KB, editor: The Harriet Lane Handbook, St. Louis, 1993, Mosby.
Primary thyroid dysfunction may be congenital or acquired. Congenital hypothyroidism usually appears in infancy. Classic features in the infant include large fontanels, wide sutures, large tongue, umbilical hernia, and decreased deep tendon reflexes. In the older child, manifestations include slow heart rate, narrow pulse pressure, growth failure, hypothermia, and cold intolerance. Severe hypothyroidism is rare but may be associated with coma, cardiovascular collapse, hyponatremia, hypothermia, and respiratory failure. Keon and Templeton (1993) reviewed the anesthetic management of patients with hypothyroidism and stressed the importance of correcting hypothyroidism gradually over a 2-week period. Sudden death has been reported in children with myxedematous heart disease 2 to 3 weeks into therapy (LaFranchi, 1979). It is suggested that these children receive one fourth of the maintenance dose of thyroid hormone (6 to 8 mcg/kg per day for an infant), with gradual incremental increases over 2 to 4 weeks until a maintenance dose is reached (Custer and Rau, 2009). Patients who are adequately treated will have normal thyroid hormone and TSH levels. Patients who do not respond to oral thyroid replacement may be given IV triiodothyronine (T3; loading dose of 0.7 mcg/kg), followed by an infusion titrated to T3 and TSH levels. Severe cardiac dysfunction in such a patient improved with T3 therapy (Mason et al., 2001). Patients with incompletely restored thyroid hormone levels may require hemodynamic monitoring and support to maintain hemodynamic stability. Patients with severe hypothyroidism may have associated adrenal insufficiency, and if so, they should receive stress steroid coverage as outlined earlier.
The anesthetic care of the symptomatic patient with hypothyroidism can be problematic and requires caution when any depressant medications are given. Prolonged effects may result from decreased drug metabolism. Important considerations in the management of hypothyroidism as described by Keon and Templeton (1993) are outlined in Table 36-8. Invasive monitoring may be indicated when significant blood loss or fluid shifts occur. Care should be taken intraoperatively to minimize heat loss. Postoperative care should include monitoring of oxygen saturation, blood pressure, heart rate, and respiratory rate; postoperative ventilation may be necessary in the patient with delayed emergence from anesthesia.
TABLE 36-8 Anesthetic Implications of Hypothyroidism
System | Anesthetic Considerations |
Pharmacologic | Possible lower MAC value; prolonged recovery from opioid anesthesia |
Cardiovascular | Decreased cardiac output, heart rate, and stroke volume; increased PVR and decreased intravascular volume; myocardial depression resulting from impaired cellular metabolism or myxedematous infiltration; baroreceptor dysfunction |
Respiratory | Abnormal response to hypercapnia and hypoxia |
Thermal regulation | Hypothermia resulting from reduced basal metabolic rate; reduced ability to increase core temperature |
Endocrine | Increased incidence of adrenal insufficiency; consideration for stress steroid coverage |
Metabolic | SIADH; hypoglycemia with prolonged fasting |
Gastrointestinal | Delayed gastric emptying; consideration for full stomach precaution |
MAC, Minimum alveolar concentration; PVR, peripheral vascular resistance; SIADH, syndrome of inappropriate antidiuretic hormone.
Hyperthyroidism
Hyperthyroidism is a syndrome produced by excess levels of circulating thyroid hormone. The most common causes are congenital hyperthyroidism and Graves disease (i.e., toxic goiter). Less commonly, acute suppurative thyroiditis, hyperfunctioning thyroid carcinoma, thyrotoxicosis factitia (i.e., exogenous administration of thyroid hormone), and toxic uninodular goiter (i.e., Plummer disease) may produce this syndrome. McCune-Albright syndrome (i.e., precocious puberty with polyostotic fibrous dysplasia) is also commonly associated with hyperthyroidism (Jones, 1988).
Congenital Hyperthyroidism
Congenital hyperthyroidism is a transient phenomenon seen in newborns that results from the transplacental transfer of thyroid-stimulating antibody from mothers who commonly have a history of Graves disease. Most of these infants have a goiter and typically appear anxious and restless or irritable. Signs of hypermetabolism, including tachycardia, tachypnea, and elevated temperature, may be present. In the severely affected infant, symptoms may progress to weight loss, severe hypertension, and high-output cardiac failure with hepatomegaly (Smith et al., 2001). Appropriate medical therapy (methimazole) should be instituted early. Because maternal immunoglobulins have a short half-life in infants, the hyperthyroid state resolves in a few weeks to a few months, and it sometimes may be followed by a period of hypothyroidism (Higuchi et al., 2001).
Thyroid Storm
An acute onset of hyperthermia, severe tachycardia, and restlessness comprises the syndrome of acute uncompensated thyrotoxicosis, or “thyroid storm.” Without appropriate and timely therapy, the patient’s condition may deteriorate to delirium, coma, and death. Therapy includes treatment of hyperthermia by cooling, maintenance of intravascular volume with balanced salt solutions, and β-adrenergic blockers such as propranolol titrated to ameliorate the cardiovascular response. Specific thyroid suppression therapy with propylthiouracil should be instituted. The clinical presentation of thyroid storm may occur intraoperatively, and this hypermetabolic state may be mistaken for malignant hyperthermia (Peters et al., 1981). The use of dantrolene mitigated the clinical signs in a patient who turned out to have thyroid storm (Bennett and Wainwright, 1989). It is well known that perioperative surgical stress can trigger the development of thyroid storm in a patient with previously unrecognized thyrotoxicosis (Stevens, 1983). For this reason, patients with signs and symptoms that may indicate the presence of hyperthyroidism should be carefully evaluated. Patients should be rendered euthyroid before any elective surgery, even if it is minor.
Treatment
The management of hyperthyroidism is aimed at controlling the cardiovascular effects. β-adrenergic receptor blockade, usually with propranolol (1 to 2 mg/kg per day), is titrated to effect. Antithyroid medications include propylthiouracil and methimazole, both of which inhibit the incorporation of inorganic iodide into organic compounds. Propylthiouracil inhibits the conversion of T4 to T3. Although early studies suggested that these agents might inhibit the formation of thyroid antibodies, later studies that included careful histopathologic analysis have shown this to be false (Paschke et al., 1995). The Food and Drug Administration (FDA) has recently recommended that propylthiouracil not be administered to pediatric patients because of reports of liver failure associated with its use. Methimazole is the antithyroid medication recommended for use in infants, children, and adolescents (FDA, 2009). Saturated solutions of potassium iodide may be administered orally (1 drop every 8 hours) to suppress thyroid hormone secretion. The clinical response to therapy is evident in 1 to 3 weeks, and the patient may require up to 3 months for adequate control to be achieved. Patients must have appropriate, regular surveillance to ensure that the T3 and T4 levels are in the normal range and that TSH concentrations are normal. Clinically, the patient demonstrates a euthyroid state by return of the heart rate, blood pressure, and reflexes to normal.
Postoperative Care
Children who have undergone thyroidectomy require close observation in the postoperative period (Fewins et al., 2003). They may develop postextubation croup or upper airway obstruction as a result of paralysis of the vocal cords, tetany (hypocalcemia), residual tracheomalacia, or tracheal compression resulting from a hematoma. Patients with postextubation croup may respond to supportive measures, including humidified supplemental oxygen, nebulized racemic epinephrine, and possibly, continuous positive airway pressure (CPAP) or bilevel positive airway pressure (BiPAP). Occasionally, these patients require brief reintubation before they can be successfully extubated. Unilateral vocal cord paralysis may go unnoticed or be associated with only mild stridor. Bilateral vocal cord paralysis, on the other hand, may manifest as severe stridor and upper airway obstruction. The child with bilateral vocal cord paralysis requires reintubation for airway support. A muscle relaxant such as rocuronium should be used to facilitate reintubation to avoid damage to the abducted cords. If the paralysis is prolonged, the child may subsequently require tracheostomy. Compression of the trachea by a hematoma may occur immediately after the operation or over the course of several hours. The child requires reintubation and surgical evacuation of the hematoma to relieve tracheal compression. Opening the wound in the recovery room may be necessary and lifesaving. After the extrinsic obstruction has been relieved and the incision closed again (if necessary), the child may be safely extubated.
Pheochromocytoma
Pheochromocytoma is a catecholamine-secreting tumor of chromaffin cells that most commonly arises in the adrenal medulla (DiGeorge, 1987). It may be found anywhere along the abdominal sympathetic chain, but it is most commonly near the aorta at the inferior mesenteric artery or the aortic bifurcation. Other sites include the neck, the mediastinum, and the walls of the bladder or ureters. Pheochromocytoma is a rare neoplasm in the pediatric population. Fewer than 5% of reported cases occur in children. The tumors may occur bilaterally or at multiple sites. This condition can be inherited as an autosomal-dominant trait (most often in association with von Hippel–Lindau syndrome) or as part of a multiple endocrine neoplasia (MEN) type II or III (Table 36-9).
MEN Syndrome | Affected Organs | Disorder |
Werner syndrome (type I, familial) | Parathyroid gland; pancreas; pituitary gland | Hypercalcemia; hypoglycemia; peptic ulcer |
Sipple syndrome (type II, autosomal dominant) | Thyroid and parathyroid glands; adrenal medulla | Medullary carcinoma; hypercalcemia; pheochromocytoma |
Type III | Nervous system; thyroid gland; adrenal medulla | Multiple neuromas; medullary carcinoma; pheochromocytoma |
MEN, Multiple endocrine neoplasia.
Diagnosis
It is extremely important to establish the diagnosis of pheochromocytoma before induction of anesthesia and start of surgery. The significant cardiovascular effects of excess catecholamines can pose difficulties for the anesthesiologist and endanger the patient during the perioperative period if an appropriate diagnosis is not made preoperatively. These tumors can produce paroxysms of hypertension and other symptoms. Between paroxysms, the patient may be totally asymptomatic, making diagnosis extremely difficult. The demonstration of increased levels of catecholamines is the most specific diagnostic test. Although pheochromocytomas can produce norepinephrine and epinephrine, the predominant catecholamine produced in children is norepinephrine, which leads to chronic hypertension. Urine catecholamine concentrations are directly proportional to circulating levels, and determination of 24-hour urinary excretion of the primary catecholamines and their metabolites (i.e., 3-methoxy 4-hydroxy vanillyl-mandelic acid [VMA] and metanephrine) used to be the primary means of establishing the diagnosis. The plasma free metanephrine determination has better sensitivity (100%) and specificity (94%). Because normal values differ with age, it is important to use age-specific norms when interpreting results (Weise et al., 2002).
Preoperative Preparation and Evaluation
Symptomatic treatment includes the administration of phenoxybenzamine over a period of several days to weeks before surgery. Phenoxybenzamine is a long-acting, orally administered α-adrenergic blocking agent that attenuates the effects of catecholamines on the peripheral circulation by blocking excessive vasoconstriction (Hoffman and Lefkowitz, 1990). The starting dose of phenoxybenzamine is 0.2 mg/kg once daily (maximum adult dose, 10 mg) (Taketomo et al., 2008). Then both dose and frequency are gradually increased (up to 3 times a day) until a clinical effect is obtained; that is, the patient’s hematocrit decreases (because of vasodilation and increased blood volume), and the patient develops orthostatic changes in vital signs. Long-standing vasoconstriction produced by chronically high catecholamine levels causes decreased intravascular volume. Although the use of phenoxybenzamine restores vascular capacity to normal, increased oral fluid intake should accompany administration of phenoxybenzamine to avoid severe orthostatic changes. In some children, β-adrenergic blocking drugs such as propranolol may be needed to control heart rate and blood pressure. However, β-blocking agents should never be used without concurrent α-blockade therapy because of the deleterious effects of unopposed α-agonism, which may result in cardiac failure as a result of increased afterload. Labetalol may have a role in the management of pheochromocytoma, because it has α- and β-adrenergic blocking properties (Blom et al., 1987). It can be useful in minimizing the cardiovascular effects of excess catecholamines during the perioperative period, but it is not as potent an a blocker as is phenoxybenzamine for preoperative treatment.
Anesthetic Induction
Induction of anesthesia is accomplished with IV anesthetics. Ketamine is specifically contraindicated, because it induces catecholamine release. Halothane should be avoided, because it may sensitize the myocardium to catecholamines and produce dysrhythmias. Mask induction with sevoflurane is well tolerated if hemodynamic parameters are well controlled, and IV cannula placement can be deferred until after induction. Intubation may proceed in the usual fashion, facilitated by a hemodynamically neutral nondepolarizing muscle relaxant such as vecuronium. Pancuronium, which causes muscarinic blockade and tachycardia, should be avoided. Despite the fact that atracurium causes histamine release and vasodilation, it has been used safely in adult patients with pheochromocytoma (Prys-Roberts, 2000). Before intubation, IV lidocaine (1 mg/kg), fentanyl (2 to 5 mcg/kg), or both are effective in minimizing the hemodynamic response to intubation.
Intraoperative Management
Anesthesia may be maintained with isoflurane or sevoflurane, air, and oxygen. Both anesthetic agents have been used without exacerbation of hypertension, despite the fact that they do not blunt the production of norepinephrine in response to surgical stimulation (Suzukawa et al., 1983). Desflurane should be avoided because of its tendency to cause tachycardia and hypertension. The addition of moderately large doses of fentanyl (10 mcg/kg) or remifentanil (0.3 to 1 mcg/kg per minute) minimizes the stress response and provides stable hemodynamics. If remifentanil is chosen, it is important to give a longer-acting opioid before the end of surgery to avoid hypertension as a result of pain on awakening. Adjunctive use of epidural anesthesia (i.e., local anesthetic with or without a small dose of fentanyl) is an excellent method of reducing the stress response and catecholamine release caused by usual surgical stimulation. However, none of these anesthetic strategies blocks catecholamine release that results from direct surgical manipulation of tumor tissue.
Historically, controlling blood pressure during the induction and maintenance of anesthesia has been accomplished by an infusion of sodium nitroprusside or phentolamine. However, resection is increasingly being performed using a laparoscopic technique, and either nicardipine or magnesium sulfate infusions are used to maintain vasodilation and normotension during this type of surgery (Pretorius et al., 1998; Minami et al., 2002). In addition to propranolol use preoperatively, esmolol has been effective as a continuous infusion titrated to the level of surgical stimulation (Nicholas et al., 1988). Infusions of only short-acting vasodilators are recommended for control of hypertension before tumor resection, because with removal of the tumor, vasodilation caused by a persistent blockade and loss of excess catecholamines may lead to precipitous hypotension. Phenoxybenzamine has a long half-life; therefore, some physicians recommend discontinuing it 24 hours before surgery to decrease the likelihood that persistent vasodilation caused by a blockade will cause severe hypotension after the tumor is removed because of withdrawal of the catecholamines of tumor origin (Prys-Roberts, 2000). Hypotension is best treated with discontinuation of vasodilator infusions, titration of anesthetic agents, and administration of crystalloid or colloid and blood products, if it is indicated by the magnitude of blood loss. If these measures are ineffective, vasopressors may be necessary, but the patient may be relatively resistant to α-agonists as a result of persistent α-blockade. If this occurs, judicious use of small doses of more potent, direct-acting vasoconstrictors (e.g., norepinephrine or epinephrine) may be necessary. Recently, vasopressin infusion has been effective in this situation (Deutsch and Tobias, 2006).
Respiratory Disorders
Upper Respiratory Tract Infection
Viral upper respiratory tract infections (URIs) are mild processes that do not preclude school attendance and other routine activities. However, URIs hold much greater significance for anesthesiologists. For many anesthesiologists, it is standard practice to avoid general anesthesia for elective surgery in children with URIs because of the respiratory complications during and after anesthesia reported in multiple small case series (McGill et al., 1979; Cohen and Cameron, 1991; Konarzewski et al., 1992; Williams et al., 1992). Unfortunately, the vexing problem of runny noses in children is accentuated by the difficulty in differentiating URIs from other causes, such as allergic rhinitis, which does not increase the risk of complications.
Pathophysiology of Upper Tract Respiratory Infection
Many investigators suggest that complications, including bronchospasm, intraoperative hypoxemia with an increased alveolar-arterial oxygen gradient, and postoperative hypoxemia, occur more often in children who undergo anesthesia while they have URIs (McGill et al., 1979; Olsson, 1987; DeSoto et al., 1988). The proclivity for these complications may be related to peripheral airway abnormalities, which have been demonstrated experimentally in adult humans and animals infected with viral respiratory pathogens (Johanson et al., 1969; Fridy et al., 1974; Dueck et al., 1991). These abnormalities include decreased diffusing capacity and increased closing volume—factors that can predispose patients to intrapulmonary shunting and hypoxemia, especially when they are combined with the effect of general anesthesia on lung volumes (decreased functional residual capacity) (see Chapter 3, Respiratory Physiology in Infants and Children) (Murat et al., 1985). These studies were done in adults who had infections involving their entire respiratory tracts rather than isolated URIs, and their results may support separation of treatment of patients with truly isolated URIs from those with any symptoms of more global airway or pulmonary parenchymal involvement (lower respiratory tract infection). Although the mechanisms by which viral respiratory infections lead to alterations in airway function are unclear, these experimental studies support the clinical impression that increased risk of perioperative hypoxemia occurs in patients with recent viral respiratory infection.
Empey et al. (1976) demonstrated in adult patients that acute viral respiratory tract infection (influenza) produced marked bronchial reactivity to experimental bronchoconstrictor challenge that may persist for 6 weeks. Mechanisms by which viral infections lead to increased airway reactivity include the release of immunologic and inflammatory mediators such as leukotrienes, bradykinin, and histamine, which cause bronchoconstriction. Vagal-mediated mechanisms may be involved, because viral infections have been associated with changes in muscarinic receptors on airway smooth muscle (Fryer et al., 1990). Tissue concentrations of important enzymes such as neutral endopeptidase, which break down the neuropeptides that cause bronchoconstriction, are also decreased in viral infections (Jacoby et al., 1988; Dusser et al., 1989). However, these patients and animals cannot be said to have only URIs, because the airways below the larynx are also clearly affected. Patients whose infections are truly uncomplicated URIs or those with noninfectious causes of runny nose should be differentiated from those who have evidence of lower respiratory involvement.
Perioperative Risk
Many case reports in the literature document that in the perioperative period children with URIs have respiratory complications, including bronchospasm, stridor caused by subglottic edema, hypoxia, and atelectasis (McGill et al., 1979; Konarzewski et al., 1992; Williams et al., 1992). Three prospective studies have shown that patients with an active or recent URI had a 2- to 10-fold higher risk of bronchospasm or laryngospasm (Olsson and Hallen, 1984; Olsson, 1987; Cohen and Cameron, 1991). The incidence was higher among younger children, especially those younger than 2 years and those whose tracheas were intubated (Cohen and Cameron, 1991). Retrospective studies of much larger numbers of patients show a higher risk of respiratory complications may actually exist in asymptomatic children with history of URI within the 2 to 4 weeks preceding surgery than in those with acute URI (Tait and Knight, 1987b; Tait et al., 2001). Other studies found that the factors that increase the risk of adverse events were history of prematurity or reactive airways disease (RAD), parental smoking, copious secretions, nasal congestion intubation, and airway surgery (Tait et al., 2000, 2001). Despite this increased risk of adverse events, complications usually were easily treated and were not associated with any significant prolonged morbidity (Rolf and Cote, 1992; Tait et al., 2000, 2001). However, some patients in one study developed atelectasis severe enough to require bronchoscopy and prolonged postoperative mechanical ventilation. Although most of these studies evaluated children undergoing relatively minor elective surgery, another study reported that children with URI symptoms at the time of cardiac surgery also had increased risks for respiratory and other complications, including nonrespiratory infection (Malviya et al., 2003). Despite these findings, patients’ hospital stays were not prolonged, and the incidence of long-term sequelae was not increased.
One study found that children with URIs who undergo mask halothane-nitrous oxide-oxygen anesthesia for myringotomy surgery had reduced severity and duration of URI symptoms in the postoperative period (Tait and Knight, 1987a). However, this reduction in symptoms may have resulted from the drainage and removal of infectious foci by the surgical procedure rather than from the beneficial effects of general anesthetics. Other investigators have reported no significant respiratory complications when children with URI were anesthetized (Hinkle, 1989; Jacoby and Hirshman, 1991). It is extraordinarily difficult to integrate the contradictory conclusions of these various series of patients to develop a logical algorithm for dealing with the child with a URI.
Anesthetic Decision Making
The most conservative approach to the child with a URI or recent URI is to postpone elective procedures for 1 to 2 weeks for uncomplicated rhinorrhea, congestion, and nonproductive cough and for 4 to 6 weeks for patients with lower-airway involvement (e.g., wheezing or productive cough). However, this may be an overly cautious and somewhat unrealistic recommendation. Children without other health conditions have an average of 3 to 8 colds per year, and children whose mothers smoke, who live in crowded conditions, and who attend daycare centers have a 61% incidence of URIs over a 2-week period (Fig. 36-2) (Fleming et al., 1987). It may be nearly impossible to find a time when the child does not have a URI or is not recovering from one. The needs of the family must be considered. Often, parents have traveled significant distances, taken time off from work, and made alternative childcare arrangements for their other children. Because the available data do not clearly indicate a single best approach to these patients, each anesthesiologist should develop a consistent approach appropriate to the individual practice.
Elective surgeries other than those cited previously are postponed if any of the following are present: “croupy” cough; rectal temperature higher than 38.3° C associated with any URI sign or symptom; malaise or decreased appetite; and any evidence or recent history of lower respiratory tract involvement such as rales, wheezes, productive cough, or abnormal chest radiograph (Box 36-5). Laboratory and radiographic tests are usually not helpful in the decision-making process, although some investigators recommend obtaining a chest radiograph and a white blood cell count to evaluate the child with a URI. The white blood cell count is neither sensitive nor specific in identifying a URI, and chest radiography associated with a normal auscultative examination is unlikely to identify abnormalities (Brill et al., 1973). The presence of rales or wheezes should lead to postponement of elective surgery, regardless of findings on the chest radiograph. A suggested algorithm for making decisions about proceeding with surgery is presented in Figure 36-3.
Anesthetic Management
Several major principles of anesthetic management can be suggested for dealing with children with acute or recent URIs (Tait and Malviya, 2005). These are especially important in anesthetizing children when surgery is urgent and cannot be delayed. In elective situations, it is best to avoid intubation if possible (if the surgical procedure allows), instead using regional anesthesia or general anesthesia by mask or laryngeal mask airway (LMA). A randomized, prospective study demonstrated a much lower incidence of bronchospasm in children with URI managed with LMA rather than ETT (0% vs. 12.2%) (Tait et al., 1998). The incidence of all respiratory complications was reduced by 50% for the LMA group (19% vs. 35%). LMA may be an excellent alternative for airway management for patients with URI if the planned surgical procedure and fasting status are compatible with its use. If intubation is indicated, it should be accomplished when the patient is at a deep plane of anesthesia using an ETT at least 1 size (0.5 cm) smaller than age would determine. Any IV induction agent is acceptable, with the most important guiding principle being that enough should be given to achieve a deep level of anesthesia. An alternative is mask induction of inhalation anesthesia with sevoflurane, with or without nitrous oxide, and oxygen. If the procedure is expected to be prolonged, heated humidification should be used, because use of dry gas may lead to inspissation of secretions.
Adjunctive agents, such as IV lidocaine (1 mg/kg), an opioid, or both, decrease airway reflexes (Hirshman, 1983). The preoperative use of anticholinergic agents (i.e., atropine and glycopyrrolate) theoretically may block muscarinic receptors, thereby interrupting the airway reflex arc (Jacoby and Hirshman, 1991). Their properties as antisialogogues may be helpful; however, these agents have not been shown to be beneficial in prospective studies (Tait et al., 2007). The use of glucocorticoids experimentally has decreased viral-associated, tachykinin-induced airway edema formation, although glucocorticoids are not routinely prescribed in this clinical situation—in contrast to their use in the patient with RAD (Piedimonte et al., 1990). Dexamethasone, however, has been used in an effort to prevent postextubation croup, but these studies have been performed in critically ill children who had undergone prolonged intubation and mechanical ventilation in the intensive care unit, rather than in children with respiratory infections who are undergoing surgery. In this high-risk population, dexamethasone may be effective (Markovitz and Randolph 2002; Lukkassen et al., 2006). If intubation is necessary, tracheal suction of URI-associated secretions after intubation and before extubation may decrease the chance of atelectasis and mucus plugging, although this hypothesis has not been studied.
Management of children with URI requires a logical approach. When this issue arises in the preoperative period, the patient, parents, surgeon, and anesthesiologist must participate in an informed fashion in the decision-making process; however, “in the final analysis, the name of the game is clinical judgment and a degree of good fortune” (Berry, 1990).
Reactive Airways Disease (Asthma)
RAD, or asthma, the most common chronic disease of childhood in industrialized countries, has received wide public attention in recent years because of increases in morbidity and mortality. Asthma is the major cause of restricted activity, absence from school, and hospital admission in children, and it is responsible for significant health care costs in the United States (Newacheck and Halfon, 2000). The prevalence of asthma among children is greater than among adults, and it has increased by an average of 4.3% each year between 1980 and 1996 (Akinbami and Schoendorf, 2002). Beginning in 1997, the survey questions used to determine the prevalence of asthma were changed, which resulted in a slightly lower prevalence than in previous years, but the increasing trend has remained constant (MMWR, 2000).
Etiologic Factors and Pathophysiology
Asthma is a chronic inflammatory disorder of medium and small airways in which many cell types play a role, including mast cells and eosinophils. These cells release mediators of inflammation that, in susceptible individuals, cause symptoms associated with variable airway obstruction and airway hyperreactivity that is partially or completely reversible spontaneously or with appropriate treatment. Understanding the important role of inflammation in the immunopathogenesis of asthma in recent years has changed the focus to a newer therapeutic approach using antiinflammatory agents. Among the immune regulatory pathways involved in the pathogenesis of asthma, 2 cytokines—interleukin-4 and interferon-γ—appear to be important in controlling immunoglobin E (IgE) production, which is critical in the allergic inflammatory process. In individuals with asthma, mast cells and eosinophils are attracted to airways and release cytokines and lipid mediators that cause inflammation (Goldstein et al., 1994). The interplay of allergens and irritants, mast cells, eosinophils and their mediators, and the end effects on pulmonary vessels and airways is depicted in Figure 36-4. These mediators include histamines, leukotrienes, prostaglandins, kinins, and cytokines. Airway obstruction in asthma results from a combination of several factors, including airway smooth muscle spasm, airway mucosal edema, hypersecretion, and mucus plugging of small bronchi and bronchioles (Djukanovic et al., 1990). These changes result in airway obstruction, increased work of breathing, uneven distribution of ventilation, and in severe disease, air trapping, hyperinflation, and ventilation-perfusion imbalance, which leads to hypoxemia, diaphragmatic fatigue, hypercapnia, and respiratory failure. In longstanding RAD, mast cells infiltrate airway smooth muscle and in combination with chronic inflammation may result in airway remodeling, potentiating bronchoconstriction and airway hyperreactivity (Brightling et al., 2002).
A strong association exists between asthma and allergy. Up to 90% of children with recurrent wheezing respond positively to bronchoconstrictor challenge, especially when associated with atopy (Clough et al., 1991). An increased prevalence of asthma is reported among first-degree relatives of asthmatic subjects; over two thirds of children with asthma appear to have a familial predisposition (Clifford et al., 1989). Various environmental factors precipitate airway hyperreactivity and trigger asthma. Recent evidence has shown that obesity is associated with a proinflammatory state and is an independent risk factor for the development of asthma (Visser et al., 2001; Guerra et al., 2004).
The onset of asthmatic symptoms is often associated with viral infection of the lower respiratory tract, particularly respiratory syncytial virus (RSV) infection in infants and children (Rooney and Williams, 1971). Severe viral bronchiolitis in infancy is significantly associated with the subsequent development of airway hyperreactivity and asthma, although familial factors cannot be ruled out (Gurwitz et al., 1981; Gern et al., 2005; Lemanske et al., 2005). The development of IgE antibody to RSV may have an important role in inducing an allergic response to the virus (Welliver et al., 1989; Rakes et al., 1999). Children who experience respiratory failure and mechanical ventilation during infancy and early childhood, such as those with bronchopulmonary dysplasia (BPD), neonatal repair of congenital diaphragmatic hernia, or severe viral bronchiolitis, develop and sustain airway hyperreactivity even without a family history of asthma (Mallory et al., 1989; Nakayama et al., 1991). Prematurity alone may be associated with a higher incidence of asthma in preadolescent children (von Mutius et al., 1993). The primary site of airway obstruction and hyperreactivity in children with a history of neonatal respiratory failure appears to be in relatively small airways—in contrast to relatively large central airway obstruction and hyperreactivity in those with typical allergic (i.e., IgE antibody-mediated) asthma (Mallory et al., 1991). In these patients, airway spasm is characterized by a rapid drop in oxygen saturation of Hb (SpO2) without audible wheezing by auscultation.
In children with RAD, parental smoking (passive smoking) increases the severity of symptoms and exacerbates airway hyperreactivity (Soussan et al., 2003). Intrauterine exposure to maternal smoking also increases the incidence of airway hyperresponsiveness in infants (Singh et al., 2003). Infants with gastroesophageal reflux and chronic esophagitis often develop airway hyperresponsiveness with or without chronic aspiration and resultant tracheobronchial inflammation (Sheikh et al., 1999). Contributing factors responsible for the development of airway hyperreactivity and asthma are listed in Figure 36-5.
Precipitating Factors for Reactive Airways Disease
Viral lower respiratory infections, particularly those caused by RSV and influenza, sensitize airways and provoke airway hyperreactivity even in individuals who are nonasthmatic and nonallergic for as long as 6 weeks (Empey et al., 1976). Exposure to dry, cold air can precipitate tracheobronchial constriction in subjects with asthma, presumably in response to reduced tracheal temperature caused by evaporative heat loss (Gilbert et al., 1988). The same mechanism appears to be responsible for exercise-induced bronchospasm and precipitation of asthma with excitement, anxiety, and hyperventilation (McFadden and Gilbert, 1994).
The time before and during the induction of anesthesia is uniquely suited to trigger bronchospasm in susceptible individuals because of the patient’s emotional stress, fear, and excitement. Hyperventilation results, with mouth breathing of dry anesthetic gas mixtures, airway irritation by volatile anesthetics, and mechanical stimulation of the pharyngeal and laryngeal mucosa by laryngoscopy and endotracheal intubation (Box 36-6).
Pharmacologic Agents for Asthma
The pharmacologic management of asthma consists of bronchodilators and antiinflammatory drugs and includes six different classes of drugs: corticosteroids, leukotriene inhibitors, β-adrenergic agonists, theophylline, cromolyn (or nedocromil), and anticholinergics. Recently published guidelines for treatment of asthma in children include a useful algorithm (Fig. 36-6) (Bacharier et al., 2008). Initial treatment for asthma most commonly consists of inhaled corticosteroids (ICSs) and leukotriene-receptor antagonists, with ICSs usually being the first-line drugs. Montelukast has been used widely in children. Other first-line alternatives are cromolyn (or nedocromil). Because of safety concerns, long-acting β2-agonists such as salmeterol are now reserved for patients with poor control despite use of an ICS and leukotriene-receptor antagonists (Martinez, 2005). Theophylline has reentered the treatment algorithm if patients have exacerbations despite ICS and β-adrenergic agonists. Oral corticosteroids in brief high-dose pulses are reserved for patients with moderate to severe asthma that is unresponsive to combinations of the other drugs (Stempel, 2003). Drugs commonly used in children are listed in Table 36-10.
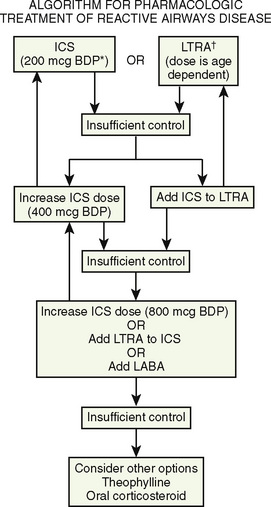
FIGURE 36-6 Algorithm for treatment of RAD. Once control is achieved, therapy may be stepped down to previous level. ICS, Inhaled corticosteroid; BDP, beclomethasone equivalent; LTRA, leukotriene receptor antagonist, for dose, see Table 36-10.
(Modified from Bacharier LB, et al: Diagnosis and treatment of asthma in childhood: a PRACTALL consensus report, Allergy 63:5, 2008.)
Drug | Formulation | Dosage |
Antiinflammatory Drugs | ||
Inhaled Corticosteroids | ||
Beclomethasone dipropionate (QVAR) | MDI, 40, 80 mcg/puff | 2-4 puffs bid (5-11 yr) |
Budesonide (Pulmicort) | DPI, 90, 180 mcg/inhalation | 1-2 inhalations bid (6-17 yr) |
Flunisolide (AeroBid) | MDI, 250 mcg/puff | 2 puffs bid (6-15 yr) |
Fluticasone proprionate (Flovent) | MDI, 44, 110, or 220 mcg/puff | 1-2 puffs bid (max, 440 mcg/d) (4-11yr) |
Fluticasone proprionate (Flovent Diskus) | DPI, 50 mcg/inhalation | 1-2 inhalations bid (4-11 yr) |
Triamcinolone acetonide (Azmacort) | MDI, 75 mcg/inhalation | 2-4 inhalations bid (6-12 yr) |
Oral Corticosteroids | ||
Prednisone/prednisolone | Oral tablets (1, 2.5, 5, 10, 20 mg) | Acute: 1 mg/kg q d/bid × 5-14 d |
Prednisone/prednisolone (Prelone, Pediapred) | Oral liquid (1 mg/mL) | Chronic: 0.25-2 mg/kg qodPreoperative: 1 mg/kg/d × 3 dMax, 60 mg/d |
Leukotriene Receptor Antagonist | ||
Montelukast (Singulair) | Oral granules, 4 mg; chewable tablets, 4.5 mg | 12 mos-5 yrs: 4 mg qd; 6-14 yrs: 4.5 mg qd |
Cromolyn Sodium (Intal) | ||
Cromolyn (generic) | Solution for nebulization (10 mg/mL) | 1-2 inhalations tid-qid |
Inhaled β-2 Agonists (Bronchodilators) | ||
Short-acting (SABA) | ||
Albuterol (Proventil, Ventolin) | MDI, 90 mcg/inhalation | 2 puffs q 4-6 hr prn |
Albuterol (generic) | Nebulized solution (0.63, 1.25, or 2.5 mg/3 mL; 2.5 mg/0.5 mL) | 0.63 or 1.25 mg tid-qid prn |
Levalbuterol (Xopenex) | Nebulized solution (0.31, 0.63, or 1.25 mg/3 mL) | 0.31-0.63 mg tid prn (6-11 yrs) |
Long-acting (LABA)* | ||
Salmeterol (Serevent) | DPI, 50 mcg/blister | 50 mcg bid (≥4 yrs) |
Theophylline (generic) | Oral solution, 80 mg/15 mL | <1 yr: max, 0.2 × (age in weeks) + 5 = dose in mg/kg/d |
Tablet (immediate release), 100 mg | >1 yr: 10 mg/kg/d; max, 16 mg/kg/d | |
Capsule (sustained release), 125, 200, 300 mg | ||
Anticholinergics | ||
Ipratropium bromide (Atrovent) | MDI, 17 mcg/puff | 3-14 yr: 1-2 puffs qid (for acute exacerbation); >14 yr: 2 puffs qid |
Ipratropium bromide (generic) | Nebulized solution (500 mcg/2.5 mL) | 125-250 mcg q 6-8 hr mixed with albuterol (5-12 yr) 250-500 mcg q 6-8 hr (>12 yr)† |
DPI, Dry powder inhaler; MDI, metered-dose inhaler; prn, as needed
* Recommended only for use in combination with inhaled corticosteroid for children >4-5 years of age. Data from Bacharier LB et al.: Diagnosis and treatment of asthma in childhood: a PRACTALL consensus report, Allergy 63:5, 2008; modified from Treatment guidelines from the medical letter 6:86, 2008.
† Zorc JJ et al.: Ipratroprium bromide added to asthma treatment in the pediatric emergency department, Pediatrics 103:748, 1999.
Corticosteroids
ICSs have become popular for the treatment of asthma because of their potent antiinflammatory effect on the airways with limited systemic effects (as compared with oral steroids) and are a first-line regimen recommended in treatment guidelines. Regular use of an ICS allows effective control of symptoms and improvement in lung function, reduces airway inflammation, and results in a gradual reduction in airway hyperreactivity (Konig, 1988; Juniper et al., 1991). Although corticosteroids inhibit the in vitro proliferation of airway smooth muscle cells from subjects without asthma, they do not do so in airway smooth muscle cells from patients who have asthma (Roth et al., 2004). Recommended doses of ICS generally have minimal effects on the HPA axis; however, high doses, especially of fluticasone, have resulted in reduction of cortisol levels and symptomatic adrenal insufficiency in children (Barnes and Pedersen, 1993; Todd et al., 2002; Sim et al., 2003). Oral or parenteral corticosteroids are most effective for acute exacerbations of asthma unresponsive to maximal bronchodilator therapy (Chapman et al., 1991; Schuh et al., 2000).
Leukotriene-Receptor Antagonists
Leukotriene-receptor antagonists are a class of drugs developed for the prevention and treatment of bronchial asthma. They are an alternative first-line treatment or may be added if ICSs do not provide adequate control. The formation of leukotrienes through the 5-lipoxygenase pathway depends on lipoxygenation of arachidonic acid, a major constituent of cell membrane phospholipids, detached by phospholipase A2 activity. Leukotrienes are potent bronchial smooth muscle constrictors; on a molecular basis, leukotrienes C4 and D4 (LTC4 and LTD4) are approximately 1000 times more potent than histamine (Undem and Lichtenstein, 2001). Bronchial smooth muscle constriction by leukotrienes is considered a major cause of asthmatic symptoms. Leukotriene-receptor antagonists (e.g., zafirlukast, montelukast) are selective high-affinity LT1-receptor antagonists (Jones et al., 1995). Their use is associated with increased exhaled nitric oxide (NO) (Straub et al., 2005). Montelukast has been reported to be effective as maintenance therapy in children with moderate to severe asthma, with or without concomitant steroid therapy, with minimal side effects (Knorr et al., 2001; Phipatanakul et al., 2003).
β2-Adrenergic Agonists
For maintenance therapy in more severe RAD and for the prevention of exercise-induced bronchoconstriction, long-acting β2-agonists (LABAs) are recommended (Bacharier et al., 2008). Studies suggest a small risk of increased asthma-related deaths in patients older than 12 years of age who take LABAs, especially if they are used regularly without an ICS; thus, the FDA requested that a “black box warning” be added to the labels of salmeterol and salmeterol/fluticasone in 2003 (Nelson et al., 2006). An FDA meeting was later convened to consider safety concerns regarding LABAs in adults and children. Although few children younger than 12 years of age have been studied, the risk of asthma exacerbations and hospitalizations was higher in children 4 to 11 years of age who received a combination drug with LABA and ICS when compared with patients who received ICS alone (Bisgaard and Szefler, 2006; Kramer, 2009). Until further studies are undertaken, it has been recommended that LABA not be given as monotherapy but only in combination with ICS (Drazen and O’Byrne, 2009). This concern does not apply to the use of short-acting β2-agonists (SABAs), which are the drugs of choice for the treatment of intermittent episodes, acute exacerbations, and prevention of exercise-induced asthma in patients with RAD. The recommended doses of commonly used β2-agonists are listed in Table 36-10.
Methylxanthines
Methylxanthines (e.g., theophylline) produce bronchodilation by inhibiting adenosine-induced bronchoconstriction in asthmatic patients—not as was formerly thought, by competitively inhibiting phosphodiesterase, which metabolizes cAMP (Holgate, 1984). Although theophylline was the mainstay of pediatric asthma therapy in the 1970s and 1980s, it is no longer used for therapy in acute exacerbations of RAD (Rooklin, 1989). Theophylline still has a role in decreasing the severity of persistent bronchospasm, especially that which occurs at night. Theophylline has a narrow therapeutic index, with greatest efficacy at serum levels of 5 to 15 mcg/mL. Levels greater than 20 mcg/mL are associated with symptoms of toxicity, such as nausea, gastroesophageal reflux, irritability, learning difficulties in children, and headache (Creer and Gustafson, 1989; Ellis, 1985). Vomiting, tachyarrhythmias, and seizures can occur at higher levels. The great variability of drug metabolism and the necessity for monitoring of blood levels are two reasons the use of theophylline has declined (Drugs for Asthma, 2002).
Cromolyn Sodium and Nedocromil Sodium
Cromolyn sodium does not have a bronchodilator effect; therefore it is exclusively a prophylactic agent and has no bearing on anesthetic practice. It attenuates bronchoconstriction caused by allergen, exercise, and bronchial challenge (Stempel, 2003). Nedocromil sodium has similar chemical and biological properties to cromolyn, which became available in the early 1990s (van Bever and Stevens, 1992). Cromolyn and nedocromil are thought to act on pulmonary mast cells and stabilize cell membranes. They reduce IgE antibody-induced release of inflammatory mediators, including histamine and leukotrienes, from activated mast cells (Douglas, 1985). Maintenance therapy with cromolyn or nedocromil is recommended in children with moderate to severe asthma.
Preanesthetic Consideration
Physical examination should be focused on careful auscultation of the chest for clinical evidence of bronchoconstriction (i.e., expiratory wheezing), use of the accessory muscles of respiration, and a prolonged expiratory phase. During severe episodes of bronchospasm, air movement may become so limited that wheezing may be barely audible. Patients with a history of BPD and asthma are most likely to have lower-airway obstruction and small airway hyperreactivity; wheezing and rhonchi may not be present (see Chapter 17, Neonatology for Anesthesiologists). The preanesthetic level of oxygen saturation should be obtained with a pulse oximeter while the child is breathing room air to determine the baseline oxygen saturation and assess for any preexisting hypoxemia. This information is exceptionally valuable for the postoperative assessment of lung function and gas exchange.
Anesthetic Management
The anesthesiologist must get to know the child with asthma and his or her parents and gain their confidence to minimize the child’s anxiety before anesthesia induction. The child should be well sedated to avoid struggle and hyperventilation, which can provoke exercise-induced asthma. Midazolam, which may be administered transmucosally (i.e., orally, nasally, or rectally) in infants and young children and orally or intravenously (if IV access is present) in older children, works well for sedation. A β2-adrenergic agonist may be given prophylactically using a metered-dose inhaler or nebulizer before induction (Table 36-10). Otherwise, the drug can be given after the induction of anesthesia through the ETT using the metered-dose inhaler and an aerosol chamber inserted in between the ETT adapter and the anesthesia circuit.
The anesthetic approach is similar to that for children with URIs. After applying standard monitors (a minimum of a pulse oximeter and precordial stethoscope if the child is uncooperative), the inhalation induction should be smooth and progress swiftly with sevoflurane and nitrous oxide (see Chapter 11, Monitoring). For infants and young children, heated humidification should be used if available; the dry gas mixture from the anesthesia machine is a perfect environment for provocation of bronchospasm secondary to irritation and reduced tracheal temperature from evaporative heat loss of the tracheal mucosa in a child with asthma (McFadden and Gilbert, 1994).
For IV induction, propofol may be a better agent of choice than thiopental because it suppresses airway reflexes as compared with barbiturates, although thiopental, despite risk of histamine release, is not necessarily contraindicated in patients with asthma (Brown et al., 1992; Gal, 1994). Propofol may also produce bronchodilation in patients with other types of airway disease (Conti et al., 1993). Regardless of the drug chosen, it is important to give sufficiently large IV doses to blunt the reflex response, and sevoflurane should be added before the peak effect of the IV agent is lost.
Whenever possible, endotracheal intubation should be avoided in patients with asthma, because the ETT stimulates large airway irritant receptors and can trigger bronchospasm (Hirshman, 1983). When no contraindications exist, an LMA is a good choice for patients with RAD, as its use avoids the laryngeal and tracheal stimulation of intubation (Groudine et al., 1995). It may also be prudent to avoid anesthetic agents that might release histamine (e.g., atracurium and morphine), although clinical evidence that such drugs actually cause intraoperative bronchospasm is limited.
An anesthetic technique using a volatile anesthetic may be preferable to a balanced technique (i.e., nitrous oxide, opioid, and muscle relaxant) for patients who have asthma because of the salutary bronchodilating properties of volatile agents. Regional anesthesia can be combined with inhalation anesthesia with sevoflurane or isoflurane. Desflurane may cause more airway irritation in patients with RAD and lead to bronchospasm and associated coughing, especially during emergence (von Ungern-Sternberg et al., 2008).
Intraoperative Wheezing
The differential diagnosis of intraoperative wheezing includes “light anesthesia,” kinked ETT, mainstem bronchial intubation, increased airway secretions, foreign body in the airway, pulmonary edema, embolus, and aspiration. In the child with RAD, wheezing can result from exacerbation of airway hyperreactivity and requires immediate attention. The treatment of intraoperative bronchospasm is detailed in Box 36-7. Treatment should begin after chest auscultation to confirm that there are bilateral breath sounds and therefore no mainstem intubation. The first step includes increasing the inhaled concentration of oxygen and deepening the level of anesthesia with volatile anesthetics, or administering IV propofol or ketamine (0.5 to 2 mg/kg), a known bronchodilator (Corssen et al., 1972; Hirshman et al., 1979). Lidocaine (1 mg/kg) may also be given intravenously to reduce airway reactivity at the earliest sign of bronchospasm. Administration of muscle relaxant and suctioning of the ETT may be performed if the patient is intubated. The second step consists of the administration of β2-agonists given by a metered-dose inhaler and adapter through the ETT, followed by the squeezing of the anesthesia bag manually to provide a vital-capacity maneuver to distribute the bronchodilator mist to the tracheobronchial tree. Because only 5% to 10% of the administered dose may reach the end of the ETT and contact the airway, 4 to 8 puffs of the β2-agonist should be administered through the ETT. This maneuver may be repeated two or three times. Parasympatholytic agents (atropine, 0.02 to 0.03 mg/kg) or antihistamines (diphenhydramine, 0.5 mg/kg) are indicated when wheezing is associated with increased vagal tone or histamine release, respectively. The development of hypotension, urticaria, or flushing should lead to the consideration of anaphylaxis. Corticosteroids (e.g., 2 mg/kg of IV hydrocortisone) should be given, and circulation should be supported with appropriate vasoactive agents.
Bronchopulmonary Dysplasia
BPD is a chronic disease of lung parenchyma and small airways with chronic respiratory insufficiency that occurs in prematurely born infants (see Chapter 17, Neonatology for Anesthesiologists). As originally described by Northway’s group (1967), BPD develops after a period of acute and subacute ventilator-induced lung injury and oxygen toxicity, in prematurely born infants with severe respiratory distress syndrome (Hazinski, 1990). Although Northway’s original series involved infants born at a mean gestational age of 34 weeks, all of whom had received excessive concentrations of oxygen during mechanical ventilation with a primitive ventilator by modern standards, over time, BPD has been seen in infants who had prolonged barotrauma (or volutrauma) in the absence of “excessive” oxygen. Early series were characterized by a high incidence of mortality with persistent respiratory symptoms and oxygen requirement beyond 4 weeks of age. Chest radiographs were abnormal and characterized by hyperinflation of the lungs with focal areas of increased density. Northway et al. called this condition BPD to “emphasize the involvement of all the tissues of the lungs in the pathologic process” (Northway et al., 1967; Northway, 2001).
The number of infants with BPD has not decreased over the past two decades despite improved neonatal intensive care, probably because of the survival of more infants who are premature. However, the clinical picture has changed with the advent of antenatal steroids, the use of surfactant therapy, and advances in ventilatory strategies for reducing volutrauma and ventilator-induced lung injury, including noninvasive techniques such as nasal continuous positive airway pressure (Geary et al., 2008, Greenough et al., 2008). Currently, most infants who develop BPD are born at 24 to 28 weeks’ gestation and are rarely born later than 32 weeks’ gestation, whereas the mean gestational age of Northway’s original series was 34 weeks (Hazinski, 1990). Because of changes in neonatal intensive care and affected patient population, many aspects of BPD have changed, including the definition, theories of pathogenesis, pathology, and clinical picture (Jobe and Ikegami, 2000; Jobe and Bancalari, 2001).
Infants with BPD today are likely to have a minimal respiratory distress syndrome that does not progress after surfactant administration. The reason for prolonged ventilation in these premature infants is more commonly apnea or poor respiratory effort, which may be related to immaturity of central respiratory control mechanisms. These infants rarely require the high airway pressures and high oxygen concentration that led to the “old BPD.” This newer clinical picture had been referred to as chronic lung disease or “new BPD,” but it is now simply called BPD. The current definition of BPD is oxygen dependence for at least 28 postnatal days. Evaluation for the presence of BPD and grading of severity is assessed at 36 weeks’ postconceptual age in infants born at fewer than 32 weeks’ gestation and at 56 days after birth in infants born at or after 32 weeks’ gestation (Jobe and Bancalari, 2001). BPD is graded as mild, moderate, or severe, based on oxygen requirement and the need for ventilatory support (Table 36-11). Prevalence ranges from 67% in the smallest weight group to 1% in the largest, with an overall prevalence of 20% of infants born at less than 1500 g (Lemons et al., 2001; Bancalari et al., 2003).
TABLE 36-11 Assessment of the Severity of Bronchopulmonary Dysplasia*
Grade | Fio2 and Ventilatory Support | |
---|---|---|
GA at Birth <32 wks Assessed at 36 Weeks’ PCA | GA at Birth ≥32 wksAssessed at 56 Days Postnatally | |
Mild | 0.21 | 0.21 |
Moderate | 0.22-0.30 | 0.22-0.30 |
Severe | >0.30 and/or continuous positive airway pressure or mechanical ventilation | >0.30 and/or continuous positive airway pressure or mechanical ventilation |
GA, Gestational age; PCA, postconceptual age.
* Applies to patients who have been treated with >21% oxygen for at least 28 days.
Modified from Baraldi E, Filippone M: Chronic lung disease after premature birth, N Engl J Med 357:1946, 2007.
Pathogenesis
In the past, the development of BPD was associated with a condition that caused respiratory failure in the neonatal period (e.g., prematurity with respiratory distress syndrome, meconium aspiration syndrome, or congenital diaphragmatic hernia). Mechanical ventilation with high concentrations of oxygen (i.e., an acute insult to immature lungs) was employed, usually lasting longer than 1 week. Oxygen free radicals, which are not well handled by an immature antioxidant host-defense system in the neonatal lungs, can cause direct cellular injury (Ackerman, 1994). Although much lower concentrations of oxygen are now used than in the past, even room air (21% oxygen) is relatively hyperoxic for a premature infant whose in utero Po2 is less than 30 mm Hg (Hazinski, 1990). Excessive hydration and patent ductus arteriosus with increased pulmonary fluid have been recognized as additional important factors contributing to the development of BPD (Gerhardt and Bancalari, 1980; van Marter et al., 1992). The current theory of the mechanism of injury in BPD also emphasizes the role of infection and inflammation (Gonzalez et al., 1996; Sadeghi et al., 1998). Recurrent bacterial or viral infections in these infants may cause persistent alveolitis, which worsens alveolar and airway damage (Rojas et al., 1995; Hannaford et al., 1999). Multiple markers of inflammation (e.g., lipid mediators, proteases, oxygen free radicals, and cytokines) are elevated (Bose et al., 2008; Ryan et al., 2008). Nutritional deficiencies may also play a role (Sosenko et al., 2000; Geary et al., 2008).
Immature, inflamed lungs with decreased compliance are most susceptible to high-volume (i.e., volutrauma) and low-volume (i.e., shear stress trauma) trauma with marked distortion and distention of terminal bronchioles at high positive pressures (Hazinski, 1990). In earlier pathologic examination of lungs of infants dying with BPD, peribronchiolar fibrosis and smooth muscle thickening were seen. They were also found in animal models exposed to prolonged positive pressure ventilation and hyperdistention (Coalson, 1999). The pathology now seen in extremely premature infants reflects the immature state of their pulmonary parenchyma, with enlarged and simplified alveolar structure and reduced numbers of capillaries that are dysmorphic in appearance. Therefore, the new BPD is now regarded primarily as a disorder of lung development with superimposition of mechanical factors. Fibroproliferation may still occur but is more variable. Changes in larger blood vessels are less prominent with less indication of pulmonary hypertension than seen in old BPD. Airway smooth muscle hyperplasia may still occur, but it is also more variable (Coalson, 2006). After this damage has occurred to immature lungs, infants may require prolonged mechanical ventilation and high oxygen concentration for weeks or months, despite having not required high oxygen concentrations in the first few weeks of life. Although less common than with old BPD, progressive respiratory failure with associated pulmonary hypertension, with or without cor pulmonale, may follow.
Even after the perinatal period, RAD persists in infants with BPD. Mallory et al. (1991) studied lung function longitudinally in infants with moderate to severe BPD during the first 4 years of life with the forced deflation technique and found that airway hyperresponsiveness or hyperreactivity continued to be present in all children studied. They postulated that airway hyperreactivity is an important etiologic factor for the pathogenesis of lower airway obstruction in BPD. Attributing the cause of RAD to BPD is confounded by the recent evidence that antenatal steroid therapy to hasten lung maturation is a risk factor for the development of RAD between the ages of 3 and 6, as well as by the fact that the rates of RAD-like symptoms occur more often in prematurely born children than in those born at term (Doyle, 2006; Pole et al., 2009). Most long-term studies of lung function are reports of infants who had the old form of BPD. Little information is available regarding long-term outcomes of infants with new BPD.
Preanesthetic Considerations
Most infants with moderate to severe BPD remain dependent on oxygen, with or without CPAP, or dependent on a ventilator beyond 4 weeks of age. They have persistent lower airway obstruction and airway hyperreactivity (Mallory et al., 1991). Tachypnea and dyspnea may be intermittently or chronically present. Growth failure because of chronic hypoxia despite oxygen therapy may occur, as well as cor pulmonale associated with pulmonary hypertension (Hazinski, 1990). Wheezing may or may not be present on auscultation, because the site of airway hyperreactivity is primarily in the periphery of the lungs as a result of increased thickness of the airway wall (Tiddens et al., 2008). In addition to lower airway obstruction primarily involving small airways, infants who have been intubated for prolonged periods sometimes develop large airway disease such as subglottic stenosis (that may or may not be recognized), tracheomalacia, and bronchomalacia (Miller et al., 1987; McCubbin et al., 1989). A later study also found a greater degree of upper airway obstruction in children with history of BPD, as compared with age-matched children with asthma (Sadeghi et al., 1998).
Infants with mild forms of BPD improve with age and may become asymptomatic, but airway hyperreactivity may persist. Parents of such an infant may not be aware of the history of BPD even when their child received prolonged mechanical ventilation as a neonate. It is appropriate, therefore, to assume that a child has or had BPD and has RAD if he or she was born prematurely and was mechanically ventilated for more than 1 week during the neonatal period. Inguinal hernia is often present in infants with BPD, probably owing to prematurity and continually increased abdominal pressure that results from airway obstruction and increased inspiratory effort. Prematurely born infants may require postoperative admission for monitoring, because they have an increased risk of postoperative apnea as discussed in Chapters 3, Respiratory Physiology in Infants and Children; 17, Neonatology for Anesthesiologists; and 18, Anesthesia for General Surgery in the Neonate.
As with asthmatic patients, careful history taking is of utmost importance before anesthetizing an infant with BPD or a history of BPD. These patients may have failure to thrive (a sign of chronic hypoxia). With lower respiratory tract infection, worsening of symptoms or even respiratory failure may occur. The patient may be taking SABAs or other treatments for asthma. Other medications may include diuretics. A family history of allergy and asthma is significant, because premature birth may be linked to smooth muscle hyperresponsiveness and asthma (Bertland et al., 1985). Relatively common surgical conditions in infants and children with BPD or a history of BPD include inguinal hernia, direct laryngoscopy and bronchoscopy for subglottic stenosis, and surgical procedures of the larynx for the complications of prolonged intubation or tracheostomy (e.g., excision of granuloma or laryngotracheoplasty).
Anesthetic Management
Anesthetic management of infants and children with BPD or a history of BPD is similar to that for those with asthma. Before anesthetizing a child in this population, it is imperative to obtain a baseline oxygen saturation measurement with a pulse oximeter (SpO2), although a normal oxygen saturation level does not necessarily guarantee the absence of lung dysfunction. Many infants and young children with a history of BPD maintain remarkably good SpO2 values, presumably because of hypoxic pulmonary vasoconstriction (HPV). The infant with BPD with near-normal SpO2 in room air may develop marked desaturation after induction with sevoflurane, presumably because of a loss of HPV under general anesthesia, although HPV in healthy human volunteers may be insignificant (Benumof, 1994). In these patients, oxygen saturation may be maintained better with IV induction and maintenance techniques using opioids and propofol; however, no evidence supports this management protocol. Preoperative prophylactic treatment with a β2-adrenergic agonist by a metered-dose inhaler or handheld nebulizer may be beneficial for patients with potential airway hyperreactivity to prevent perioperative bronchoconstriction. In intubating a child with a history of mechanical ventilation, it is prudent to start with an ETT one size (0.5 mm inner diameter) smaller than the appropriate size for the age in anticipation of subglottic narrowing, which may be the result of prolonged intubation. If rapid sequence intubation is required because of fasting violation or intestinal obstruction, desaturation may be rapid when apnea occurs, and gentle ventilation by mask with maintenance of cricoid pressure may be necessary to maintain saturation if intubation is not rapidly accomplished.
Cystic Fibrosis
Cystic fibrosis (CF), an autosomal-recessive disorder, is the most common life-limiting inherited disorder among whites (Rosenstein and Cutting, 1998). In the United States, the gene frequency (heterozygotes) in whites is approximately 1 in 28; it is uncommon among Hispanics (1 in 46) and African Americans (1 in 65) and lowest among Asians and Native Americans (1 in 90) (Hamosh et al., 1998). The disease incidence among whites is approximately 1 in 2500 live births. Owing to early diagnosis and aggressive treatment over the past 40 years, in 2007 the median survival of a CF patient had increased to 37 years (Cystic Fibrosis Foundation, 2007).
In 1985, Tsui et al. localized the gene responsible for the manifestation of CF to 250 kilobases on the long arm of chromosome 7. The deletion of three base pairs, removing a phenylalanine residue at position 508 (d508) from a 1480-amino acid protein called CF transmembrane conductance regulator (CFTR), a cAMP-dependent chloride ion channel, accounts for approximately 70% of CF chromosome abnormalities (Moskowitz et al., 2008). Of the other 1000 documented mutations, 20 account for most of the remaining 30% of cases, with the prevalence of mutations varying among ethnic groups (Tsui and Zielenski, 2007). Although the type of CFTR mutation does correlate with pancreatic function, poor correlation exists between the type of mutation and the severity of lung disease. Evidence suggests that other genetic polymorphisms influence the severity of lung disease (Drumm et al., 2005).
Pathogenesis
CF is characterized by exocrine gland dysfunction that results in chronic pulmonary disease, pancreatic dysfunction, and abnormalities in electrolyte reabsorption in the sweat duct, with increases in sweat sodium and chloride concentrations and electrolyte imbalance. The fact that CF patients have sweat chloride levels in excess of 60 mEq/L (normal is less than 40 mEq/L), as measured by pilocarpine iontophoresis, is the basis of the sweat chloride test, which is still the gold standard for making the diagnosis of CF. Increasingly, the diagnosis of CF is being made earlier because of newborn screening techniques that are designed to detect elevated serum levels of immunoreactive trypsinogen and the most common CFTR mutations (Comeau et al., 2007). Such screening accounted for 21.6% of the diagnoses of CF in the United States in 2006 (Cystic Fibrosis Foundation, 2007). Even if these screenings are found to be positive, two sweat chloride test results of greater than 60 mEq/L are necessary to make the diagnosis of CF. Significant clinical manifestations of CF, other than pulmonary disease, include those listed in Table 36-12.
Organ System | Incidence (%) |
ENT | |
Pansinusitis | 90-100 |
Nasal polyps | 20 |
Gastrointestinal | |
Pancreatic | |
Enzyme deficiency | 85-90 |
Diabetes secondary to pancreatic failure | 15 |
Intestinal | |
Meconium ileus (newborn) | 7-20 |
Distal intestinal obstruction syndrome (includes intussusception) | 10-30 |
Rectal prolapse | 20 |
Gastroesophageal reflux disease | 50 |
Liver | |
Hepatic failure | 5-20 |
Coagulopathy caused by vitamin K deficiency | 100 if untreated |
Pulmonary | |
Pneumothorax caused by bleb rupture | 5-8 |
Pulmonary disease is the most common cause of morbidity and death. Enhanced absorption of sodium across the airway epithelium and failure to secrete chloride and fluid toward the airway lumen is thought to lead to dehydration and thickening of airway mucus and abnormal mucociliary clearance with subsequent bronchial inflammation and infection. Patients are initially colonized with Haemophilus influenzae and then by Staphylococcus aureus, and eventually by the mucoid variant of Pseudomonas aeruginosa. Colonization with Aspergillus and atypical mycobacteria may occur. The chronic infection in the periphery of the tracheobronchial tree results in bronchiolitis, which may lead to airway hyperresponsiveness, bronchiectasis, lobar or segmental atelectasis, and pneumothorax. Advanced disease is associated with destruction of airway architecture, fibrosis of lung parenchyma, and development of abscesses. Hemoptysis and eventually, cor pulmonale and respiratory failure ensue (Eckles and Anderson, 2003).
Small airway obstruction, hyperinflation, and ventilation- perfusion imbalance are the most common and important pulmonary changes in children with moderate-to-severe CF. The early signs of lung dysfunction include a reduction in maximum expiratory flow rates at low lung volumes (e.g., FEF25-75, FEF50, and FEF75) and an increase in the ratio of residual volume to total lung capacity (RV/TLC) (see Chapter 3, Respiratory Physiology in Infants and Children). Airway hyperreactivity is often present, probably in response to airway inflammation. Some patients have a good response to bronchodilators, but others have inconsistent or even paradoxical responses, sometimes worsening airway function because of the relaxation of airway smooth muscles and resultant increases in airway collapsibility (Brand, 2000).
Treatment
Patients with CF take multiple medications. In 2007, a committee organized by the Cystic Fibrosis Foundation published guidelines for long-term medication used for optimal pulmonary symptom control in CF patients older than 6 years (Flume et al., 2007). Patients with a prominent bronchospastic component are on long acting β2-agonist therapy (Hordvik et al., 2002). They often take inhaled or oral antibiotics for prophylaxis or treatment of pulmonary infection. Patients infected with P. aeruginosa often take aerosolized tobramycin, which when administered on a bimonthly basis has been shown to preserve pulmonary function and reduce hospitalization (Ramsey et al., 1999). Patients with infectious exacerbations are treated with IV antibiotics in a hospital or at home. Chest physiotherapy several times per day is a mainstay of CF treatment. Inhaled mucolytics (N-acetylcysteine) have long been used to decrease the viscosity of pulmonary secretions, but little is found in the literature documenting their efficacy (Duijvestijn and Brand, 1999). Inhaled hypertonic saline, which is thought to improve fluidity of pulmonary secretions, has been shown to decrease the frequency of pulmonary exacerbations (Flume et al., 2007). Dornase alfa (i.e., human recombinant DNase), which dissolves deoxyribonucleic acid (DNA) released from neutrophils, has improved pulmonary function and reduced the frequency of infection (Jones et al., 2003; Flume et al., 2007). High-dose ibuprofen therapy has been found to slow the decline of forced expiratory volume during the first second of measurement (FEV1) in children and adolescents if started when the FEV1 is greater than 60% of that predicted (Lands and Stanojevic, 2007). This effect is related to reduction in inflammation (Nichols et al., 2008). Doses between 20 and 30 mg/kg every 6 hours are administered to achieve plasma concentrations of 50 to 100 mcg/mL (Arranz et al., 2003). Ibuprofen treatment has been associated with a significant increase in gastrointestinal (GI) bleeding compared with patients who are not taking ibuprofen, although the absolute number of patients affected is small (Konstan et al., 2007). Patients with pancreatic impairment require pancreatic enzyme replacement.
Preanesthetic Considerations
Common surgical indications in infants and children with CF are listed in Table 36-13. Although some patients with CF require surgery in the neonatal period for meconium ileus, no special anesthetic considerations are necessary. Management of children with CF is a challenge to the anesthesiologist. These patients are often frail and malnourished. Decreased plasma albumin levels may affect anesthetic potency. Intravascular volume may be diminished because of chronic diarrhea, poor oral intake, and diuretic therapy. Electrolyte imbalance may result from excessive chloride and sodium losses. Pulmonary function ranges from near normal without airway obstruction to severe obstruction, air trapping, hypoxemia, and hypercapnia. Copious thick secretions and resultant ventilation-perfusion imbalance may prolong mask induction with volatile anesthetics. Secretions may irritate the larynx and precipitate laryngospasm. Nasal polyps may block the nasal airway completely during mask induction. Pathophysiologic considerations in patients with CF that may affect anesthetic management are listed in Table 36-14.
TABLE 36-13 Surgical Indications for Patients with Cystic Fibrosis
Conditions | Typical Age Range |
Meconium ileus or equivalent | 1 d-3 yr |
Nasal polyp/sinusitis | 10-18 yr |
Other procedures | 10-18 yr |
Bronchoscopy | |
Feeding gastrostomy; central venous access (port or PICC) | |
Lobectomy or thoracoplasty/thoracoscopy | |
Organ transplantation (double lungs; heart-lungs) |
PICC, Peripherally inserted central catheter.
TABLE 36-14 Pathophysiology of Cystic Fibrosis: Effect on Anesthetic Management
Pathophysiology | Possible Outcome |
Pulmonary Dysfunction | |
Airway obstruction V/Q imbalance |
Prolonged mask induction |
Copious secretions Airway hyperreactivity |
Laryngospasm, bronchospasm |
Nasal polyp | Upper airway obstruction |
Gastrointestinal and Hepatobiliary Disorders | |
Decreased serum albumin levels | Increased drug potency |
Coagulopathies | Increased bleeding |
Diabetes or glucose intolerance | Hyperglycemia, acidosis |
Abnormal sweat gland function | Electrolyte imbalance |
Cor pulmonale | Hemodynamic instability; dysrhythmia |
The preoperative evaluation should include the assessment of pulmonary function by history, physical examination, and pulmonary-function testing. Pulmonary-function testing should include assessments of maximum expiratory flow-volume curves, lung volume measurements, and response to bronchodilators. An increase in TLC and the RV/TLC ratio with decreased vital capacity indicates the presence of hyperinflation and air trapping. Lower airway obstruction with small airway involvement is demonstrated when FEF25-75, FEF50, and especially FEF75 are markedly decreased from predicted values. A recent preoperative chest radiograph is needed in patients with moderate to severe pulmonary disease. Preoperative oxygen saturation should be obtained by means of pulse oximetry in room air for postoperative comparison. Recent tracheal culture results should be reviewed as a guide to choice of perioperative antibiotic therapy. In patients with significant lower airway obstruction and air trapping, preoperative arterial blood gas measurement is recommended to assess the degree of hypoxemia more accurately and to evaluate the presence of hypercapnia and acid-base status. In those with long-standing hypoxemia, pulmonary hypertension and cor pulmonale should be suspected. These patients should have preoperative electrocardiography and echocardiography to evaluate myocardial function and reserve. Blood sugar, liver-function tests, and coagulation studies may be indicated. Eight percent of CF patients between the ages of 11 and 17 develop CF-related diabetes mellitus as a result of fibrosis and fatty infiltration of the pancreas (Cystic Fibrosis Foundation, 2007). Some, but not all, may require insulin, because some insulin continues to be produced. Malabsorption and liver disease may be associated with abnormal coagulation. Additional vitamin K supplementation may be necessary to correct coagulopathy. The patient’s pulmonary physician should be consulted to ensure that the patient’s condition is optimized as much as is possible before surgery. Ibuprofen should be stopped 2 days before surgery to allow its inhibitory effects on platelet aggregation to dissipate.
The child with CF and his or her family are exceedingly knowledgeable regarding the pathogenesis and treatment of the disease. A lack of knowledge of CF in general, and of the patient’s past history and present conditions in particular, at the time of the preoperative visit could quickly undermine the confidence of the family in the anesthesiologist. More importantly, the patient with CF is often petrified by the thought of death under anesthesia, in part because of a long-held belief in the pulmonary medicine community that general anesthesia leads to prolonged deterioration of pulmonary function (Price, 1986). It is therefore prudent for the anesthesiologist to gain the patient’s and the parents’ confidence and administer preoperative sedation such as oral midazolam (Della Rocca, 2002). Opioid premedication should be avoided in severe cases because of possible respiratory depression and hypoxemia.
Anesthetic Management
In patients with significant pulmonary involvement, IV access should be established before the induction of anesthesia because of prolonged mask induction and possible nasal obstruction from nasal polyps. An anticholinergic may be given during induction. Concern about excessive drying of secretions is unfounded, because atropine decreases secretions without changes in viscosity and has not been a significant problem in clinical practice (Lamberty and Rubin, 1985). IV propofol may be preferred to thiopental because it is less irritating to the upper airways and actually causes bronchodilation. Ketamine, despite its bronchodilating properties, is relatively contraindicated because it tends to increase secretions and may cause laryngospasm. Fifty percent of children with CF have gastroesophageal reflux disease and may require rapid-sequence intubation, although chronic treatment with H2-blockers and or a proton-pump inhibitor along with an adequate fasting interval mitigates the risk of aspiration. Inhalation induction is usually satisfactory in young children with mild lung disease. Anesthetic gases should be heated and humidified to prevent irritation of the upper airways and laryngospasm and to avoid drying and inspissation of secretions. When trapped gas volume is suspected or proven by pulmonary function test, nitrous oxide should be avoided to prevent expansion of the emphysematous area and the potential danger of bleb rupture.
Endotracheal intubation with muscle relaxation is mandatory in patients with severe respiratory involvement, although the anesthesiologist should be exceedingly careful not to overdistend already air-trapped lungs. When a nondepolarizing muscle relaxant is chosen, the effect of aminoglycoside antibiotics to prolong the duration of action of such drugs must be kept in mind, and monitoring of train-of-four should be used to guide relaxant administration. It is also mandatory to carefully monitor end-tidal carbon dioxide to prevent hyperventilation and to maintain preoperative arterial partial pressure of carbon dioxide (Pco2) levels, which may be elevated. Sudden hypocapnia in a chronically hypercapnic patient can disrupt the patient’s ventilatory control mechanisms, increasing the chance that the patient might require postoperative ventilation. After intubation, tracheobronchial suction should be performed and repeated at intervals throughout surgery and before extubation to improve pulmonary gas exchange. Although the use of an LMA might be an option for short cases, disadvantages include the inability to suction secretions, obstruction of the LMA by thick secretions, risk of laryngospasm, and risk of aspiration in patients with gastroesophageal reflux disease. For bronchoscopy for the purpose of removal of secretions for culture and bronchial lavage, the LMA has been safely used in patients with CF (Nussbaum and Zagnoev, 2001). Intraoperatively, glucose should be monitored in patients with glucose intolerance. Care should be taken to conserve heat in these patients with reduced body fat.
Regional anesthesia should be considered whenever applicable. Although regional anesthetic techniques without general anesthesia might be useful in some situations, these techniques should be carefully considered in children with severe pulmonary disease. Depression of abdominal and intercostal muscle function by thoracic levels of spinal or epidural anesthesia may not be tolerated. Pediatricians and pulmonologists often request regional anesthesia instead of general anesthesia because of fear that severely affected patients with CF will not tolerate general anesthesia or may become dependent on a ventilator after endotracheal intubation. However, most of these sick patients with CF, dyspneic or orthopneic with hypercapnia and oxygen dependence, may not tolerate even a short surgical intervention, such as insertion of a central venous catheter or MediPort, with local anesthesia and sedation. Instead, general endotracheal anesthesia with an inhaled agent, supplemented by caudal, lumbar, or thoracic epidural anesthesia for abdominal or thoracic procedures, is much better tolerated, safer, and provides good operative conditions, rapid emergence, and a pain-free postoperative state (Dalens et al., 1986). If epidural anesthesia is to be used, coagulopathy should be ruled out, and the appropriate concentration of local anesthetic drugs should be chosen to minimize motor block. Continuous caudal or epidural anesthesia with local anesthetic with or without carefully chosen doses of an opioid provides prolonged postoperative pain relief and facilitates coughing and deep breathing after upper abdominal or thoracic procedures. If regional anesthesia is not appropriate, judicious use of inhalation agents and short-acting opioids and wound infiltration with local anesthetic by the surgeon should facilitate early extubation, which is desirable in most cases. After surgeries without a high risk of postoperative bleeding, the use of NSAIDs may be effective in reducing the amount of opioid needed for analgesia.
Cystic Fibrosis and Hemoptysis
Hemoptysis may occur in older children with more severe lung disease. These children may come to the hospital for angiography and bronchial artery embolization. Preanesthetic considerations as described above should be evaluated, and the patient’s pulmonary status should be optimized to the greatest extent. Pulmonary hemorrhage has been reported after induction of general anesthesia, intubation, and institution of positive-pressure ventilation (McDougall and Sherrington, 1999). It is possible that airway and parenchymal distention associated with positive pressure ventilation results in the breach of relatively thin-walled vessels. These authors have recommended that sedation and local anesthesia may be safer in this situation (McDougall and Sherrington, 1999; Barben et al., 2002)
Cystic Fibrosis and Lung Transplantation
For patients with end-stage pulmonary disease, lung transplantation may be the final surgical option. Fifty percent of patients with CF and FEV1 of less than 30%, partial pressure of oxygen (Pao2) less than 55 mm Hg, or partial pressure of carbon dioxide (Paco2) greater than 50 mm Hg will survive for 2 years and may be candidates for lung transplantation (i.e., double-lung or heart-lung procedure) (Belkin et al., 2006). Approximately 175 patients with CF per year undergo bilateral lung transplantation in the United States, of whom the minority are children (Cystic Fibrosis Foundation, 2007). The 3-year survival rate is 60%, which is similar to that seen in patients who do not have CF (Sweet et al., 1997). Some (27%) lung-transplant patients develop bronchiolitis obliterans, which is responsible for 40% of deaths that occur more than 1 year after transplantation (Boucek et al., 2004). Among the survivors of lung transplantation, no recurrence of CF in the transplanted lungs has been reported, as measured by the transepithelial potential differences (Alton et al., 1991). The management of end-stage CF patients for lung transplantation is described in Chapter 3, Respiratory Physiology, and Chapter 28, Anesthesia for Organ Transplantation.
Cardiovascular Disorders
Cardiovascular disorders are commonly encountered in the pediatric population. The baseline incidence of congenital heart disease in the population is approximately 0.8 in 100 births, on which is superimposed an incidence of acquired heart disease. However, more and more congenital cardiac defects have been appreciated to have either a genetic or an environmental basis, either alone or in combination, such that incidence and risks in offspring may be higher in selected populations (Jenkins et al., 2007). Both congenital and acquired diseases have the ability to affect myocardial function, valve function, and conduction tissue, all of which can also be affected by anesthetics. In addition, anesthetic effects on vascular tone can have a positive or negative impact on myocardial function and shunting of blood through intracardiac defects.
Patients with cardiac disease should be identified preoperatively. Although children with congenital heart disease who undergo noncardiac surgery should generally do well with appropriate anesthetic and perioperative care, preliminary information suggests that in the aggregate, congenital cardiac disease of even a moderate degree can increase mortality and adverse events during and after noncardiac surgery (Baum et al., 2000). Even hemodynamically insignificant lesions may necessitate perioperative endocarditis prophylaxis (Table 36-15). However, not all surgical procedures or all children with cardiac disease require endocarditis prophylaxis. Recommendations for endocarditis prophylaxis were significantly changed in 2007, with a general decrement in the use of prophylaxis, because previous recommendations were recognized to be lacking in validity or efficacy or were overly complicated (Wilson et al., 2007). Current recommendations are outlined in Boxes 36-8 and 36-9.
TABLE 36-15 Endocarditis Prophylaxis Regimens for Dental Procedures
Reason | Agent | Regimen |
Standard general prophylaxis | Amoxicillin | 50 mg/kg orally (adults: 2 g) |
Unable to take orally | Ampicillin | 50 mg/kg IM or IV* (adults: 2 g) |
OR | ||
Cefazolin or ceftriaxone | 50 mg/kg IM or IV* (adults: 1 g) | |
Allergic to penicillin | Clindamycin | 20 mg/kg orally (adults: 600 mg) |
OR | ||
Cephalexin | 50 mg/kg orally (adults: 2 g) | |
OR | ||
Azithromycin or clarithromycin | 15 mg/kg orally (adults: 500 mg) | |
Allergic to penicillin and unable to take orally | Clindamycin | 20 mg/kg IV* (adults: 600 mg) |
OR | ||
Cefazolin or ceftriaxone | 50 mg/kg IM or IV* (adults: 1 g) |
IM, Intramuscularly; IV, intravenously.
* It is appreciated that many children do not have IV access prior to surgery. IV antibiotics should be given as soon as possible and before surgical incision. Children’s dose should not exceed adult dose.
Modified from Wilson W et al: Prevention of infective endocarditis: guidelines from the American Heart Association, Circulation 116:1736, 2007.
Box 36-8 Changes in Most Recent Endocarditis Prophylaxis Recommendations
Data from Wilson W, et al: Prevention of infective endocarditis: guidelines from the American Heart Association, Circulation 116:1736, 2007.
Box 36-9 Cardiac Conditions that Necessitate Antibiotic Endocarditis Prophylaxis
Data from Wilson W, et al: Prevention of infective endocarditis: guidelines from the American Heart Association, Circulation 116:1736, 2007.
Anesthetic Management
Although the specifics of the anesthetic management of individual cardiac problems are discussed in Chapters 20, Anesthesia for Congenital Heart Surgery, and 21, Anesthesia for Children with Congenital Heart Disease Undergoing Non-Cardiac Surgery, the following comments are generally applicable.
Intraoperative Period
Although much discussion is appropriately devoted to the specifics of cardiac pathophysiology, most children with congenital heart disease who develop problems during anesthesia do so for primarily noncardiac reasons, particularly airway compromise (Strafford and Henderson, 1991). Infants who are cyanotic, in particular, begin with decreased oxygen saturation and can rapidly desaturate with transient interruption in ventilation, whether as a result of apnea or airway obstruction. Children with severe congestive failure or cyanosis have a decreased margin of safety and tolerate failures of respiratory or hemodynamic management poorly.
Much time is spent discussing the effects of left-to-right and right-to-left shunts on the onset time of IV and volatile anesthetics. Although differences exist, with currently available anesthetic gases of relatively low solubility, these differences are almost always so small as to be clinically irrelevant. In the absence of a complication such as loss of the airway or the development of a hypercyanotic “tet” spell in children with tetralogy of Fallot or variants, oxygen saturation in children with cyanotic disease almost invariably increases with induction of anesthesia (Laishley et al., 1986; Greeley et al., 1986). Several reasons are possible, one of the most likely being a decrease in oxygen consumption that causes an increase in mixed venous oxygen saturation and subsequently higher arterial oxygen saturation when some of this blood is shunted right to left.
Nitrous oxide is a mild myocardial depressant. In adult patients, it can increase PVR, particularly in patients in whom PVR is already elevated. However, in children, no significant increase in PVR has been observed with 50% nitrous oxide, regardless of the preexisting PVR (Hickey et al., 1986).
End-tidal Pco2 correlates with arterial Pco2 in acyanotic patients. However, in children and adults with cyanotic congenital heart disease, end-tidal Pco2 tends to underestimate arterial Pco2 (Burrows, 1989).
The Child with a Murmur and Possible Heart Disease
Cardiac murmurs are exceedingly common in healthy children, with an overall lifetime incidence of at least 50%. Most of these are the somewhat inappropriately named functional murmurs (also called innocent). The incidence of functional murmurs is highest at 3 to 4 years of age. Functional murmurs represent the sound of blood flowing through a structurally normal heart (Fig. 36-7). No anesthetic concern is associated with these murmurs, and the family should be reassured. Several functional murmurs are commonly recognized, almost all of which are short and soft but become louder when the patient lies supine. Most functional murmurs become louder with increased cardiac output, as would occur with anemia, fever, exercise, or anxiety.
Occasionally, a murmur is appreciated for the first time when children arrive for a preanesthetic evaluation. The exact method of evaluation remains somewhat controversial (Yu et al., 2002). Isolated chest radiographs and electrocardiograms are generally a poor investment (Yu et al., 2002). In addition, electrocardiograms that are interpreted by computer or an adult cardiologist may need to be reinterpreted using age-appropriate normal values. Location of the murmur can also aid in the diagnosis (see Chapter 9, Preoperative Preparation, Fig. 9-3).
Noncardiac Manifestations of Congenital Heart Disease
Longstanding cyanotic and acyanotic congenital disease can have effects on the function of a variety of other organ systems. Some of these effects may not become clinically apparent until years after surgical correction of the underlying cardiac defect (Table 36-16).
TABLE 36-16 Potential Noncardiac Manifestations of Congenital Heart Disease
System | Causes/Implications for Anesthetic Management |
Pulmonary/Thoracic | |
Decreased lung compliance | Occurs in lesions with increased pulmonary blood flow (i.e., left-to-right shunting) or pulmonary venous obstruction; can require higher airway pressure for ventilation; can impinge on small airways, resulting in air trapping, wheezing |
Scoliosis | More common with cyanotic lesions; can manifest in adolescence, years after corrective cardiac surgery |
Hemoptysis | Can occur in end-stage Eisenmenger syndrome (pulmonary hypertension caused by prolonged excessive pulmonary blood flow) |
Phrenic nerve injury | From prior surgery; more common after surgery at the apices of the thorax (e.g., patent ductus arteriosus ligation, coarctation, pulmonary artery banding, or Blalock-Taussig shunt) |
Recurrent laryngeal nerve injury | From prior surgery or from an enlarged hypertensive pulmonary artery; see phrenic nerve injury (above) |
Blunted ventilatory response to hypoxemia | In cyanotic patients; normalizes after surgical repair; normal ventilatory response to hypercarbia |
Hematologic | |
Symptomatic hyperviscosity | Due to chronic hypoxemia; occurs with hematocrit above approximately 65% (or lower if iron deficient); may cause neurologic symptoms |
Bleeding diathesis | Abnormalities of a variety of factors have been described in patients who have cyanosis with no consistent pattern; elevated central venous pressure can cause increased operative bleeding, as can increased tissue vascularity with cyanotic disease (collateral blood vessel formation); increased risk of bleeding with prior thoracic surgery during subsequent thoracic procedures |
Gallstones | Calcium bilirubinate stones from increased heme turnover in cyanotic disease; may not become symptomatic until years after corrective cardiac surgery |
Neurologic | |
Paradoxical emboli to central nervous system | Can manifest even with a predominantly left-to-right shunt lesion |
Brain abscess in patients with right-to-left shunts | Can manifest with seizure focus years later |
Cerebral thrombosis | In children with erythrocytosis but not in adults |
Compression of nerve by vascular structure | Recurrent laryngeal nerve by enlarged, hypertensive pulmonary artery |
Nerve injury during prior surgery | Recurrent laryngeal, phrenic, or sympathetic chain; see phrenic nerve injury (above) for high-risk surgeries |
Vascular | |
Femoral vein complications | Thrombosis or ligation from prior cardiac catheterization |
Anatomic discontinuity of inferior vena cava and right atrium | Congenital, associated with polysplenia syndrome |
Anatomic discontinuity of right internal jugular and innominate vein with right atrium | As a result of Glenn shunt |
Reduced lower extremity blood pressure | Coarctation of the aorta; left arm involvement is variable |
Discontinuity of subclavian artery | With classic Blalock-Taussig anastomosis; stenosis of the subclavian artery after the modified Blalock-Taussig anastomosis; currently, classic Blalock-Taussig shunt is rarely performed |
Artifactually elevated right arm blood pressure | Supravalvar aortic stenosis (the Coanda effect) |
Kawasaki Disease
Originally named mucocutaneous lymph node syndrome after its major manifestations, Kawasaki disease is the most common cause of acquired heart disease in children in the United States. The etiology for this disease, with characteristics of an infectious disease without an identifiable agent and a vasculitis that is not easily treated with steroids, has yet to be determined. In the United States, the peak incidence occurs between 13 and 24 months of age. Diagnosis and therapy have been reviewed in detail, and a general overview has been presented by Burns (2007) (Newburger et al., 2004). No diagnostic test has been developed. The acute illness is associated with fever; intense conjunctival injection; red, cracked lips and oral mucosa; erythema of the palms and soles; and less commonly, cervical lymphadenitis of the neck followed weeks later by desquamation of the skin of the fingers and toes. Arthritis and arthralgia can also develop. Other uncommon systemic manifestations include transient high-frequency hearing loss, gastrointestinal complaints, hepatomegaly, and hydrops of the gallbladder. Erythema and induration at the site of a previous bacillus Calmette-Guérin (BCG) vaccination can also occur. The most concerning feature of the disease is that it causes an infantile periarteritis nodosa-like vasculitis of medium and large arteries in 10% to 15% of children. Of particular concern is involvement of coronary arteries, with the risk of subsequent thrombosis or, less commonly, rupture (Fig. 36-8). The risk of coronary artery aneurysms is higher in infants. In addition, the acute phase of the illness can be associated with myocarditis—usually mild but sometimes associated with heart failure. Myocarditis is usually transient, lasting several weeks. Laboratory findings during the acute phase reveal an intense acute-phase response that includes neutrophilia, elevated sedimentation rate and C-reactive protein, and thrombocytosis to over 800,000/mcL3.
Treatment in the acute phase includes IVIG and aspirin. Aspirin is begun at a high dosage (80 to 100 mg/kg per day) and continued either until the child is afebrile or until day 14 of illness, after which the high antiinflammatory dose is decreased to antiplatelet levels (3 to 5 mg/kg per day). The lower dose is continued until the child shows no evidence of aneurysm formation (6 to 8 weeks after onset) or is continued indefinitely if coronary aneurysms develop. Although the mechanism is undetermined, IVIG (2 g/kg) is an effective acute therapy that is successful in approximately 80% of cases. If IVIG therapy fails, children are at high risk for the development of coronary artery complications, which may be treatable with steroids, although a recent study suggests this may not be effective (Newburger et al., 2007).
If coronary artery aneurysms do develop, approximately one half to two thirds regress within 2 years, and approximately one fifth develop coronary stenoses. Smaller aneurysms (less than 8 mm in diameter) and fusiform aneurysms are more likely to regress than larger or saccular aneurysms. Larger aneurysms can develop thromboses or stenoses with subsequent ischemia, or they can rupture. Rupture is rare and usually occurs within the first month or two of disease. Ischemia can develop years after the acute illness. Even if aneurysms regress, intimal proliferation can result in endothelial dysfunction (Furuyama et al., 2003). Warfarin has been used at some centers to treat children with giant aneurysms. Angioplasty has been attempted at several centers with mixed results, and surgical bypass grafting has been performed on occasion for high-grade obstruction of the left main coronary artery or at least two of the major coronary arteries. Because of the young age of the patients, grafting is completed with arterial rather than venous grafts (Kitamura et al., 1994).
Takayasu Arteritis
Takayasu arteritis, a vasculitis of the aorta and its major branches that is sometimes known by its catchy synonym “pulseless disease,” is an uncommon disease in children. However, 75% of patients will have begun to develop symptoms during adolescence. An important cause of hypertension in teenagers in Asia, where it is more common, it occurs 8 times more often in females. Narrowing of major arteries results in limb claudication or end-organ disease. Blood pressure in limbs can be artifactually low or unobtainable. Early vessel inflammation is followed by fibrosis. Headaches are a common symptom. The subclavian artery is involved in 90% of cases, and two thirds of all cases involve the aorta, both supradiaphragmatic and infradiaphragmatic. The carotid artery, almost always the left, is involved in half of cases. Stenoses are more common than occlusion, and occlusion is more common than aneurysm formation. Mural inflammation and thickening occur, and coronary and pulmonary arteries are uncommonly affected. Aortic root dilation can result in aortic valve insufficiency. Initial treatment is with corticosteroids. Long-term therapy is often required, and cytotoxic drugs are sometimes added. Once fibrosis has occurred, treatment is by stenting or surgery (Rigby et al., 2002; Kalangos et al., 2006). Unfortunately, there is often recurrence with tapering of steroid therapy or with time after surgical treatment (Maksimowicz-McKinnon et al., 2007).